This Spotlight examines emerging trends in next generation wireless networks that are shaping the connectivity ecosystem. These trends raise questions about how to make the next generation of wireless technologies a commercial and ubiquitous reality. The Spotlight dives into research initiatives, market trends and developments towards integration of terrestrial wireless connectivity solutions like 5G and 6G with non-terrestrial technologies, such as satellites and other aerial platforms. In so doing, it identifies challenges for spectrum policy, regulatory collaboration, interoperability, environmental sustainability, safety and digital security, and digital divides. These issues, both technical and regulatory, must be addressed to realise future visions.
OECD Digital Economy Outlook 2024 (Volume 1)
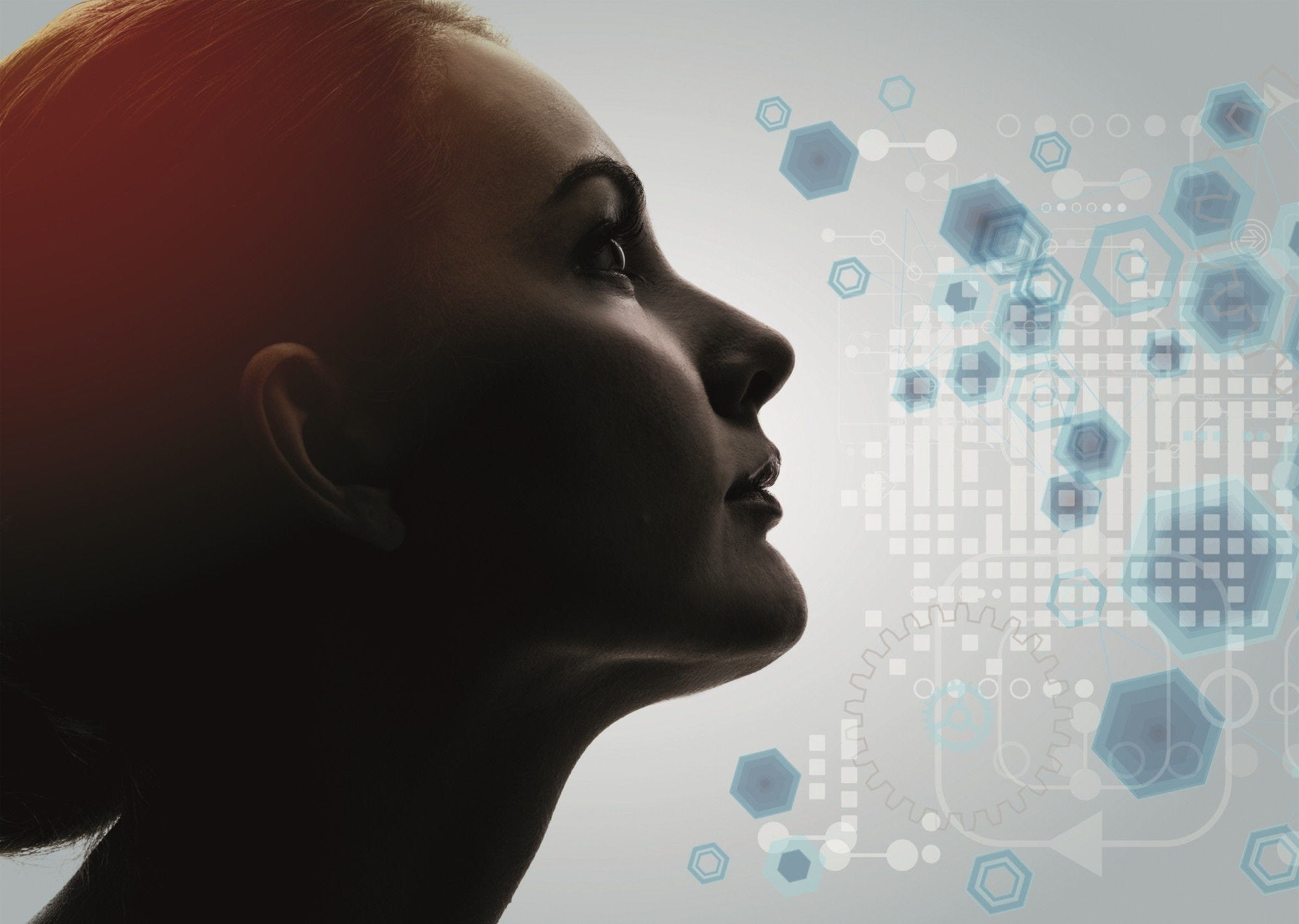
Spotlight. Next generation wireless networks and the connectivity ecosystem
Copy link to Spotlight. Next generation wireless networks and the connectivity ecosystemAbstract
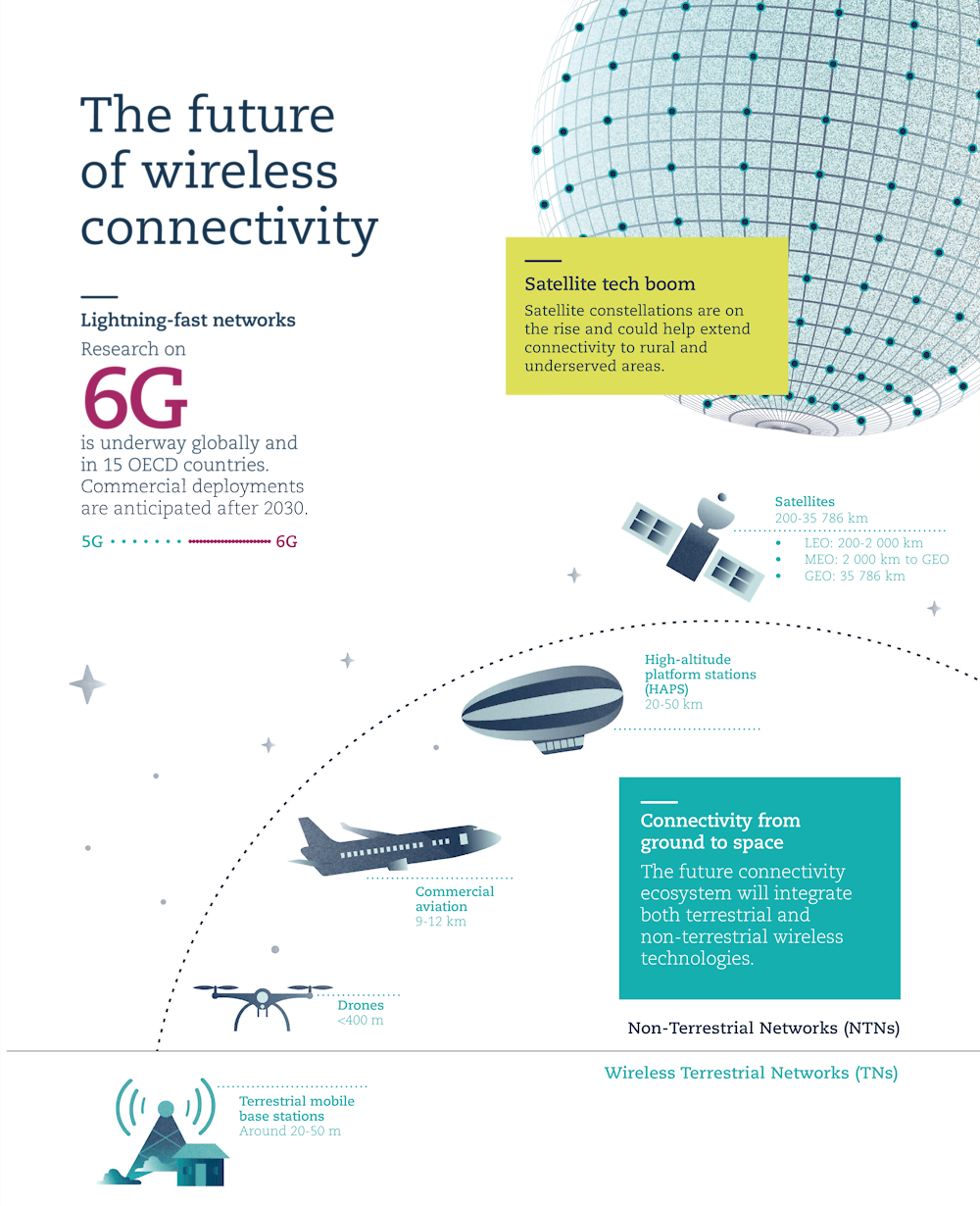
Source: OECD elaboration based on multiple sources, including Ofcom, the European Space Agency and GSMA.
Next generation communication networks are driven by technological developments in the communications industry, as well as current and anticipated demand on networks. The amount of data sent over communication networks has increased steadily for both consumer and business use cases. This growing volume has been spurred by developments in artificial intelligence (AI) systems and the Internet of Things (IoT), and other emerging technologies. Enhanced machine and human communications and interactions, as well as human-augmenting technologies for sensorial communication, including haptics (Internet-of-senses), are likely to influence future demand on networks. In addition, fully automated vehicles, for example, will shape future demand on wireless networks.
The combination of virtual reality (VR), augmented reality (AR) and extended reality (XR) environments and applications is shaping human communications. Moreover, it influences the way humans communicate with machines and their environments as the digital, human and physical worlds merge (Hexa-X, 2022[1]). These trends in immersive technologies, a topic explored in Chapter 4 on virtual reality, will require broadband networks to adapt. Moreover, haptic technologies – which are relatively nascent – are advancing together with AR applications. They are starting to be used in areas such as surgery simulations, immersive communication and health care, and for vision- and hearing-impaired people.
All these advances have important implications for future networks: they generate large amounts of data, leading to higher bandwidth and data processing requirements. Most of these applications also rely on improved broadband performance, i.e. higher speeds and lower network response times (latency). On top of these network performance requirements, increased network resilience will be needed, not only for critical applications, but for all use cases. Most importantly, broadband networks have evolved from connecting people to connecting “everything”, a step towards unlimited and embedded connectivity “everywhere” in the future. As such, a key question is how to prepare networks, and in particular wireless networks, to respond to these technological developments.
Extending connectivity is both necessary and desirable as digital transformation depends on it. Continuing to grow an understanding of the connectivity ecosystem is therefore essential to ensure evidence-based policy making in this area. That said, the OECD sets policy rather than technological standards, and technological futures are highly uncertain in a dynamic area such as communication infrastructures and services. With this in mind, the Spotlight sheds light on possible developments in next generation wireless networks in the next five to ten years, highlighting the potential to extend high-quality connectivity “everywhere”. As such, it complements OECD work on trends driving the networks of the future. These include a higher level of integration of cloud into networks, edge computing, virtualisation and network automation (OECD, 2022[2]). While the Spotlight concentrates on wireless networks, extending fibre deeper into networks is critical to increase network performance and reliability of all broadband access technologies.
The Spotlight focuses on two important developments likely to shape wireless communications in the next decade. First, “beyond 5G” terrestrial technologies are expected to push performance boundaries in wireless communications. Second, non-terrestrial networks (NTNs),1 including low Earth orbit (LEO) satellite constellations and different types of aerial platforms, will also shape the future of wireless communications.
The integration of wireless terrestrial networks (TNs)2 and NTNs raises new challenges, both from technical and policy perspectives. Emerging research explores how to integrate these network types (i.e. hybrid connectivity topologies). In the future, hybrid satellite and terrestrial wireless networks could, for example, provide connectivity solutions for customers using IoT, maritime and aviation applications (OECD, 2022[2]).
Terrestrial connectivity: Beyond 5G technologies
Copy link to Terrestrial connectivity: Beyond 5G technologies“Beyond 5G” – sometimes called “6G” – technologies have not yet been defined. Several countries, research institutes and the communication sector (including operators and equipment manufacturers) are conducting research and development on beyond 5G technologies with an expected commercial launch at the end of this decade (OECD, 2022[2]). Research is taking place in such countries as the People’s Republic of China (hereafter “China”), Finland, Germany, Japan, Korea, Singapore, the United Kingdom and the United States, as well as in the European Union. In the OECD area, 15 countries have government initiatives and/or industry alliances working towards development of 6G technology.3 The ITU-R Working Party 5D, for example, started work on the “Vision of IMT beyond 2030” in 2021 (ITU, 2021[3]). It has set a timeline to complete the International Mobile Telecommunications (IMT) 2030 specifications by the end of 2029 (ITU-R, 2022[4]).4
Figure 1.S.1 illustrates a potential timeline of 6G development. While 6G research is ongoing, the vision for the next evolution of mobile networks is being conceived in the context of 5G network deployments. As of January 2024, 5G commercial deployments were available in 37 of 38 OECD countries in some form. Most 5G commercial networks are based on non-standalone (NSA)-5G (i.e. relying on a 4G core network and using NSA-5G standards in the radio interface known as New Radio).5 However, standalone (SA)-5G deployments are on the rise.
Many of the promised transformational aspects of 5G are likely to commence with SA-5G networks that use both the 3rd Generation Partnership Project (3GPP)6 core network architecture standards for 5G (i.e. 5G Core), as well as the 5G radio interface (i.e. New Radio) (OECD, 2022[2]). As such, the 5G standard will continue to evolve. The 3GPP has already announced “5G advanced” for upcoming releases of the standard (3GPP Releases 18 to 20 for “5G Advanced”) that will lead to 6G.
The vision of IMT beyond 2030, expected from the ITU-R Working Party 5D by the end of 2023, sets the main specifications for beyond 5G technologies. This will allow standard-setting bodies to work on mapping these identified key values and specifications into technical standards. The standardisation process of 6G will likely commence after 2025, with the first commercial deployments occurring perhaps after 2030. With regards to spectrum allocation, preparatory work to identify possible additional spectrum for 6G (“IMT 2030 Framework”) is ongoing (ITU-R, 2023[5]). This was discussed during the ITU World Radiocommunication Conference 2023 (WRC-23) with a view to potentially incorporating it as an agenda Item for WRC-27. During the WRC-23 in November 2023, discussions centred on what the appropriate frequencies might be, and whether the IMT badge would be required (Figure 1.S.1). The WRC-23 concluded with an agreement to analyse the 7 to 8.5 GHz band for 6G services for the next WRC conference taking place in 2027 (LightReading, 2023[6]).
Figure 1.S.1. The road to 6G: Potential timeline
Copy link to Figure 1.S.1. The road to 6G: Potential timeline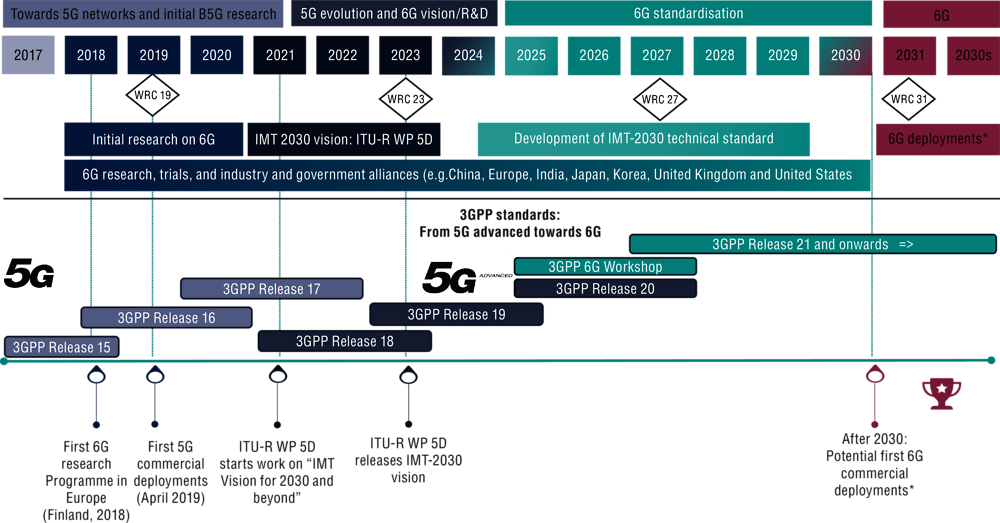
Notes: B5G = Beyond 5G.
*Descriptive and tentative timeline for illustrative purposes. Development and commercial launch of 6G depends on multiple factors, including when different countries start to deploy.
Source: OECD elaboration based on desk research from several sources: presentations from different stakeholders at the June 2022 ITU-R Workshop “IMT for 2030 and Beyond” (ITU-R, 2022[7]) [e.g. Wireless World Research Forum, Samsung Research, HAPS Alliance, European 6G Flagship Hexa X, NextG Alliance (United States); Beyond 5G Promotion Consortium (Japan), one6G Association (Greece); “6G: Building Metaverse ready Mobile Networks” and “Use cases” (several universities), Finish Transport and Communications Agency; Telecommunications Standards Development Society in India]; Ahokangas, Matinmikko-Blue and Yrjölä (2023[8]), “Envisioning a Future-Proof Global 6G from Business, Regulation, and Technology Perspectives”; Qualcomm (2022[9]), “Vision, market drivers, and research directions on the path to 6G”, www.qualcomm.com/content/dam/qcomm-martech/dm-assets/documents/Qualcomm-Whitepaper-Vision-market-drivers-and-research-directions-on-the-path-to-6G.pdf; Ericsson (2022[10]), “6G: Connecting a cyber-physical world”, www.ericsson.com/4927de/assets/local/reports-papers/white-papers/6g--connecting-a-cyber-physical-world.pdf; and 3GPP (2023[11]), “Releases”, www.3gpp.org/specifications-technologies/releases.
While 6G discussions are nascent, they will combine evolutionary and revolutionary aspects. On the one hand, they will build on 5G wireless networks. On the other, they will rely on wireless applications combining communication and sensing, and increased use of higher frequency bands, such as terahertz spectrum (OECD, 2022[2]). Researchers assume the integration of sensing information and time synchronisation may enable new applications for interactive and multiparty connectivity, within and between virtual and physical worlds (ITU-R, 2022[7]).
Like previous generations of mobile networks, several interrelated factors will likely shape the future of mobile wireless networks and their impact on the evolving connectivity ecosystem. This evolution will likely include integration of various wireless connectivity solutions beyond mobile connectivity (e.g. Wireless Local Area Networks [WLANs], such as Wi-Fi, and NTNs) together with terrestrial connectivity solutions, such as submarine cables, and an increased focus on indoor connectivity. As networks become more sophisticated, the move towards a “network of networks” (also referred to as “multi- layered networks”) will raise new challenges. Factors shaping future mobile networks include i) the overarching policy objectives or key values being considered for the next generation of mobile networks; ii) spectrum management and other regulatory challenges; iii) the evolution of the technological standard itself based on research, trials and emerging use cases; and iv) implementation of use cases through evolving business models (Figure 1.S.2).
Figure 1.S.2. Interrelated factors that will likely shape 6G during the next decade
Copy link to Figure 1.S.2. Interrelated factors that will likely shape 6G during the next decade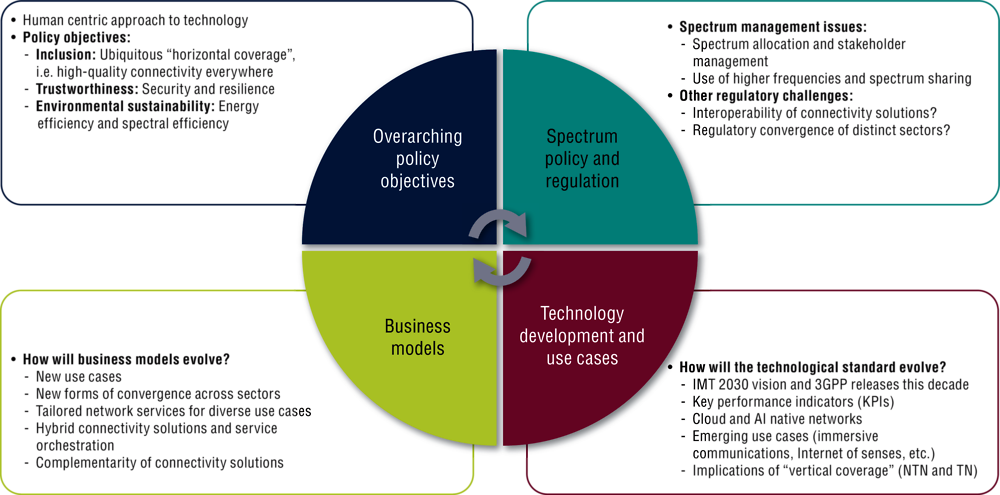
Notes: NTN = Non-terrestrial network; TN = Terrestrial network. Definitions: Resilience of communication networks refers to the ability of a network to cope with shocks, while maintaining an acceptable level of service. It can be strengthened by ensuring network diversity and redundancy when planning and rolling out infrastructure. A digital security incident is an intentional or unintentional event that can disrupt the availability, integrity and confidentiality of data, information systems and communication networks.
Sources: OECD elaboration based on desk research on the different 6G visions being discussed (as of February 2023). Sources include: presentations from different stakeholders at the ITU-R Workshop “IMT for 2030 and Beyond” (ITU-R, 2022[7]); Ahokangas, Matinmikko-Blue and Yrjölä (2023[8]), “Envisioning a Future-Proof Global 6G from Business, Regulation and Technology Perspectives”; and Policy Tracker (23 January 2023[12]), “Do 5G and 6G change spectrum policy?”, www.policytracker.com/blog/our-new-podcast-spectrum-policy-101.
Overarching policy objectives. The work towards 6G focuses on the values of sustainability and digital inclusion, as well as increased network security. Environmental sustainability, for example, aims at a higher energy and spectral efficiency than previous mobile network generations. For its part, digital inclusion focuses on bridging connectivity divides through “horizontal” coverage everywhere for everyone. Another fundamental aspect is increased digital security and network resilience, both reflecting the value “trustworthiness”. As such, several institutes and researchers involved in recent 6G initiatives underscore that new applications and technologies should follow a human-centric approach (6GSymposium, 2021[13]; OECD, 2022[2]). The values of sustainability, inclusion and trustworthiness, all part of a human-centric approach, have been prominent in the 6G visions of researchers, industry alliances and countries. Nevertheless, how these values will manifest in the standardisation phase through key performance indicators remains to be seen.
In this regard, Digital and Tech Ministers of G7 countries noted their common “Vision for future networks in the Beyond 5G/6G era” in 2023. The statement highlights the importance of high-capacity and ultra-low latency networks to enable critical applications, the role of “multi-layered networks” that marry TN and NTN solutions to ensure universal “digital connectivity”, the need to minimise the environmental impacts of networks, and the importance of spectrum efficiency, modularity, and interoperability to reach policy objectives (G7, 2023[14]).
Spectrum management and regulatory issues. A range of factors will influence the management and regulation of spectrum. These include research into 6G, the continued relevance of low- and mid-band spectrum, and the higher profile of policies to improve wireless networks, allocate spectrum, manage stakeholders and ensure interoperability. Some use cases being researched for 6G include higher spectrum bands, such as millimetre wave (above 24 GHz) and Terahertz spectrum (above 100 GHz).7 For example, in December 2022, the European Telecommunications Standards Institute launched an industry specification group on Terahertz communications as a potential candidate for 6G. It focuses on data-intensive applications such as VR and AR, as well as applications merging sensing and communication functionalities, such as holographic telepresence (ETSI, 2022[15]). Low- and mid-band spectrum will likely continue to play a key role (GSMA, 6 February 2023[16]).
Overall, spectrum policies that fundamentally affect the improvement of wireless networks will become more relevant. For example, spectrum-sharing models will only increase in importance. The same is true for equipment standards not directly related to the operation of a communication network but affecting spectrum efficiency (e.g. receiver performance).8 Flexibility in spectrum management, including flexible access spectrum sharing, will be key given the considerable uncertainty in demand, both in terms of new applications and services, as well as the diverse options to deliver next generation wireless communication services (Ofcom, 2023[17]). As new players from outside communication sectors become more prominent in the wireless broadband connectivity ecosystem, spectrum allocation and stakeholder management will become even more critical. Apart from spectrum policy, other regulatory challenges include how to ensure the interoperability of diverse connectivity solutions, and how to deal with the convergence of distinct sectors that may be outside the remit of communication regulators.
Technology development and use cases. Some researchers believe that 6G may foster new use cases providing ubiquitous communication, as well as high accuracy and low-latency joint communication, sensing and positioning systems (Bourdoux et al., 2020[18]; Ofcom, 2021[19]).9 Two factors may enable ubiquitous coverage and enhanced quality of experience. First, research is exploring use of smart surfaces made of artificial materials (“metamaterials”) deployed along streets and buildings to go beyond the traditional physical wireless limits. Second, research is also moving towards hybrid terrestrial-aerial topologies (Ofcom, 2021[19]). Examples of potential applications for 6G technologies include advanced multimedia services such as immersive XR, holograms, digital twins, three-dimensional (3D) calls, haptic communication (i.e. the transmission of touch and motion), fully automated mobility solutions and nano-technology-inspired IoT sensors (Alleven, 2021[20]).
Researchers also point to the need for 6G to be cloud- and AI-native from the start. Such an approach could optimise network management, energy consumption and spectral efficiency, as well as provide enhanced digital security. The use of AI combined with “idle” or “sleep mode”, already available for 5G (OECD, 2022[2]), are also being considered for future wireless networks to improve energy efficiency. In the advent of quantum computing, “security by design” will be key for 6G, including features robust to quantum attacks (Fitzek et al., 2022[21]). In addition, given that countries are likely to deploy 6G networks at different stages, fully virtualised and software-defined networks may support cost-effective and backward compatible solutions to achieve inclusion objectives (Wireless World Research Forum, 2022[22]). Applications are also emerging in the automotive sector (fully automotive vehicles), health care (AI-tailored wireless hospitals) and industry (VR, AI, edge computing and robotics) (6G Flagship, 2023[23]). The use of increasingly higher frequency bands to achieve more throughput coupled increased coverage requirements may impact energy consumption of networks. In this sense, 6G research is focusing on reconciling the trade-off between communication and sensing requirements of 6G applications and energy efficiency (Fitzek et al., 2022[21]). Moreover, ongoing research on 6G has also focused on expanding “vertical coverage” with the integration of cellular TNs with NTNs. This could include satellites in LEO, medium Earth orbit (MEO), geostationary orbit (GEO), air-to-ground (A2G) networks and other aerial platforms possibly resembling high-altitude platform stations (HAPS).
Business models. Multiple issues affect the emergence of business models for 5G and 6G, including the iterative rollout of 5G and its widespread impact across economies, and the kinds of partnerships needed to fully exploit the potential of 6G. Given that standalone 5G is yet to become a reality in most countries, some stakeholders are considering first how 5G will affect digital transformation prior to 6G networks becoming widely accessible. Namely, SA-5G use cases may shed light on future applications and business models for 6G where connectivity may become “network-as-a-service” for tailored use cases. With SA-5G deployments, the promise of the revolutionary aspects of 5G would spread across all economic sectors. Thus, it may help to consider some challenges associated with this deployment when identifying the type of partnerships required to reap the societal benefits of 6G. New forms of collaboration will likely develop across different sectors with the advent of hybrid connectivity solutions and multi-layered networks. Questions of whether different connectivity solutions are complements or substitutes will likely shape business models. Moreover, models to monetise projected use cases, which is a contemporaneous concern for 5G, will likely also be a main discussion point for business models in the next generation of wireless networks.
This section has focused on “beyond 5G” mobile technologies, but other wireless connectivity solutions alongside cellular (mobile) and non-terrestrial wireless networks, are important. These can help meet performance requirements of a variety of use cases stemming from the digital transformation. WLANs, such as Wi-Fi, are a particularly important use case. They benefit from unlicensed spectrum to support connectivity, primarily indoors, for residential home fixed networks, as well as local area networks for enterprises. Wi-Fi networks are also instrumental to offload mobile traffic (OECD, 2022[24], 2022[2]). Building upon previous Wi-Fi generations, the Wi-Fi 610 technological standard aims to improve performance, especially for large outdoor Wi-Fi deployments (e.g. outdoor hotspots). It also seeks to increase throughput in dense deployments and reduce device power consumption (Oughton et al., 2021[25]). Early discussions of Wi-Fi 711 have focused on further increasing performance to support use cases with more stringent service requirements for throughput and latency, e.g. virtual and AR applications (Oughton et al., 2021[25]). The Wi-Fi 7 standard is expected to be published in May 2024 (IEEE, 2022[26]; OECD, 2022[24]).
The development of next generation wireless technologies, such as 6G, provokes an array of questions. Will the world be hyper-connected in 2030? If so, what are the implications? Are there new risks and challenges for networks? What are the key concerns with regards to security, resilience and data capacity? How can policy makers and regulators ensure appropriate levels of spectrum access for the variety of use cases within the context of a constrained resource?
Moreover, many questions surround the need to bridge connectivity divides. The digital divide especially affects vulnerable segments of the population, such as rural and low-income households. By the end of 2022, 34% of the world’s population remained “offline” (ITU, 2023[27]). As such, how can policy makers promote investment and effective competition to expand affordable and high-quality broadband connectivity to all, regardless of where people live? In addition, how can countries build an ecosystem together where trustworthy AI and IoT applications that transcend national borders can flourish? Common approaches to regulation and policy across countries are needed, as well as interoperable standards.
Non-terrestrial connectivity: Developments in satellites and other non-terrestrial wireless technologies
Copy link to Non-terrestrial connectivity: Developments in satellites and other non-terrestrial wireless technologiesSatellites are already providing broadband connectivity to end-users, especially in under- or unserved areas by TNs (e.g. rural and remote areas) and support TNs by offering backhaul services. In addition, other aerial technologies are under development to bridge connectivity divides, and first efforts are taking place to integrate TNs and NTNs.
High-throughput geostationary satellites and non-geostationary orbit satellite constellations
Copy link to High-throughput geostationary satellites and non-geostationary orbit satellite constellationsAdvances in satellite technology, coupled with improvements in launch capabilities and the reduced cost and size of terminal devices, have lowered barriers to entry and expanded the array of satellite-based connectivity solutions. Different constellations are emerging. Some aim to provide end-user communication services (e.g. Starlink or Kuiper), especially in rural and remote areas. Others (Oneweb, Telesat, O3B, Rivada, Kuiper) look to extend the reach of TNs. Together, they complement the connectivity ecosystem. Moreover, an increasing number of satellites provide IoT solutions in remote areas or aim for the logistics sector, including for aircraft and maritime coverage (e.g. Swarm Technologies, Hiber, Kinéis and Globalstar). All these applications strive at achieving connectivity “everywhere”.
In the past, satellites in GEO, synchronised with the Earth’s rotation, largely provided satellite connectivity. While GEO satellites have a large coverage area, they are typically more expensive to build. They also suffer from higher latency as radio signals must travel back and forth to the geostationary orbit in 36 000 km altitude. Newer approaches propose satellites in LEO or MEO, also known as non-geostationary orbit (NGSO) satellites. These are cheaper to build and launch, depending on their size and complexity (OECD, 2017[28]). However, their lower orbits require many more satellites to provide uninterrupted services due to smaller coverage areas globally. As a result, this drives overall costs to generally exceed those of a geostationary satellite fleet.
Several companies plan or have already launched LEO constellations to offer satellite connectivity, including Starlink, OneWeb, Boeing, Telesat and Amazon’s Project Kuiper. Starlink and OneWeb launched their first satellites in 2019. While Starlink and OneWeb have completed their first-generation constellations and are already looking to “Gen2”, Project Kuiper plans to launch its first commercial services in mid-2025. Kuiper successfully launched its first two prototype satellites in October 2023 (Amazon, 2023[29]), and will begin to offer beta services to customers in 2024. As of January 2024, Starlink had 5 374 satellites in orbit and OneWeb 634 satellites in orbit (McDowell, 2024[30]). In December 2022, SpaceX’s Starlink launched 54 second generation satellites to new orbits, adding capacity to its network using the 27-30 GHz bands. SpaceX noted those will allow them to “add more customers and provide faster service, particularly in areas that are oversubscribed” (Howell, 2022[31]).
The new generation of satellites for both GEO and NGSO constellations, known as high-throughput satellites (HTS), offer a host of emerging possibilities. HTS satellites typically use the 10.7-12.7 GHz, 13.75-14.5 GHz, 17.8-18.6 GHz, 18.8- 20.2 GHz, 27.5-30 GHz, 37.5-42 GHz, 47.2-50.2 GHz and 50.4-52.4 GHz bands, with wide beams, spot beams, steerable beams and frequency-reuse technology. This could enable satellites to increase performance and capacity per satellite and focus additional capacity in areas where it is most needed.12 Consequently, smaller remote terminals can be used. Increased power and bandwidth provide faster speeds, lowering equipment cost in the case of geostationary satellites (OECD, 2022[24]). In addition, inter-satellite links are advancing to allow for quicker transmission between satellites. This is particularly useful for constellations as data/content can be routed to the right part of the world (IETF, 2022[32]). For example, inter-satellite links can be used to transfer data more rapidly from Earth observation satellites via communication satellites to the ground. In this way, communications and sensing networks merge in space, as well as on the ground.13
New advances point to using connectivity from satellites for mobile terminals through messaging services facilitated by including satellites in the 3GPP standard Release 15 and onwards.14 Some mobile communication operators are striking partnerships with satellite providers to expand coverage of their networks. For example, T-Mobile in the United States partnered with Starlink (SpaceX) to provide text service to its clients in all US areas, including national parks and other remote regions (T-Mobile, 2022[33]). There is also increasing momentum from the device ecosystem. Several smartphone equipment manufacturers (e.g. Apple or Samsung), chipset providers (MediaTek, Qualcomm) and mobile operators have announced developments to link mobile phones to satellites for emergency communications (Apple, 2023[34]; Browne, 2023[35]; McGregor, 2023[36]; Qualcomm, 2023[37]).
In the United States, the Federal Communications Commission (FCC) created a “space bureau” to support the burgeoning satellite industry. This decision responded to increased applications for satellites that featured new commercial models, players and technologies. The FCC has also adopted rules to shorten the deorbiting period for LEO satellites from 25 to 5 years. In December 2022, it issued a Notice of Proposed Rulemaking to streamline its review processes for satellite applications (FCC, 2022[38]). Furthermore, in March 2023, the FCC proposed a new framework (Notice of Proposed Rulemaking) to facilitate innovative collaborations between satellite operators and mobile providers. It will allow space-based services to connect directly to smartphone users in remote, unserved and underserved areas. The FCC proposed to allow authorised NGSO operators to apply to access terrestrial spectrum under certain conditions (FCC, 2023[39]). Companies like AST Spacemobile and Lynk have been testing their satellite-to-phone services in the United States through experimental licences (Clark, 2023[40]).
OECD countries, including Canada, Colombia, France, Germany and the United Kingdom, have already authorised NGSO constellations to leverage satellites’ potential to help meet domestic connectivity goals, given how satellites can reach remote areas that are under- or unserved by TNs (OECD, 2022[24]). For example, in Colombia in February 2022, the Ministry of Information and Communication Technologies issued a new regulatory regime for satellite services to encourage development of satellite connectivity in the country, especially for hard-to-reach areas (MinTIC, 2022[41]).
The European Commission recently announced plans for an “EU space-based secure connectivity system” leveraging satellite connectivity (European Commission, 2022[42]). In November 2022, the EU Parliament and EU Council announced a provisional agreement for a satellite constellation, IRIS, which will include GEO, MEO and LEO satellites (Evroux, 2023[43]). This will support EU priorities for connectivity – for government, residential and enterprise users – to support the economy, environment, security and defence (European Commission, 2022[44]).
Developments in satellites raise both technical and regulatory challenges, especially for spectrum policy.15 Regulating satellites’ use of spectrum to manage radio interference takes place on two axes. The International Telecommunication Union (ITU) co-ordinates the international level, while regulatory authorities manage the national level (OECD, 2022[24]). The ITU maintains the Master International Frequency Register to record the use of spectrum in space (ITU, 2022[45]). Meanwhile, national communication regulators, including spectrum managers, oversee filings for proposed satellite systems. They submit requested frequencies to the ITU but authorise Earth stations (OECD, 2022[24]). Regulators also consider dangers from space debris when studying the feasibility of proposed satellites (OECD, 2022[24]).16
High-altitude platform stations
Copy link to High-altitude platform stationsHigh-altitude platform stations (HAPS) are flying base stations or network nodes that operate in the stratosphere 20 km above the ground (ITU-R, 2020[46]).17 They show promise to bridge connectivity divides in rural and remote areas (OECD, 2021[47]). Their cell radius can be up to 100 km, compared to 8 km in rural areas for IMT 2020,18 creating a cell area equivalent to the city of Paris (1 000 km2) (ITU-R, 2022[48]).19 HAPS have recently become more viable due to technological advances in energy efficiency, among other factors (ITU, 2022[49]).20 Namely, developments in HAPS technologies may enable high-altitude IMT base stations (HIBSs) – or “HAPS as IMT base stations” as labelled by the ITU-R. This brings them a step closer to connecting the unconnected (RSPG, 2021[50]).
In addition to improving coverage and capacity on the ground, HAPS – like satellites – can help provide connectivity to lower-level aircrafts and drones. This can enable connectivity corridors outside of TN coverage (e.g. over the sea). When used with TNs, such corridors can improve reliability and resilience.
Given that HAPS are flying objects, they are subject to both aviation and spectrum regulations. They can be lighter than air, like balloons, or heavier-than-air, like motorised gliders. As the wind speed is less intense in the stratosphere than in lower altitudes, HAPS can maintain their position in the higher airspace while consuming less energy. As another advantage, they have a large coverage area for mobile connectivity (Araripe d’Oliveira, Cristovão Lourenço de Melo and Campos Devezas, 2016[51]).
During the past decade, several attempts have been made to commercialise HAPS solutions to bridge connectivity divides. Google’s Loon project, for example, was withdrawn in 2021 (Reynolds, 2018[52]; Singh, 2021[53]). SoftBank, through its subsidiary HAPS Mobile, has worked in developing various parts of the HAPS ecosystem (HAPS Mobile, 2023[54]). As one possible explanation for limited use of aerial platforms to date, integration of HAPS/HIBS into mobile networks can create potential interference. It also requires that frequency bands allocated for mobile communications do not restrict use of aeronautical mobile services21 (ITU-R, 2022[48]).
The economic viability of aerial platforms also depends on spectrum availability for these services (OECD, 2022[24]). Policy makers expected to study possible new identifications for using HIBSs as part of IMT networks during WRC-23 (ITU, 2022[49]). HAPS/HIBSs may be deployed more widely as they could provide cost-effective connectivity for consumers in rural areas with limited or no access to communication services.
Hybrid topologies for connectivity: Towards the integration of terrestrial and non-terrestrial network technologies
Copy link to Hybrid topologies for connectivity: Towards the integration of terrestrial and non-terrestrial network technologiesIntegrating different terrestrial and non-terrestrial wireless technologies is a next step in expanding the connectivity ecosystem. Some broadband providers have begun exploring how satellite connectivity and other aerial communication technologies might complement TNs to provide seamless communication with extended coverage. They might do this, for example, through backhaul solutions (Fletcher, 2021[55]) or through hybrid satellite and ground networks for IoT applications (Erwin, 2021[56]). Deutsche Telekom claims to have achieved the world’s first seamless 5G connection through different network layers to the stratosphere, space (Intelsat satellite network) and back to the mobile network (HT Group, 2023[57]).22
The communication industry also aims to integrate TNs and NTNs within the scope of 6G projects (Figure 1.S.3).23 This would support global, ubiquitous and continuous connectivity both on the ground and for aerial users. To that end, it would integrate mobile terrestrial connectivity solutions with MEO, GEO, air-to-ground (A2G) networks, HAPS and other aerial platforms. Integrated networks in 3D with a multi-layered architecture would combine the advantages of TNs and NTNs (aerial platforms as well as satellite networks). In this way, they would provide improved reliable, secure and robust connectivity for aerial and ground users through flexible and adaptive network architectures with multiple technologies (Ozger et al., 2023[58]). However, this integration is not without challenges. These technologies differ greatly, do not provide the same performance features and are not all operated by the same company.
The multi-layered architecture requires an interplay between the aviation, the terrestrial mobile communication sector and space industries. This calls for collaboration between different sectors that over the years have been competing for spectrum. The aviation community, for example, is facing several challenges as its legacy communication systems have a low rate of technology development. It may also have difficulty operating in parts of the spectrum allocated for aeronautical services. At the same time, the aviation industry is advancing in its digitalisation, requiring higher efficiency in the use of airspace with connected aircrafts and the emergence of a wide variety of flying objects. Unmanned vehicles and electric vertical take-off and landing aircrafts such as “flying taxis” could contribute to a more social and healthier environment in cities (Box 1.S.1) (EASA, 2022[59]). In November 2022, for example, France opened a hub for testing electric air taxis (Bloomberg, 2022[60]).
Figure 1.S.3. Towards vertical coverage: Convergence of terrestrial and non-terrestrial networks
Copy link to Figure 1.S.3. Towards vertical coverage: Convergence of terrestrial and non-terrestrial networks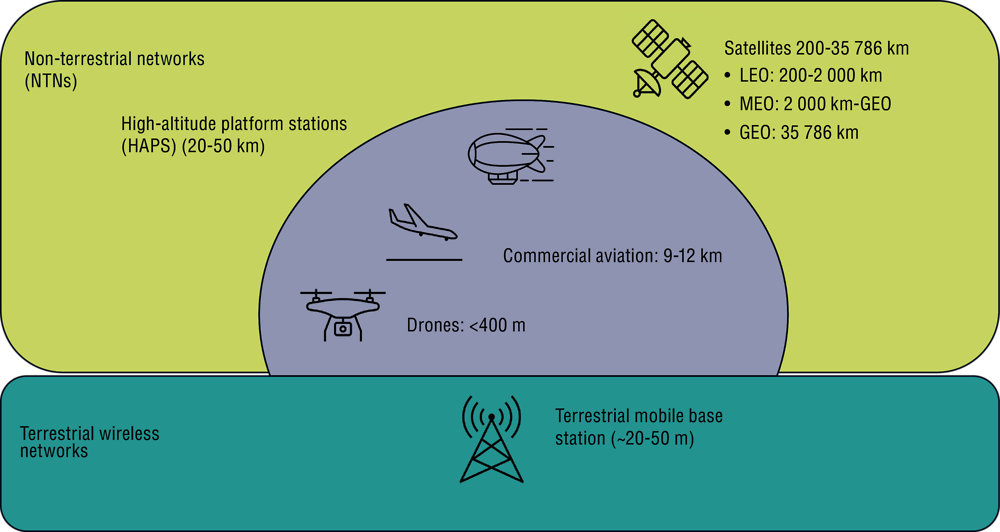
Note: LEO = Low Earth orbit; MEO = Medium Earth orbit; GEO = Geostationary orbit.
Source: Authors’ elaboration based on several sources, including: Ofcom (2021[19]), “Technology Futures – spotlight on the technologies shaping communications for the future”, European Space Agency (2020[61]), “Types of orbits”; GSMA (2021[62]), “High Altitude Platform Systems: Towers in the skies”.
Box 1.S.1. Flying taxis with electric vertical take-off and landing aircrafts for future transportation
Copy link to Box 1.S.1. Flying taxis with electric vertical take-off and landing aircrafts for future transportationResearch continues into the integration of terrestrial and non-terrestrial networks that would marry airborne transport systems with urban air mobility together with flying vehicles. Several manufacturers plan to launch commercial operations of electric vertical take-off and landing (eVTOLs) aircrafts, or “flying taxis”, around 2025-26. For example, companies working on developing eVTOLS include Airbus SE in France, Archer Aviation and Joby Aviation in the United States, Lilium and Volocopter in Germany and Vertical Aerospace in the United Kingdom (NASA, 2020[63]). The 2024 Paris Olympic Games aims to be the first to provide transportation with eVTOLs (Alcock, 2022[64]). The eVTOLs will be managed by a pilot on board and could eventually become autonomous.
The eVTOLS will take off and land at so-called vertiports.* With the growing number of unmanned aerial vehicles in the lower airspace, airspace management is critical. This is done through unmanned aircraft system traffic management (UTM) (FAA, 2023[65]). The UTM complements the Air Traffic Management (ATM) system, which manage aircrafts in the airspace.** The eVTOLs will have advanced flight decks with a communication system that combines legacy aviation systems with satellite and mobile communication networks. As such, different frequency bands allocated to mobile networks, satellite and aviation will be needed.
A fundamental shift in airspace regulation is under way in response to these developments. Moreover, security concerns of flying objects over cities need to be addressed to guarantee safe and reliable air traffic. The aviation sector foresees largely using commercial mobile networks to handle growing demand for data transmission from aircrafts. They would rely on legacy aviation systems as fall-back solutions (EASA, 2022[59]).
Notes:
* The European Union Aviation Safety Agency (EASA) defines a vertiport as “an area of land, water or structure used or intended to be used for the landing and take-off of eVTOL aircraft” (EASA, 2022[59]).
** According to EASA, “an ATM aggregation of the airborne and ground-based functions (air traffic services, airspace management and air traffic flow management) is required to ensure the safe and efficient movement of aircraft during all phases of operations” (EASA, 2022[59]).
Connectivity provided by terrestrial mobile communication operators can be an interesting solution for the aviation sector. At the very least, it could support communication services that are not mission critical and as such could be offloaded from their legacy systems. This would be more cost effective than developing propriety systems for the aviation sector (EASA, 2022[59]).
One example of ongoing research in this field is the European Union-financed Single European Sky Air Traffic Management Research (SESAR) 3 Joint Undertaking. SESAR 3 aims to modernise Europe’s air and ground Air Traffic Management (ATM) infrastructure and operational procedures. In so doing, it would contribute to smarter, more sustainable, better connected and more accessible air transport systems (SESAR 3, 2022[66]). Under that scenario, multi-layer broadband connectivity (e.g. future 6G networks) would need some form of integration with digitalised aviation systems. The expansion of communication services in airborne networks, along with future air urban mobility, drones and other unmanned air vehicles, will drive demand for a wide range of broadband communication services. The goal is to allow for limitless communication in and between terrestrial, airborne and satellite networks (Celtic-Next, 2022[67]).
The satellite industry is seeking to develop new business models and connectivity solutions. Although the inclusion of satellite in the 3GPP standard has led to more interaction between terrestrial mobile operators and satellite companies, more opportunities and partnerships may arise in the future. Examples of recent partnerships include Starlink with operators KDDI and Salt (KDDI, 2022[68]; Swinhoe, 2023[69]), and OneWeb with the operator VEON (OneWeb, 2023[70]). Kuiper has also announced agreements with Verizon and Vodafone to extend the reach of their mobile networks (Vodafone, 2023[71]; Verizon, 2021[72]).
The development of user terminals that follow standardised radio interfaces, offering seamless connection on both terrestrial and satellite networks, is paving the way for a more integrated ecosystem between TNs and NTNs. Countries and industry players would do well to follow the process for evaluating IMT Terrestrial and Satellite Radio Interfaces led by the ITU-R Working Parties 5D and 4B (ITU-R, 2022[73]).
The foundation for hybrid topology wireless networks that integrate terrestrial and non-terrestrial networks rests on a combination of three elements: an aviation sector facing increased data demand, a satellite sector looking for new use cases and a terrestrial wireless communication sector wrestling with exponential growth in mobile traffic. Moreover, the combination of different connectivity solutions, relying on different technologies, industry structures, and customer interfaces, impacts market dynamics. This raises both technical and regulatory challenges related to regulatory collaboration, spectrum management, digital security and digital divides, among others.
Collaboration. Policy makers could facilitate a constructive regulatory collaboration between three distinct sectors: space, aviation and mobile communications. Responsibilities are currently divided between different sector authorities.
Spectrum policy. The emergence of hybrid topology networks extending across national borders and stretching from the ground through aerospace may call for more dynamic and flexible access to spectrum and improved spectrum sharing to provide benefits to all users.
Digital security. Apart from physical security and safety issues for traditional aircrafts and other flying objects, digital security is of great concern for the next generation of wireless networks. This could also involve satellite communication and data processing in cloud solutions in the airspace.
Digital divides. Coupled with the promising technology advances shaping the connectivity ecosystem this next decade, policy makers need to continue bridging connectivity divides through the expansion of high-quality broadband infrastructure and services at competitive prices.
References
[76] 3GPP (2023), “Introducing 3GPP”, webpage, https://www.3gpp.org/about-us/introducing-3gpp (accessed on 5 March 2023).
[11] 3GPP (2023), “Releases”, webpage, https://www.3gpp.org/specifications-technologies/releases (accessed on 5 March 2023).
[23] 6G Flagship (2023), “Verticals – 6G Flagship”, webpage, https://www.6gflagship.com/research/verticals (accessed on 6 March 2023).
[13] 6GSymposium (2021), “6GSymposium Europe: Shaping Industry and Society Beyond 6G”, webpage, https://www.6gworld.com/spring-2021-6g-symposium-agenda (accessed on 17 March 2023).
[8] Ahokangas, P., M. Matinmikko-Blue and S. Yrjölä (2023), “Envisioning a future-proof global 6G from business, regulation, and technology perspectives”, IEEE Communications Magazine, Vol. 61/2, pp. 72-78, https://doi.org/10.1109/MCOM.001.2200310.
[64] Alcock, C. (2022), “Quiet flights will be key to eVTOL aircraft victory at 2024 Paris Olympic Games”, 18 April, FutureFlight, https://www.futureflight.aero/news-article/2022-04-15/quiet-evtol-flights-will-be-benchmark-olympic-gold-paris-2024-games.
[20] Alleven, M. (2021), “If mmWave sounds crazy, just wait for 6G and Terahertz”, Special Report, 30 July, Fierce Wireless, https://www.fiercewireless.com.
[29] Amazon (2023), “All systems go: Amazon confirms 100% success rate for Project Kuiper Protoflight mission”, 16 November, Press Release, https://www.aboutamazon.com/news/innovation-at-amazon/amazon-project-kuiper-protoflight-mission-november-2023-update.
[34] Apple (2023), “Use Emergency SOS via Satellite on your iPhone 14 – Apple Support”, webpage, https://support.apple.com/en-us/HT213426 (accessed on 7 March 2023).
[51] Araripe d’Oliveira, F., F. Cristovão Lourenço de Melo and T. Campos Devezas (2016), “High-altitude platforms — Present situation and technology trends”, Technology Management, Vol. 8/3, pp. 249-262, https://www.scielo.br/j/jatm/a/JQv95PgKcDCtrn95vLLV8qN/?format=pdf&lang=en.
[60] Bloomberg (2022), “Paris Opens Flying Taxi Hub Targeting Flights for 2024 Olympics”, Bloomberg, https://www.bloomberg.com/news/articles/2022-11-10/paris-opens-flying-taxi-hub-targeting-flights-for-2024-olympics (accessed on 14 November 2022).
[18] Bourdoux, A. et al. (2020), “6G White Paper on localization and sensing”, Research Visions, No. 12, 6G Flagship, https://www.6gflagship.com/6g-white-paper-on-localization-and-sensing.
[35] Browne, R. (2023), “Samsung turns to space with satellite-enabled smartphone chip”, 23 February, CNBC, https://www.cnbc.com/2023/02/23/samsung-turns-to-space-with-satellite-enabled-smartphone-chip.html.
[67] Celtic-Next (2022), Project 6G-SKY, website, https://www.celticnext.eu/project-6g-sky (accessed on 22 February 2023).
[77] CEPT (2022), “CEPT Workshops – CEPT Workshop on Satellite Innovations and Regulatory Challenges”, webpage, https://www.cept.org/ecc/tools-and-services/cept-workshops/cept-workshop-on-satellite-innovations-and-regulatory-challenges (accessed on 5 March 2023).
[75] Chuberre, N. and C. Michel (2018), “Satellite components for the 5G system”, 4 January, 3GPP, https://www.3gpp.org/news-events/3gpp-news/sat-ntn.
[40] Clark, M. (2023), “The FCC wants to get satellite-to-smartphone service rolling”, 16 March, The Verge, https://www.theverge.com/2023/3/16/23643215/fcc-satellite-to-smartphone-regulation-proposal.
[59] EASA (2022), Future Connectivity for Aviation, EU/US Task force White Paper, European Union Aviation Safety Agency, https://www.easa.europa.eu/en/document-library/general-publications/future-connectivity-aviation.
[10] Ericsson (2022), “6G – Connecting a cyber-physical world”, White Paper, Ericsson, https://www.ericsson.com/en/reports-and-papers/white-papers/a-research-outlook-towards-6g.
[56] Erwin, S. (2021), “Lockheed Martin signs agreement with Omnispace to explore 5G in space”, 23 March, Spacenews, https://spacenews.com/lockheed-martin-signs-agreement-with-omnispace-to-explore-5g-in-space.
[61] ESA (2020), “Types of Orbits”, webpage, https://www.esa.int/Enabling_Support/Space_Transportation/Types_of_orbits#GEO (accessed on 5 March 2023).
[15] ETSI (2022), “ETSI launches a new group on Terahertz, a candidate technology for 6G”, 12 December, Press Release, ETSI Group, https://www.etsi.org/newsroom/press-releases/2158-etsi-launches-a-new-group-on-terahertz-a-candidate-technology-for-6g?jjj=1676631829546.
[44] European Commission (2022), “Commission welcomes political agreement to launch IRIS²”, European Commission, Brussels, https://ec.europa.eu/commission/presscorner/detail/en/ip_22_6952?utm_source=Ookla%20Insights&utm_medium=email&utm_campaign=Express_2023-02-22_09:00:00&utm_content=Starlink%20Resurgence.
[42] European Commission (2022), “Space: EU initiates a satellite-based connectivity system and boosts action on management of space traffic for a more digital and resilient Europe”, 15 February, Press Release, European Commission, Brussels, https://ec.europa.eu/commission/presscorner/detail/en/IP_22_921.
[43] Evroux, C. (2023), “EU secure connectivity programme 2023-2027: Building a multi-orbital satellite constellation”, Briefing, European Parliament, https://www.europarl.europa.eu/thinktank/en/document/EPRS_BRI(2022)729442?utm_source=Ookla%20Insights&utm_medium=email&utm_campaign=Express_2023-02-22_09:00:00&utm_content=Starlink%20Resurgence?%20Speeds%20Increase%20in%20Europe%20and%20Oceania.
[65] FAA (2023), “Unmanned Aircraft System Traffic Management (UTM)”, webpage, https://www.faa.gov/uas/research_development/traffic_management (accessed on 6 March 2023).
[39] FCC (2023), “FCC proposes framework to facilitate supplemental coverage from space”, 16 March, Press Release, Federal Communications Commission, Washington, D.C., https://www.fcc.gov/document/fcc-proposes-framework-facilitate-supplemental-coverage-space.
[38] FCC (2022), “FCC takes latest step to improve satellite application process”, 22 December, Federal Communications Commission, Washington, D.C., https://www.fcc.gov/document/fcc-takes-latest-step-improve-satellite-application-process.
[55] Fletcher, B. (2021), “Musk says Starlink ‘nice complement’ to fiber, 5G”, 29 March, Fierce Wireless, https://www.fiercewireless.com/wireless/musk-says-starlink-satellite-broadband-complements-fiber-5g.
[14] G7 (2023), G7 Digital and Tech Track Annex 2: G7 Vision for future networks in the Beyond 5G/6G era, https://www.soumu.go.jp/joho_kokusai/g7digital-tech-2023/topics/pdf/pdf_20230430/annex2.pdf.
[79] German Federal Ministry of Education and Research (2023), 6G TakeOff: Holistic 3D communication networks for 6G, https://www.forschung-it-sicherheit-kommunikationssysteme.de/projekte/6g-takeoff.
[16] GSMA (6 February 2023), “Setting the stage for 6G”, GSMA Spectrum blog, https://www.gsma.com/spectrum/setting-the-stage-for-6g.
[62] GSMA (2021), “High altitude platform systems: Towers in the skies”, White Paper, June, GSMA, https://www.gsma.com/futurenetworks/wp-content/uploads/2021/06/GSMA-HAPS-Towers-in-the-skies-Whitepaper-2021.pdf.
[54] HAPS Mobile (2023), HAPS Mobile, website, https://www.hapsmobile.com/en (accessed on 20 March 2023).
[1] Hexa-X (2022), “A flagship for B5G/6G vision and intelligent fabric of technology enablers connectinghuman, physical, and digital worlds”, Hexa X, https://hexa-x.eu/wp-content/uploads/2021/05/Hexa-X_D1.2.pdf.
[31] Howell, E. (2022), “SpaceX launches 54 upgraded Starlink satellites, lands rocket in 60th flight of 2022”, 28 December, Space.com, https://www.space.com/spacex-starlink-satellites-5-1-group-launch.
[57] HT Group (2023), “Cooperation between Deutsche Telekom and the European Space Agency with a world premiere in Croatia”, 2 February, Press Release, European Space Agency (ESA) and Deutsche Telekom, https://www.t.ht.hr/en/Press/press-releases/6727/Cooperation-between-Deutsche-Telekom-and-the-European-Space-Agency-with-a-world-premiere-in-Croatia.html.
[21] IEEE (ed.) (2022), IEEE Future Networks, Enabling 5G and Beyond: 6G Activities in Germany, https://futurenetworks.ieee.org/tech-focus/december-2022/6g-activities-in-germany.
[26] IEEE (2022), “Official IEEE 802.11 Working Group Project Timelines – 2022-02-23”, webpage, https://www.ieee802.org/11/Reports/802.11_Timelines.htm (accessed on 2 March 2022).
[32] IETF (2022), “Problems and requirements of satellite constellation for Internet”, presentation at meeting 114, November, Internet Engineering Task Force, Datatracker.
[27] ITU (2023), Measuring Digital Development: Facts and Figures 2023, International Telecommunication Union, Geneva, https://www.itu.int/hub/publication/d-ind-ict_mdd-2023.
[49] ITU (2022), “HAPS – High-Altitude Platform Systems”, webpage, https://www.itu.int/en/mediacentre/backgrounders/Pages/High-altitude-platform-systems.aspx (accessed on 8 March 2022).
[45] ITU (2022), “ITU and space: Ensuring interference-free satellite orbits in LEO and beyond”, 8 February, International Telecommunication Union, Geneva, https://www.itu.int/hub/2022/02/itu-space-interference-free-satellite-orbits-leo (accessed on 8 March 2022).
[3] ITU (2021), “Beyond 5G: What’s next for IMT?”, 2 February, International Telecommunication Union, Geneva, https://www.itu.int/en/myitu/News/2021/02/02/09/20/Beyond-5G-IMT-2020-update-new-Recommendation.
[5] ITU-R (2023), New Recommendation ITU-R M.2160 on the “IMT-2030 Framework”, November, https://www.itu.int/en/ITU-R/study-groups/rsg5/rwp5d/imt-2030/Pages/default.aspx.
[4] ITU-R (2022), Meeting Report No. 41 Attachment 2.12 to Chapter 2 of Document 5D/136, Working Party 5D: Workshop “ITU for 2030 and beyond”, 14 June, https://www.itu.int/en/ITU-R/study-groups/rsg5/rwp5d/Pages/wsp-imt-vision-2030-and-beyond.aspx.
[48] ITU-R (2022), “Unlocking the potential of the stratosphere”, Working Party 5D: Workshop on “IMT for 2030 and Beyond”, HAPS Alliance, 14 June, https://www.itu.int/en/ITU-R/study-groups/rsg5/rwp5d/Pages/wsp-imt-vision-2030-and-beyond.aspx.
[73] ITU-R (2022), Vision, Requirements and Evaluation Guidelines for Satellite Radio Interface(s) of IMT-2020, International Telecommunication Union, Geneva, https://www.itu.int/hub/publication/r-rep-m-2514-2022.
[7] ITU-R (2022), “Workshop on ‘IMT for 2030 and Beyond’”, webpage, https://www.itu.int/en/ITU-R/study-groups/rsg5/rwp5d/Pages/wsp-imt-vision-2030-and-beyond.aspx (accessed on 6 March 2023).
[46] ITU-R (2020), Radio Regulations 2020, International Telecommunication Union, Geneva, https://www.itu.int/en/myitu/Publications/2020/09/02/14/23/Radio-Regulations-2020.
[74] ITU-R (1997), “Final Acts WRC-97”, World Radiocommunication Conference, Geneva, https://www.itu.int/dms_pub/itu-r/opb/act/R-ACT-WRC.5-1997-PDF-E.pdf.
[68] KDDI (2022), “KDDI launches the 1st mobile tower powered by SpaceX’s Starlink in Japan”, 1 December, KDDI Corporation, https://news.kddi.com/kddi/corporate/english/newsrelease/2022/12/01/6415.html.
[6] LightReading (2023), 6GHz, satellites and 6G addressed at WRC-23, https://www.lightreading.com/6g/6ghz-satellites-and-6g-addressed-at-wrc-23#close-modal.
[30] McDowell, J. (2024), “Enormous ‘mega’ satellite considerations”, webpage, https://planet4589.org/space/con/conlist.html (accessed on 5 March 2023).
[36] McGregor, J. (2023), “MediaTek brings practical satellite communications to mobile devices”, 24 February, Forbes, https://www.forbes.com/sites/tiriasresearch/2023/02/24/mediatek-brings-practical-satellite-communications-to-mobile-devices/?sh=4cab78c632be.
[41] MinTIC (2022), “Resolución número 000376 del 3 de febrero de 2022”, [Resolution Number 000376, 3 February], https://www.mintic.gov.co/portal/715/articles-198598_resolucion_00376_2022_v20220204.pdf.
[63] NASA (2020), “STEM Learning: Advanced Air Mobility: What is AAM? Student Guide”, National Aeronautics and Space Administration, Washington, D.C., https://www.nasa.gov/sites/default/files/atoms/files/what-is-aam-student-guide_0.pdf.
[2] OECD (2022), “Broadband networks of the future”, OECD Digital Economy Papers, No. 327, OECD Publishing, Paris, https://doi.org/10.1787/755e2d0c-en.
[24] OECD (2022), “Developments in spectrum management for communication services”, OECD Digital Economy Papers, No. 332, OECD Publishing, Paris, https://doi.org/10.1787/175e7ce5-en.
[47] OECD (2021), “Bridging connectivity divides”, OECD Digital Economy Papers, No. 315, OECD Publishing, Paris, https://doi.org/10.1787/e38f5db7-en.
[78] OECD (2019), “The road to 5G networks: Experience to date and future developments”, OECD Digital Economy Papers, No. 284, OECD Publishing, Paris, https://doi.org/10.1787/2f880843-en.
[28] OECD (2017), “The evolving role of satellite networks in rural and remote broadband access”, OECD Digital Economy Papers, No. 264, OECD Publishing, Paris, https://doi.org/10.1787/7610090d-en.
[17] Ofcom (2023), Spectrum Management for Next Generation Wireless Broadband: Flexible Access and Spectrum Sharing, 18 October, Ofcom, https://www.ofcom.org.uk/__data/assets/pdf_file/0036/269784/next-generation-wireless-broadband-oct-23.pdf.
[19] Ofcom (2021), Technology Futures: Spotlight on the Technologies Shaping Communications for the Future, 14 January, Ofcom, https://www.ofcom.org.uk/__data/assets/pdf_file/0011/211115/report-emerging-technologies.pdf.
[70] OneWeb (2023), “VEON and OneWeb partner to deliver seamless communication and digital services”, 1 March, Press Release, OneWeb, https://oneweb.net/resources/veon-and-oneweb-partner-deliver-seamless-communication-and-digital-services.
[25] Oughton, E. et al. (2021), “Revisiting wireless Internet connectivity: 5G vs. Wi-Fi 6”, Telecommunications Policy, Vol. 45/5, https://doi.org/10.1016/j.telpol.2021.102127.
[58] Ozger et al. (2023), “6G for connected sky: 6G-SKY visions to integrate terrestrial and non-terrestrial Networks”, paper submitted to EUCNC 6G Summit, Gothenburg, Sweden, 6-9 June.
[12] Policy Tracker (23 January 2023), “Our new podcast: Spectrum Policy 101”, Policy Tracker blog, https://www.policytracker.com/blog/our-new-podcast-spectrum-policy-101.
[37] Qualcomm (2023), “Qualcomm Introduces Snapdragon satellite, the world’s first satellite-based solution capable of supporting two-way messaging for premium smartphones and beyond”, 5 January, Press Release, Qualcomm, https://www.qualcomm.com/news/releases/2023/01/qualcomm-introduces-snapdragon-satellite--the-world-s-first-sate.
[9] Qualcomm (2022), Vision, Market Drivers, and Research Directions on the Path to 6G, December, Qualcomm, https://www.qualcomm.com/content/dam/qcomm-martech/dm-assets/documents/Qualcomm-Whitepaper-Vision-market-drivers-and-research-directions-on-the-path-to-6G.pdf.
[52] Reynolds, M. (2018), “Facebook and Google’s race to connect the world is heating up”, 26 July, Wired UK, https://www.wired.co.uk/article/google-project-loon-balloon-facebook-aquila-internet-africa.
[50] RSPG (2021), RSPG Opinion on a Radio Spectrum Policy Programme (RSPP) RSPG21-033 FINAL 2, RSPG, https://rspg-spectrum.eu/wp-content/uploads/2021/06/RSPG21-033final-RSPG_Opinion_on_RSPP.pdf.
[66] SESAR 3 (2022), SESAR 3 Joint Undertaking, website, https://www.sesarju.eu (accessed on 14 February 2023).
[53] Singh, M. (2021), “Alphabet shuts down Loon internet balloon company”, 21 January, TechCrunch, https://techcrunch.com/2021/01/21/google-alphabet-is-shutting-down-loon-internet.
[69] Swinhoe, D. (2023), “SpaceX’s Starlink signs direct-to-cell deal with Swiss telco Salt”, 3 March, Datacenter Dynamics, https://www.datacenterdynamics.com/en/news/spacexs-starlink-signs-direct-to-cell-deal-with-swiss-telco-salt.
[33] T-Mobile (2022), “T-Mobile takes coverage above and beyond with SpaceX”, 25 August, Press Release, T-Mobile, https://www.t-mobile.com.
[72] Verizon (2021), “5G + LEO: Verizon and Project Kuiper team up to develop connectivity solutions”, 26 October, Press Release, https://www.verizon.com/about/news/5g-leo-verizon-project-kuiper-team.
[71] Vodafone (2023), “Vodafone and Amazon’s Project Kuiper to extend connectivity in Africa and Europe”, 5 September, Press Release, https://www.vodafone.com/news/technology/vodafone-and-amazons-project-kuiper-extend-connectivity-africa-and-europe.
[22] Wireless World Research Forum (2022), “WWRF vision for ‘IMT 2030 and beyond’”, presentation at the ITU-R WP5D workshop on “IMT for 2030 and Beyond”, 14 June.
Notes
Copy link to Notes← 1. According to 3GPP, NTN refers to networks, or segments of networks, using an airborne or spaceborne vehicle for transmission (Chuberre and Michel, 2018[75]).
← 2. The ITU Radio Regulations defines terrestrial radiocommunication as any radiocommunication other than space radio communication or radio astronomy. See ITU Radio Regulations, article 1, section 1 “General terms”, Sub-section 1.7 (ITU-R, 2020[46]).
← 3. Based on the CISP Regulatory questionnaire for the DEO and upcoming reports 2023-24, where 37 of 38 OECD countries replied.
← 4. With previous mobile generations, such as 4G and 5G, 8-12 years elapsed between setting the “technology” vision and agreeing upon the technological standard and the first commercial deployments. For example, for 5G, the ITU-R Working Party 5D defined the vision for IMT-2020 (5G) in 2012, and the first commercial deployments started in April 2019, with many networks launching during 2020.
← 5. Non-Standalone (NSA) 5G means that much of the network will rely on presently deployed 4G technology, but that devices can use the “5G New Radio” standard in the Radio Access Network (RAN). Two important milestones of the 5G standardisation process by 3GPP include the “non-standalone 5G” (NSA-5G) specifications, completed in December 2017, and the “standalone 5G” (SA-5G) specifications completed in June 2018. These two milestones are part of 3GPP’s Release 15 of the “5G standard” and marked the first phase of the 5G standardisation process complying with the ITU IMT-2020 requirements. Therefore, the difference between NSA-5G and SA-5G is that the former requires current 4G core network equipment to be deployed, and the latter requires deploying an entire new network (OECD, 2019[78]).
← 6. The 3rd Generation Partnership Project (3GPP) unites seven telecommunications standard development organisations (ARIB, ATIS, CCSA, ETSI, TSDSI, TTA, TTC). 3GPP specifications cover cellular telecommunications technologies, including radio access, core network and service capabilities (3GPP, 2023[76]).
← 7. In addition, equipment and devices capable of operating in the terahertz range will rely on advanced semiconductors potentially increasing the challenges for spectrum monitoring.
← 8. For more information on the latest developments in spectrum policy in OECD countries, see OECD (2022[24]).
← 9. Historically, radar or sensing systems were developed independently from mobile communication technologies. There is growing interest in merging these two technologies. Namely, the use of mmWave or terahertz frequencies used in combination with massive MIMO and machine-learning techniques may enable a finer spatial resolution to detect even subtle movements, such as finger gestures. Communication systems using terahertz spectrum may allow for very high throughput and very low latency, providing an opportunity to conceive joint communication, sensing and positioning systems. Thus, it is a promising technology for future 6G systems (Ofcom, 2021[19]).
← 10. Wi-Fi 6 refers to the networking standard IEEE 802.11ax.
← 11. Wi-Fi 7 refers to the networking standard IEEE 802.11be.
← 12. This would enable a move from wide beams to multiple smaller spot beams and concentrate power on a smaller area, increasing link performance, as well as making it possible to reuse spectrum multiple times, increasing the amount of capacity per satellite.
← 13. The allocation of spectrum for Inter satellite network links in satellite bands around 12, 18 and 28 GHz were part of the ITU’s World Radiocommunication Conference 2023 (WRC-23) Agenda Item 1.17. WRC-23 took place on 20 November-15 December 2023.
← 14. 3GPP Release 17 (frozen in 2022) offers two forms of satellite communications to mobile devices: IoT-NTN, which will provide low data-rate narrow band communications, and NR-NTN, which will provide broadband communications for high data-rate communications, such as video calls (McGregor, 2023[36]).
← 15. Different stakeholders are engaging on this topic, such as the European Conference on Postal and Telecommunication Administrations (CEPT) (CEPT, 2022[77]).
← 16. The United Nations Committee on the Peaceful Uses of Outer Space is responsible for space object and space debris. At a national level, agencies or ministries in charge of space regulate space objects.
← 17. High-altitude platform stations are defined by the ITU’s Radio Regulations as radio stations located on an object at an altitude of 20-50 km and at a specified, nominal, fixed point relative to Earth (ITU-R, 2020[46]).
← 18. IMT-2020 are the requirements of the ITU-R in 2015 for 5G networks, devices and services.
← 19. The idea of using platforms in the stratosphere to provide communication services goes back to the end of the 1960s with the first experimental projects. R&D on aerial platforms, given different labels over time, have been ongoing ever since (Araripe d’Oliveira, Cristovão Lourenço de Melo and Campos Devezas, 2016[51]).
← 20. High-altitude platform stations (HAPS) are defined by the ITU’s Radio Regulations as radio stations located on an object at an altitude of 20-50 km and at a specified, nominal, fixed point relative to Earth (ITU-R, 2020[46]). The initial definition of HAPS was established at the World Radio Conference (WRC) 1997 and spectrum was allocated in the 47-48 GHz-band (ITU-R, 1997[74]). The ITU later redefined it.
← 21. A mobile service transmitting signals between aeronautical stations and aircraft stations.
← 22. The European Space Agency and DT agreed to work on hybrid networks of the future for more resilient and secure connectivity solutions (HT Group, 2023[57]).