This chapter focuses on the environmental potential of circular business models. Drawing primarily on insights from the lifecycle assessment (LCA) literature, it assesses and compares the environmental footprint of goods and services produced via circular modes of production to those produced via more traditional means. Thus, recycled materials are compared to those made from virgin natural resources, remanufactured products are compared to new products, and the sharing or leasing of assets is compared to conventional ownership. While the environmental potential of circular business models is found to be broadly positive, the analysis also identifies several risks and unintended consequences that could result from their more widespread adoption.
Business Models for the Circular Economy
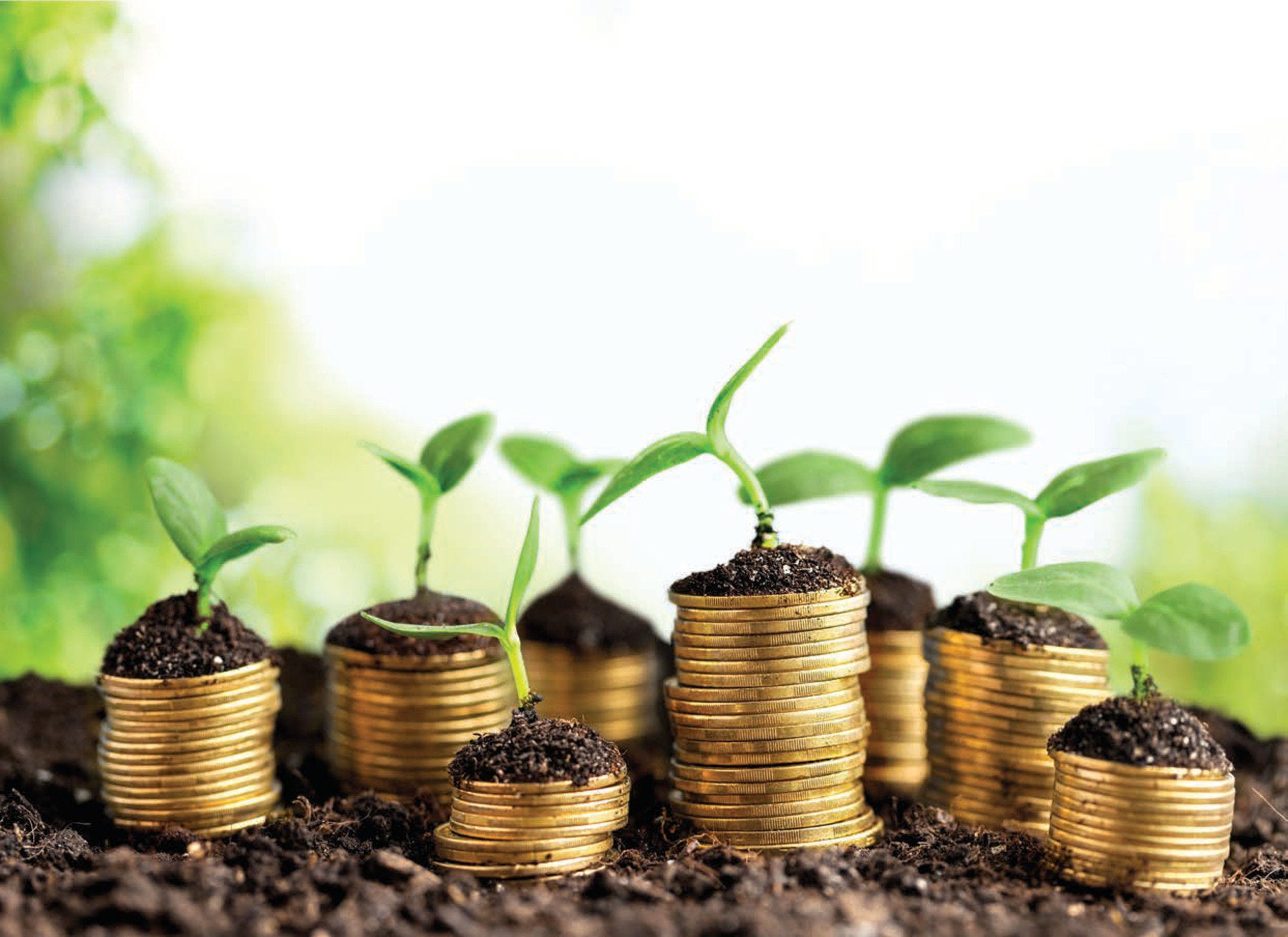
Chapter 4. The environmental impacts of circular business models
Abstract
4.1. Introduction
One reason for the recent interest in promoting a circular economy transition is the reduction in environmental impacts that could result. Circular business models, by closing resource loops and by slowing and narrowing resource flows, will, in theory, reduce the environmental footprint of production and consumption activities. That said, what is the evidence that these business models actually serve to displace resource extraction, processing, and disposal? And how does this translate into improved environmental outcomes? Which business models hold the greatest promise in this respect? These are the questions that this chapter attempts to address.
Establishing the environmental impacts associated with the emergence of circular business models is complex task for various reasons:
There are five business models considered in this paper, all of which have distinct sub-types. Each individual business model, or business model sub-type, operates in more than one economic sector. For example, sharing models are best known in the short term lodging and transport sectors, but are also relevant across a wider range of consumer products.1 This diversity makes it difficult to generalize about the likely environmental impacts.
The emergence of a particular business model in a specific economic sector will have implications across a range of environmental impact categories including, but not limited to, global warming, particulate air pollution, acidification, eutrophication, toxicity, and solid waste generation potential.
Changes in each environmental impact category can emerge at different points in the product lifecycle. For example, remanufacturing heavy machinery probably reduces the greenhouse gas emissions associated with upstream material extraction, transport, and processing but, to the extent that it results in an energy efficiency differential between new and remanufactured products, it can also affect the emissions during the machinery’s in-use phase.
The environmental impacts of a particular business model probably change as that business model emerges. For example, it is likely that the greenhouse gas mitigation potential associated with recycling will decrease at high recycling rates.
The emergence of a particular business model in a specific sector will lead to changes in relative prices and an array of indirect economic effects. The resulting changes in activity levels in other sectors will also have an environmental impact.
The intention in this chapter is to be as comprehensive as possible, however the above complexity serves to limit what can be feasibly covered.
The environmental impact associated with the emergence of a circular business model depends on both the market share that it captures (and the feedbacks this has on output from traditional modes of production, and on the wider economy) and on the relative environmental footprint of the circular and traditional modes of production. This chapter sets out to address both issues. Section 4.2 describes insights from intuitive approaches such as the circularity ladder concept. Section 4.3 draws on the life cycle analysis (LCA) literature to provide insights into the environmental footprint of different business models. The main weakness of these studies is their engineering approach; little attention is given to economic mechanisms and to the extent that circular modes of production will actually displace other activities. Section 4.4 therefore addresses economic effects, and particularly how the emergence of a particular circular business model might alter the structure of the broader economy.
4.2. Insights from the circularity ladder concept
The environmental desirability of different solid waste management options is embedded in the concept of a waste hierarchy (Box 4.1). In this framework, waste prevention is considered to be preferable to waste disposal because the environmental pressures associated with the mobilisation and disposal of materials are avoided. Different circular business models have different implications for the volume and ultimate destination of industrial and municipal solid wastes. Some business models – those relating to product life extension for example – probably serve to reduce the amount of waste generated. Others – resource recovery business models for example – are unlikely to influence waste generation, but will divert the waste that is generated away from disposal activities. Mapping the incidence of each circular business model to the waste hierarchy may therefore provide some insight into their relative environmental impacts.
Box 4.1. The waste hierarchy
The waste hierarchy is a concept that guides waste management policy in many OECD and non-OECD countries. Although the goal or objective of the waste hierarchy is rarely made explicit, it is often interpreted to be the minimization of the environmental impacts associated with the various solid waste management options (e.g. SEI, 2005 ([1])). Various versions of the hierarchy exist; the version embedded in the EU Waste Framework Directive (EU Directive 2008/98/EC) is perhaps the most cited. It ranks solid waste management options from most to least preferable as follows: (i) prevention, (ii) preparing for re-use, (iii) recycling, (iv) other recovery (e.g., energy), (v) disposal. In other words, from an environmental perspective, the priority should be to first limit the generation of waste, then to recycle and recover what is generated, and only then to dispose of what remains. This hierarchy is broadly consistent with the idea that the main objective of the circular economy is to maintain the value of products, components, and materials at their highest level.
This approach to assessing the relative environmental desirability of different circular business models has been undertaken by De Groene and Ethica (2015[2]), who term the resulting output the “circularity ladder” (Figure 4.1). Based on their work, product life extension and sharing models appear to be environmentally preferable to resource recovery business models. Product life extension and sharing business models, by slowing resource loops and narrowing resource flows respectively, have the potential to reduce the amount of waste generated. In contrast, the influence of resource recovery business models tends to be limited to diverting already existing waste towards material and energy recovery facilities. Product services can have an influence throughout the entire waste hierarchy. Product-oriented PSS variants, by including after sales service in the sales proposition, extend the life of products and prevent waste generation. Result-oriented PSS variants can have a similar impact since service providers have a greater incentive to use material inputs sparingly. Both result-oriented and user-oriented PSS variants, where product ownership is retained by the manufacturer, can create better incentives for recycling.
The circularity ladder approach provides a useful “first-pass” assessment of the relative environmental desirability of different circular business models. There are two main issues to be aware of. First, the waste hierarchy ranks waste management options according to the environmental impacts associated with the end of life phase of the product lifecycle; it is unclear whether the ranking remains valid when the entire product life-cycle is taken into account. For example, if the in-use environmental impacts of long-lived, energy intensive consumer products are considered, it may not always be the case that product repair is preferable to recycling, especially when new products have significant improve energy or water efficiency. Second, the circularity ladder provides little insight into the likely magnitude of the environmental benefits associated with different business models. Questions regarding the size of the potential greenhouse gas savings associated with remanufacturing activities, or the reduction in acidification potential associated with metal recycling, are better addressed by LCAs.
Figure 4.1. The circularity ladder concept

Source: Adapted from De Groene and Ethica (2015[2]), Boosting circular design for a circular economy, https://bit.ly/2phG1jl
4.3. Insights from the Life Cycle Assessment (LCA) literature
This section presents insights on the environmental footprints of different circular business models from the LCA studies (Box 4.2). This literature is extensive for some of the resource recovery, product life extension, and product service system business models, and the selection presented here should not be considered to be exhaustive. The circular supply and sharing business models have received relatively little attention in the LCA literature. In this section, the likely environmental footprint of circular supply models is therefore discussed alongside those for the resource recovery business model. The rationale for this is that these two business models, from an environmental perspective, can be seen as two sides to the same coin. The former involves product manufacturers switching to bio-based and/or recovered inputs while the latter involves the provision of some of these inputs. Finally, the discussion of the environmental footprint of sharing models, in the absence of LCA data, is more general and based on the underlying characteristics of that business model.
Box 4.2. Life cycle analysis: a brief summary
Life Cycle Assessment (LCA) is an internationally standardized methodology for establishing the environmental footprint of a particular product (good or service). Within the requirements of ISO 14040 and 14044, an LCA must comprise the following steps (WRAP, 2010[3]):
Goal and scope definition which defines the goal and intended use of the LCA, and scopes the assessment concerning system boundaries, function and flow, required data quality, technology and assessment parameters,
Inventory analysis (LCI) which consists in collecting data on inputs (resources and intermediate products) and outputs (emissions, wastes) for all the processes in the product system.
Impact assessment (LCIA), phase during which inventory data on inputs and outputs are translated into indicators of potential impacts on the environment, on human health, and on the availability of natural resources.
Interpretation of results where the results of the LCI and LCIA are interpreted according to the goal of the study and where sensitivity and uncertainty analysis are performed to qualify the results and the conclusions.
A LCA can either be undertaken for a product in isolation, or for one product relative to another. This distinction is important in the context of this chapter, which seeks to establish the environmental footprint of circular modes of production, either relative to other circular modes (recycling vs remanufacturing), or relative to more traditional modes (recycling vs primary material production). Because their underlying scope and assumptions often differ, it is generally difficult to compare the results from different LCA studies. As such, the data presented here are taken mostly from individual studies that effectively compare two LCAs: one for the footprint of the “circular” product, and one for the footprint of the “traditional” product. This is the origin of indicators like avoided resource extraction, energy use, and waste disposal.
Relative to the circularity ladder approach,2 the key strengths of LCA are its transparent methodology and its capacity to provide quantitative results. It is possible to assess the potential magnitude of the change in environmental footprint as well as its general direction. With respect to the mainly “comparative” LCAs considered in this chapter, the main weakness relates to assumptions about the nature of the counterfactual. For example, it is often assumed, when calculating the environmental impact of recycling, that the resulting unit of secondary output displaces one unit of primary output further upstream.3 This allows estimates of avoided material and energy extraction to be made, and these can then be converted into changes in global warming, acidification, eutrophication, and toxicity potential. As discussed further in Section 4.4, the extent to which output associated with circular modes of production actually displaces that associated with traditional production is largely determined by economic mechanisms.4 Assuming a one to one displacement of traditional output may be overly optimistic, especially when rebound and other indirect economic effects are accounted for.
4.3.1. Circular supply and resource recovery business models
General environmental considerations
Relative to a scenario involving disposal, transforming waste into secondary materials and using them as new inputs is widely considered to have positive first order environmental effects. By diverting waste towards recovery facilities, resource recovery business models reduce the volume of material that requires landfilling or incineration, and therefore the environmental impacts of these activities. Similarly, by increasing the supply of secondary materials, resource recovery business models can reduce demand for primary resources, and the environmental impacts associated with their extraction. Further, producing finished materials from waste typically requires considerably less energy and, depending on the energy mix, greenhouse gas emissions than doing so from virgin resources. For example, secondary copper and aluminum production requires only around 35% and 5% of the energy used in the respective primary processes (BIR, 2008[4]).
LCA data: the example of recycling
There are a vast number of LCAs that assess the environmental footprint of recycling relative to other end of life solid waste management options. Various meta-analyses of this body of work have been undertaken and, in some cases, comprise as many as several hundred individual studies (Table 4.1). Some meta-analyses focus on a particular material; plastics (HPRC, 2015[5]; Bernardo, Simões and Pinto, 2016[6]), paper and cardboard (EEA, 2006[7]; NCASI, 2012[8]), and organic waste (Morris, Matthews and Morawski, 2012[9]) are all common. Others focus on either a variety of materials (WRAP, 2010[10]; Valerio, 2010[11]; DSEWPC, 2012[12]; Laurent et al., 2014[13]), or on a composite waste product like municipal solid waste (Cleary, 2009[14]). In terms of the environmental impact categories considered, most individual studies assess the effect of recycling on resource consumption, energy use, and greenhouse gas emissions. Some studies also assess the impacts on acidification and eutrophication potential, eco-toxicity, and avoided solid waste disposal. Less than 20% of LCAs on solid waste management assess impacts on land and water use (Laurent et al., 2014[13]).
Table 4.1. Selected literature assessing the environmental impacts of recycling
Study |
Material focus |
# studies |
Environmental impact categories |
---|---|---|---|
EEA (2006[7]) |
Paper and Cardboard |
9 |
Resource consumption, energy use, GWP, AP, toxicity, solid waste |
WRAP (2006[15]) |
Various |
55 |
Resource consumption, energy use, water use, GWP |
Cleary (2009[14]) |
Waste |
20 |
Energy use, GWP, AP |
WRAP (2010[10]) |
Various |
>200 |
Resource consumption, energy use, water use, GWP |
Valerio (2010[11]) |
Various |
31 |
GWP, AP, EP, PCOP, toxicity |
NCASI (2012[8]) |
Paper |
41 |
? |
DSEWPC (2012[12]) |
Various |
5 |
Resource consumption, energy use, water use, GWP, EP, PCOP, solid waste |
Morris et al. (2012[9]) |
Organics |
82 |
GWP, AP, human toxicity, eco toxicity |
Astrup et al. (2015[16]) |
Waste |
250 |
? |
Laurent et al. (2014[13]) |
Various |
222 |
Varies according to individual study |
HPRC (2015[5]) |
Plastics |
17 |
GWP, AP, EP |
Bernardo et al. (2016[6]) |
Plastics |
20 |
Energy use, GWP |
Note: GWP = global warming potential, AP = acidification potential, EP = eutrophication potential, PCOP = Photochemical oxidation potential
Source: Various
The assumptions underlying individual LCAs vary considerably; a useful summary is provided by Laurent et al. (2014[13]). One key issue relates to the multi-functional character of material and energy recovery processes. For example, the environmental impacts of recycling result from both its waste management function (avoided disposal) as well as its role in the production of secondary commodities (avoided virgin resource extraction and processing). Assumptions about the volume (how much waste is recovered as opposed to disposed of?) and type (how substitutable are the recovered secondary materials for their primary equivalents?) of avoided resource extraction will significantly influence results. According to Laurent et al. (2014[13]), “most reviewed studies assume a substitution ratio of 1:1 and/or a quality similar to the substituted product”. By ignoring the existence of down-cycling, this approach can lead to overestimates of the environmental benefits resulting from material recovery.5 Other key issues that are highlighted include the treatment of capital goods (are the environmental impacts associated with the construction of material recovery infrastructure accounted for?), and collection and transport (are the environmental impacts associated with these activities accounted for?).
The key high level conclusion that emerges from the meta-analyses presented in Table 4.1 is that recycling has a small environmental footprint relative to traditional disposal activities.6 WRAP (2006[15]) assessed 55 “state of the art” LCAs of paper and cardboard, glass, plastics, aluminium, steel, wood, and aggregates, and conclude that “most studies show that recycling offers more environmental benefits and lower environmental impacts than the other waste management options”. On the basis of 222 individual studies, Laurent et al. (2014[13]) state that “ … for waste paper, all studies favour recycling or thermal treatment over landfilling” and “ … a relatively large proportion of studies tend to favour recycling over landfilling and thermal processes for plastics and paper”. Bernardo, Simoes and Pinto (2016[6]) focussed on LCAs of plastics and found that “the results reported are generally consistent, showing that recycling has the lowest environmental impact on Global Warming Potential (GWP) and Total Energy Use (TEU) impacts”.
Several of the meta-analyses present caveats to the conclusion that the environmental footprint of recycling is smaller than that for traditional disposal. Some caveats relate to specific materials. WRAP (2010[10]) state that, “for wood and textiles, more studies are needed to be able to make firmer conclusions regarding the environmental benefits of recycling”. Other caveats relate to data limitations. NCASI (2012[8]) state that, “while many of the reviewed studies resulted in findings that suggest a lower LCA profile for recovery for recycling over landfilling as an end-of-life option for paper, the applicability of this finding is limited, given the extent to which it depends on assumptions regarding paper degradation in landfills and the methods used to account for biogenic carbon, and the relative weakness of current LCA toxicity-related impact assessment”.
It is also apparent from the existing literature that the environmental impacts of recycling vary significantly across materials. Figure 4.2 shows data from a LCA undertaken in New South Wales, Australia by the Department of Environment, Climate Change, and Water (NSW EPA, 2010[17]). Recycling of metals is shown to have considerably larger environmental benefits in terms of avoided greenhouse gas emissions, energy use, and water use than recycling of building materials, paper and cardboard, organics, glass, or plastics. This is probably a function of the extremely high energy and water intensity of crushing, beneficiating, and refining mineral ores into finished metal products.
Figure 4.2. Average net environmental benefits of recycling 1 tonne of waste in Australia
Finally, the environmental impacts of recycling are also likely to vary significantly with recycling rates. This issue is raised by Ekvall (2007[18]), who state that “the environmental burdens of collection and recycling are likely to be a non-linear function of the collection rate”, and, “at very high recycling rates, the required extra transport and processing of materials may increase fuel consumption and emissions greatly for each additional tonne of material that is collected”. In more concrete terms, it is easy to imagine that the environmental benefits of collecting recyclable materials from smaller rural communities and transporting them to distant processing centres could require more energy use than it saves. This, in tandem with the private costs and benefits of recycling, raises the possibility of an optimum recycling rate. For example, cost benefit analysis undertaken for Japan finds that the recycling rate that minimises the average social cost7 of municipal solid waste management is around 10%, which is significantly less than current recycling rates of 20% (Kinnaman, Shinkuma and Yamamoto, 2014[19]). Optimum recycling rates are likely to evolve over time as new technologies emerge and market prices change.
4.3.2. Product life extension models
General environmental considerations
Product life extension business models, whether classic long life, direct reuse, maintenance and repair, or refurbishment and remanufacturing, tend to slow the flow of products and materials through the economy. This is widely considered to be positive from an environmental viewpoint. Reduced demand for new products translates up the value chain into reduced extraction and processing of new resources, and down the value chain into reduced disposal. The associated environmental impact of these activities is thereby reduced.
There are at least three second order factors that may, at least partially, offset such environmental gains.8 First, the average environmental footprint of in-use products may increase if longer lived products slow the diffusion of new, relatively efficient designs. This is of particular concern for product categories characterized by large use phase impacts and rapid technological progress.9 Second, with the exception of the classic long life business model, product life extension processes also require inputs of materials and energy. For example, the environmental footprint of transporting a product to a repair or remanufacturing facility may be non-negligible. Third, if choosing repaired, second-hand, or remanufactured goods creates significant consumer savings, then there will also be potential for rebound effects. Essentially, the additional consumption resulting from increased disposable income also has an environmental footprint.
These three concerns apply to different extents for each of the four product life extension sub-models. For example, because higher prices are central to the classic long life business model, there is little potential for direct rebound effects. Further, because this business model (when considered in isolation) dispels with the need for post-sales repair or remanufacture, it also negates the environmental footprint of these activities (transport for example). As such, the classic long life business model has considerable potential from an environmental perspective, especially for product categories (such as clothing) where a low proportion of the lifecycle environmental footprint is associated with the use-phase (see DEFRA (2011[20]) for further discussion). More generally, it is unclear from the existing literature to what extent the environmental benefits associated with longer lived products (reduced extraction and processing of new resources, reduced disposal of end of life products) are offset by second order effects.
LCA data: the example of remanufacturing
There are a considerable number of LCAs that assess the environmental footprint of product remanufacturing, typically relative to a scenario involving product disposal and the purchase of an equivalent new product. The product focus in these studies is diverse, but common categories include automobiles (and their constituent parts), imaging equipment, and printer cartridges. A selection of this literature is presented in Table 4.2. Most studies focus on a limited subset of impact categories. Avoided energy use and greenhouse gas emissions are the most common, and avoided resource extraction and waste disposal are also occasionally assessed.
Table 4.2. Selected literature assessing the environmental impacts of remanufacturing
Author |
Study type |
Product Focus |
Change in resource extraction |
Change in energy consumption |
Change in GHG emissions |
Waste disposal |
---|---|---|---|---|---|---|
Kerr and Ryan (2001[21]) |
LCA |
Photocopiers |
-19% to -25% |
-27% |
-23% |
-35% |
Photocopiers (modular) |
-39% to -48% |
-68% |
-65% |
-47% |
||
Smith and Keoleian (2004[22]) |
LCA |
Engines |
-26% to -90% |
-68% to -83% |
-73% to -87% |
-65% to -88% |
Steinhilper (1998[23]) |
? |
Various |
- |
-85% |
- |
- |
Neto and Bloemhof (2009[24]) |
LCA |
Personal computers |
- |
-80% |
- |
- |
Kara (2010[25]) |
LCA |
Printer cartridges |
- |
- |
-33% |
- |
Gutowski et al. (2011[26]) |
Meta-review |
Furniture |
- |
-100% |
- |
- |
Clothing |
- |
-64% |
- |
- |
||
Computers |
- |
-57% |
- |
- |
||
Electric motors |
- |
3% |
- |
- |
||
Tires |
- |
9% |
- |
- |
||
Appliances |
- |
75% |
- |
- |
||
Engines |
- |
-4% |
- |
- |
||
Toner cartridges |
- |
-6% |
- |
- |
||
Warsen et al. (2011[27]) |
LCA |
Gearbox |
>50% |
-33% |
- |
- |
Biswas and Rosano (2011[28]) |
LCA |
Compressors |
- |
- |
-90% |
- |
Liu et al. (2014[29]) |
LCA |
Engines |
-95% |
-66% |
-67% |
- |
Wilson et al. (2014[30]) |
LCA |
Turbine blades |
- |
-36% |
-45% |
- |
ERN (2015[31]) |
Meta-review |
Aerospace |
- |
- |
-356,000 |
-136,000 |
Automotive |
- |
- |
-3,298,000 |
-902,000 |
||
EEE |
- |
- |
-177,000 |
-150,000 |
||
Furniture |
- |
- |
-131,000 |
-76,000 |
||
Heavy duty equipment |
- |
- |
-3,458,000 |
-855,000 |
||
Machinery |
- |
- |
-393,000 |
-35,000 |
||
Marine |
- |
- |
-40,000 |
-15,000 |
||
Medical equipment |
- |
- |
-58,000 |
-22,000 |
||
Rail |
- |
- |
-344,000 |
-69,000 |
||
Gao et al. (2017[32]) |
LCA |
Turbochargers |
- |
-82.50% |
-73% |
- |
Note: Change in GHG emissions and waste disposal in ERN (2015[31]) are at the European level due to current remanufacturing rates.
Note: The International Resource Panel is undertaking a major research project on remanufacturing, refurbishment, repair, and direct reuse. Among other outputs, this work includes calculations of the environmental impacts of life extension activities in the automobile, heavy machinery, and imaging equipment sectors. Final results were unavailable at the time of writing.
Source: Various
The key conclusion that emerges from the LCA literature is that remanufacturing can result in sectoral reductions in resource extraction and waste disposal that are often in the order of 80%. This, in turn, will translate into a variety of environmental impacts. The one that is most frequently evaluated in the literature is the avoided greenhouse gas emissions associated with extraction and processing of virgin resources into new products. Although the sectoral reductions in resource extraction, energy consumption, and greenhouse gas emissions shown in Table 4.2 seem large, they are consistent with the nature of remanufacturing. In many cases, it is the deterioration of a small number of parts or components, rather than a failure of the product itself, that leads to a loss of functionality. Remanufacturing worn out parts, while retaining the core of the original product, can therefore prevent the loss of a high proportion of the constituent materials, along with their embedded energy content.
As discussed in the introduction to this section, there are at least three second order effects that may partially offset the upstream environmental benefits associated with longer lived products. Two of these – the environmental footprint of transporting used cores, and the potential for rebound effects resulting from consumer savings – are largely ignored in the remanufacturing LCAs considered here. Further, most of the LCAs in Table 4.2 appear to assume zero recycling rates in their “disposal and buy a new product scenario” when, in reality, significant proportions of some end of life products are recycled (the chassis’ of end of life vehicles for example).
Gutowski et al. (2011[26]) is the only study considered here that accounts for the impact of remanufacturing on the diffusion of new product designs.10 One important conclusion that emerges from this work is that, for certain product categories, the relatively poor energy efficiency of retained (remanufactured) products can more than offset the energy savings associated with reduced material production and traditional manufacturing (e.g. Figure 4.3: products 20 to 25 – dishwashers, monitor screens, refrigerators, and some computers). The authors highlight that this risk is greatest for long-lived product categories characterised by high use-phase energy requirements and rapid efficiency improvements.
Figure 4.3. Life cycle energy use of remanufactured products relative to new equivalents

Note: Furniture (1 and 2), textiles (3 and 5), appliances (20, 23, 25), computers (4, 6, 7, 8, 21, 24), toner cartridge (10), engines (11, 12), electric engines (13, 14, 15, 17, 18, 19), tires (9, 22, 16).
In sum, remanufacturing, like other product life extension business models, leads to avoided resource extraction, processing, and disposal; the environmental damages resulting from these associated with these activities will therefore be reduced. What is less clear from this review is the extent to which these environmental benefits are offset by various second order effects, especially those associated with the use phase of the product life cycle.11 Because remanufacturing keeps existing products in circulation for longer, it may also slow the diffusion of new and relatively efficient product designs. Caution in promoting manufacturing is warranted when the affected product categories are characterized by: (i) long product lives, (ii) energy or water intensive during the use phase, and (iii) high rates of efficiency improvements.
4.3.3. Sharing models
General environmental considerations
Sharing models are based upon short term rental transactions of under-utilised economic assets or goods. They provide consumers with additional choice: instead of buying goods from a traditional business provider, it is possible to temporarily lease them from an individual. In terms of the environmental implications of this shift, there is a widely held view that, by facilitating the use of under-utilised goods, sharing models will have positive environmental impacts. Many platforms advertise themselves as green, and particularly as carbon-footprint reducing (e.g., Frenken and Schor, 2017 ([33])). Consumers often appear to share this opinion. A survey undertaken by PWC in 2016 found that 76% of surveyed individuals believed that “the sharing economy is better for the environment” (PWC, 2015[34]). Further, a survey undertaken by Bocker and Meelen (2017[35]) found that this perceived greenness was a key motivation for participating in certain sectors of the sharing economy.12
The idea underlying these attitudes seems to be that lower demand for new goods can reduce virgin resource extraction, material processing, and manufacturing, and therefore the environmental impacts associated with these activities. While there is likely to be some substance to this idea, the academic literature on these effects is more equivocal. There is little empirical evidence that sharing business models are, by definition, positive from an environmental viewpoint. Box 4.3 provides key conclusions from a set of recent assessments.
Box 4.3. Environmental impacts of sharing models: evidence from recent assessments
IDDRI (2014[36]): “the environmental impact of these (sharing) practices, in the way that they have developed today, is rarely obvious and there are many reasons to raise doubts: a degree of pragmatism is required” … and … “In particular, the analysis of the sustainability of the (sharing) models is hampered by the lack of studies and its dissemination. To quote the candid remarks of one expert, “it is hard to reason on the basis of very poor statistical data.”
The Economist (2015): “In many cases, the benefits of sharing economy services are that they reduce cost and improve convenience—both of which might, in fact, boost consumption”.
European Commission (2016[37]): “Available empirical evidence to date is very partial and inconclusive - in many cases, it is simply anecdotal and often presented by stakeholders in the current controversies. For example, Uber and Airbnb have released dozens of reports, the reliability of which could not be independently validated because the methodologies are not transparently illustrated and data are kept internal and not made accessible to researchers”.
Sierra Club (2017[38]): “Many well-meaning, environment-friendly people have bought the line that the sharing economy is green” and “When it comes to the “greenness” of the sharing economy, magical thinking and corporate press releases have replaced fact-based discourse”.
ADEME (2016[39]): “Collaborative consumption does not necessarily signify responsible consumption. Indeed, the environmental gain depends strongly on the traditional activity that the collaborative activity replaces.”
Trinomics (2017[40]): “To date, little objective and thorough research has been done on the true environmental impacts of the collaborative economy. Many studies only use anecdotal evidence or only took into account direct and indirect environmental impacts, but not the rebound effect, that is the effect of increased consumption because of a decrease in prices, extra money earned and saved through engaging in the collaborative economy instead of the traditional economy”.
Nordic Council of Ministers (2017[41]): “More efficient use of the resources through sharing initiatives could also contribute to environmental benefits, but this depends on how people change their behaviour and spend their savings from using the initiatives.”
Although robust data are unavailable, the environmental impacts associated with the continued emergence of sharing models will depend largely on at least three factors: (i) the effect that increased utilisation rates have on goods’ expected lifetimes, (ii) the composition of the additional consumption resulting from any financial savings,13 and (iii) the existence of any differences in the life-cycle environmental footprint of shared and traditional goods (e.g., IDDRI, 2014 ([36])). Given the previously mentioned lack of empirical data, the remainder of this section focusses on discussing each of these factors.
The effect that increased utilisation of spare capacity will have on demand for new goods, and therefore on demand for virgin resource inputs, will depend on how increased utilisation affects the expected lifetime of the relevant goods. If the expected life of a good is strongly related to how frequently it is used, then any increase in utilisation rates will lead to shorter product lives, more rapid product turnover, and a limited impact on overall demand.14 This scenario is probably most relevant for products like vehicles or personal computers. In contrast, if the expected life of a good is not strongly related to how frequently it is used (consider housing or household tools for example), then increased utilisation may well lead to reduced demand for new production.
Consumer surveys suggest that a key motivation for participating in the peer to peer markets is the availability of considerable savings (Böcker and Meelen, 2017[35]). In concrete terms, it is considerably cheaper for consumers to satisfy demand for goods, mobility, and short stay accommodation through leasing rather than buying. The resulting financial savings can result in a rebound effect, where the environmental footprint of new consumption at least partially offsets any environmental benefits resulting from sharing. Relatedly, there is some (mostly anecdotal) evidence that the earning opportunities made possible by the emergence of sharing models are actually stimulating investment in new capacity in certain markets. For example, it seems likely that some of the vehicles used by Uberpool drivers, and some of the apartments leased by Airbnb hosts, would never have been purchased in the absence of those platforms. Keeping these considerations in mind is important when considering the overall environmental impact of sharing models (especially the avoided resource extraction and processing associated with the displacement of traditional providers).
If shared goods and their traditionally marketed equivalents were identical, then the two factors discussed above would probably determine much of the environmental impact of sharing models. However, in reality, the goods involved are often not identical, and this means that they can have differing environmental footprints. This effect can work in both directions. For example, in the context of mobility, the shared vehicles that are listed on peer to peer platforms like Getaround are likely to have a higher emissions signature than the vehicles leased by traditional car rental agencies. In contrast, in the context of short term lodging, it is often argued that the rooms listed on platforms like Airbnb have a smaller energy and water footprint than those in traditional hotels (see below).
LCA and survey data: the example of sharing under-utilised accommodation
As noted above, there is little systematic research that has assessed the environmental impacts of sharing models. What does exist often focusses on these business models in the short term lodging segment of the hospitality sector. This section presents three such assessments. Some of this work has been commissioned by the platform operators themselves; the results should be interpreted with that in mind.
A 2016 study undertaken by the World Economic Forum (WEF) and the Massachusetts Institute of Technology (MIT) assesses the material savings that might be associated with the emergence of accommodation sharing (WEF, 2016[42]). Researchers were interested in the role that Airbnb played in Rio de Janeiro before, during, and after the 2014 Football World Cup and 2016 Olympic Games. The authors found that short-term Airbnb leases housed around 17% of the estimated 500 000 tourists who visited Rio de Janeiro during the Olympic Games. This was calculated to represent the equivalent of 257 newly built hotels, each of which “would have been a large undertaking in terms of financing and material use”. This study was a preliminary effort, but does serve to highlight two of the shortcomings that are common to other assessments of sharing models. First, by overlooking economic mechanisms, it probably overestimates the impact that Airbnb had on hotel construction and, by extension, on resource extraction and use.15 Second, this estimate of avoided resource extraction and use is not extended to more tangible environmental impact categories such as global warming, acidification, and toxicity potential. Not all resource extraction is necessarily “bad”; the impacts involved will vary significantly according to the types of materials involved and where they are extracted and processed.
A 2014 study commissioned by AirBnb focusses more on the comparative use phase environmental impacts of shared and traditional (hotel) short term accommodation (Cleantech, 2014[43]). The research was based on 8 000 survey respondents in Europe and North America; jurisdictions which currently make up the vast majority of Airbnb stays. The study found that Airbnb guests in these two geographic areas use 63 - 84% less energy and 12 – 57% less water per guest night than typical hotel guests.16 Based on 92% of the respondents reporting that they produced an equal or lower amount of waste than when at home, it was also concluded that Airbnb stays produce 28-53% less waste than hotel stays. On the basis of these reductions, it was calculated that Airbnb stays in Europe resulted in annual greenhouse gas savings equivalent to removing 200 000 cars from the road and annual water use savings equivalent to 1 100 Olympic size swimming pools.
A 2017 study undertaken by the Agence de l’Environnement et de la Matrise de l’Energie (ADEME) also assesses short term sharing relative to traditional hotel rooms (ADEME, 2016[39]). The authors find that the differential environmental impacts of this choice are difficult to evaluate because of the number of factors that require consideration. The importance of individual behaviour, the relative sizes of rooms and apartments associated with Airbnb and hotel stays, and the electricity mix in different host countries are all factors that need to be considered. One firm conclusion provided by ADEME is that the sharing of under-utlilised capacity in the short term lodging segment is most likely to be environmentally beneficial when it involves individual rooms rather than entire apartments. In this situation, the energy and water use required for lighting, heating, and cleaning will be shared across more than one individual.
4.3.4. Product-service systems
General considerations
Product service systems change patterns of asset ownership. Instead of purchasing a good or asset, consumers purchase the service(s) that the good or service provides: actual ownership often remains with the original manufacturer. This characteristic of PSS creates a new set of incentives around product design, product use, and product disposal, with associated implications for the life-cycle environmental footprint of affected goods.
From a manufacturer’s perspective, the adoption of the user-oriented or result-oriented PSS variants means assuming responsibility for product disposal at the end of life. In a similar way to extended producer responsibility schemes, this can create an incentive to design products that are amenable to life extension processes such as remanufacture, refurbishment, or repair. This incentive, which is likely to be positive from an environmental viewpoint, may be at least partially offset by three opposing effects.
It has been suggested that concerns about cannibalizing rentals of relatively new product lines can lead PSS providers to prematurely remove old products from the market. Agrawal et al. (2012[44]) argue that such behavior could contribute to shorter product lives relative to a situation where individuals seek to sell, rather than dispose of assets (e.g. cars in the second hand car market).
It is possible that consumers use leased goods less carefully than goods that they own (Kuo, 2011[45]) which, if true, would also contribute to shorter product lives.
As discussed in Section 3.3, the environmental implications of longer lived products are not entirely clear; the upstream environmental benefits resulting from any reduction in demand for virgin resources may be partially offset by an increased environmental footprint in the use phase.
The adoption of PSS also creates other environmentally favourable incentives. For manufacturers producing consumable goods like energy and chemicals, the adoption of the result-oriented PSS variant will create incentives for their more efficient use. This is nicely explained by (Tukker, 2015[46]), who states that “in result-oriented business models, the use of materials also becomes merely a cost factor – using more materials or creating more products does not lead to increased revenues”. In addition, from a consumer perspective, the pay for use tariff structure of the user-oriented PSS variant may create incentives for more efficient product use. Because the variable cost of product use is more prominent than when products are owned, there will be a tendency to use them relatively sparingly.
User-oriented PSS business models are also being increasingly adopted in the context of digital products: e-books, streamed music and film, and digital media. This has potentially significant environmental impacts because the material basis for these products becomes unnecessary. A subscription to Spotify or an online media outlet provides access to music and news without the need for CDs or newspapers. The key question in this context is the extent to which the associated reductions in resource extraction, processing, and eventual product disposal are offset by the increased resource mobilisation required for the development of digital infrastructure (data servers and cable networks) and personal electronic devices. Establishing the net environmental impact is challenging because of the different materials involved.
Despite the existence of a considerable body of work on the environmental implications of PSS, there is little empirical evidence that they result in tangibly lower environmental pressure. The most comprehensive meta-analysis to date (Tukker, 2015[46]) concludes that “PSS will not by definition be more resource-efficient or ‘circular’ than product systems” but that “result-oriented PSS offers the greatest prospect of radical resource efficiency gains”. Currently operating examples of result-oriented – chemical leasing (e.g., OECD, 2017 ([47])) or lighting or heating service contracts – are therefore worthy of additional attention. It is also likely that the environmental implications of the emergence of PSS will depend on the product category affected. Agrawal et al. (2012[44]) and Intlekofer, Bras and Ferguson (2010[48]) find that environmental benefits are more likely to emerge when the product category involved is characterized by high use phase impacts and rapid technological progress. The following example therefore focusses on PSS as applied to urban car sharing.
LCA and survey data: the example of urban car sharing schemes
There is a considerable body of work that assesses the environmental impacts of car sharing. A selection of this literature is presented in Table 4.3. Most studies focus on the environmental impacts related to vehicle ownership and use. Vehicle kilometers travelled (VKT) and the associated greenhouse gas emissions, along with the number of privately owned cars replaced by each shared vehicle are the most common impact categories. Changes in vehicle kilometers travelled are often viewed as a proxy for energy extraction and use, or for particulate emissions in urban environments. The number of privately owned cars replaced is viewed as having implications for urban land use patterns, congestion, and virgin resource extraction rates (see below). Few studies consider the potential rebound effect associated with savings resulting from car sharing. This new consumption will tend to offset any gains associated with shared mobility.
Most studies assessing the environmental impacts of car sharing use consumer surveys to establish how the existence of a car sharing scheme changes vehicle ownership and mobility decisions. Car sharing members are asked a variety of questions about how their driving behavior, vehicle ownership, and use of other transport modes change due to the introduction of a car sharing scheme. Typically, respondents are presented with a before and after scenario; they are asked to compare their current behavior with a counterfactual based on recollections of their past behavior.17 It is important to keep in mind the shortcomings of such an approach. First, individuals’ recollections of their past behavior may deviate from their actual behavior; any systematic deviation will tend to bias the results of the subsequent analysis. Second, the current behavior of surveyed individuals is unlikely to be entirely attributable to the introduction of a vehicle sharing scheme. For example, changes in fuel prices are also likely to affect transport decisions.18
The vehicles available through car sharing schemes are not necessarily representative of the wider vehicle fleet. They are often smaller and are increasingly electric, and may therefore have lower environmental impacts relative to an “average” private vehicle. Some studies incorporate this effect via life cycle analysis. This approach is typically of limited scope, focusing mostly on the differential energy use and greenhouse gas emissions associated with the use phase of the vehicle life. Any differential environmental impacts associated with vehicle manufacturing and disposal are largely ignored (although see Doka and Ziegler, 2001 ([49])).
Table 4.3. Selected literature assessing the environmental impacts of car sharing
Author |
Methodology |
Average change in VKT |
Average change in mobility related GHG emissions |
Private cars replaced |
---|---|---|---|---|
Doka and Ziegler (2001[49]) |
LCA |
- |
-39% |
- |
Lane (2005[50]) |
Survey |
- |
- |
23 |
Millard-Ball et al. (2005[51]) |
? |
-37% |
- |
15 |
Ryder and Morin (2005[52]) |
Survey |
-28% to -48% |
-39% to -54% |
- |
Cervero et al. (2007[53]) |
Survey |
-33% |
- |
- |
MOMO (2010[54]) |
Meta-review |
- |
-8% to -36% |
4 to 14 |
Martin et al. (2011[55]) Martin and Shaheen (2011[56]) |
Survey |
- |
-15% |
9 to 13 |
Firnkorn and Muller (2011[57]) |
Survey |
- |
- |
11 |
Stasko et al. (2013[58]) |
Survey |
- |
- |
15 |
Martin and Shaheen (2016[59]) |
Survey |
-6% to -16% |
-4% to -18% |
7 to 11 |
Chen and Kockelman (2016[60]) |
Meta-review |
-27% to -67% |
-51% |
- |
Nijland and van Meerkerk (2017[61]) |
Survey |
-15% to -20% |
-13% to -18% |
- |
Note: Changes in average VKT and mobility related GHG emissions are for survey respondents only (i.e. members of car sharing schemes). Changes in average mobility related GHG emissions reflect differing combinations of: (i) the lower emissions intensity of car sharing vehicles, (ii) changed driving behavior resulting from access to a car sharing scheme, and (iii) associated shifts in transport modes.
Source: Various
Results from individual studies shown in Table 4.3 are not directly comparable. The boundary of each analysis varies, as do assumptions about key parameters. For example, in estimating the impact of car sharing on transport related GHG emissions, some studies restrict their analysis to the impact resulting purely from different car designs (e.g. Doka and Ziegler, 2001 ([49])), or purely from behavioral changes (e.g. Martin and Shaheen, 2011 ([56])). Further, the treatment of changes in transport modes resulting from the introduction of car sharing differs across studies. Some assessments account for emissions associated with shifts to other transport modes (e.g. Firnkorn and Muller, 2011 ([57])) whereas others ignore this effect on the basis that is likely to be small (e.g. Martin and Shaheen, 2011 ([56])). Finally, different types of car sharing are considered by different studies. Some assessments only consider one-way systems (e.g. Firnkorn and Muller, 2011 ([57])), others only consider roundtrip systems (e.g Millard-Ball et al. 2005 ([51])), and some (e.g. Nijland and van Meerkerk, 2017 ([61])) include both.
The environmental impact associated with the introduction of a car sharing scheme is consistent across the studies shown in Table 4.3. On average, members drive fewer kilometers, emit fewer GHG, and own fewer cars than they did previously. This aggregate outcome masks important variations in individual level behaviour. For example, various studies indicate that the majority of active car sharing members do not own a private vehicle. Around 58% of respondents in Martin, Shaheen and Lidicker (2011[55]) said that they joined car sharing because they didn’t have a car of their own. For this portion of the population, gaining access to a car sharing scheme probably serves to increase VKT (and probably also GHG emissions) as individuals shift away from other transport modes. The fact that overall VKT and GHG emissions fall appears to be, in large part, due to the behavior of a minority of car owning individuals. Survey data indicates that this portion of the population responds to the introduction of a car sharing scheme by reducing vehicle ownership and substituting shared mobility or other transport modes for private car ownership. Research by Martin and Shaheen (2011[56]) finds that the emissions reductions associated with this portion of the population more than offset the emissions increases associated with individuals without previous vehicle access (Figure 4.4).
Figure 4.4. Changes in mobility related to GHG emissions due to the introduction of a car sharing scheme

Note: Cumulative annual change in emissions represents the annual change in emissions made by each car sharing member multiplied by the number of individuals that make the same response.
Source: Martin and Shaheen (2011[56]).
The studies shown in Table 4.3 also indicate that having access to a car sharing scheme can lead to reduced rates of car ownership. This has at least two potential impacts on the environmental pressures associated with mobility. First, a smaller vehicle stock reduces demand for parking space. This can have positive environmental implications in urban areas where the sealing of bare ground can lower aquifer recharge rates and reduce resilience to storm events. Second, reduced vehicle demand may lead to lower rates or virgin resource extraction further upstream. This idea is controversial, it relies heavily on the assumption that demand reductions resulting from reduced private car ownership are not offset by any reduction in average vehicle life (i.e., due to high utilisation rates of shared vehicles). The net effect of car sharing on overall vehicle demand and resource extraction is uncertain without data on the average life of shared vehicles.
4.4. How economic feedbacks influence environmental outcomes
The LCA literature provides a valuable insight into the environmental footprint of circular products and modes of production relative to traditional equivalents. However, the actual environmental impact of circular business models will also depend on the output growth they can achieve, the extent to which this output displaces that associated with traditional production modes, and on the indirect effects that this has on the composition of the broader economy (Zink and Geyer, 2017[62]). Furthermore, the emergence of global value chains means that these broader effects are unlikely to be restricted to a particular jurisdiction, but will influence economic and environmental outcomes across borders. In sum, focussing solely on LCA studies alone may result in an incomplete view of the environmental potential of circular business models.
There is a growing literature of accounting studies that deal with this issue by combining results from LCA studies with assumptions about the market share that a circular business model could capture in the future. For example, TNO (2013[63]) find that increased recycling in the Dutch consumer electronics and food sectors could lead to emissions reductions of 747 000 tonnes and 150 000 tonnes of CO2 per year respectively. Similarly, the Ellen Macarthur Foundation (2013[64]) find that higher reuse and remanufacturing rates for mobile phones in the EU could eradicate 1 300 000 tonnes of CO2e annually. While these accounting approaches do give some indication of the overall environmental potential of a circular economy transition, they tend to ignore the economic realities that such a transition is conditional upon. In particular, it is often assumed that circular business models will scale up regardless of their underlying profitability, and that the new output will displace that associated with traditional production modes on a one-to-one basis. These assumptions, along with the fact that indirect economic effects are largely ignored, mean that the likelihood of achieving the stated environmental benefits is probably small.
Addressing the various market failures, policy misalignments, status quo biases, and other barriers (see Chapter 3) that hinder the profitability of circular business models will tend to stimulate future scale up. The environmental implications of this scale up will emerge through two main channels. Direct environmental impacts will result from the expansion of the new business model in a particular sector. Indirect environmental impacts will result from changes in the activity levels of other sectors of the economy in response to general equilibrium price effects. Establishing the overall environmental impact associated with the emergence of a circular business model requires consideration of both (Figure 4.5).
Figure 4.5. Direct and indirect impacts of addressing the barriers to circular business model adoption

4.4.1. Direct environmental impacts of circular business model scale up
The selected LCA literature presented in this chapter indicates that the environmental footprint of circular products and circular modes of production is typically significantly smaller than for their traditional equivalents. By closing and slowing resource loops, and by narrowing resource flows, these business models reduce the extraction, transport, processing, and disposal of new resources, and the environmental pressures associated with these activities. For example, the existing LCAs focusing on remanufacturing find that it can save 80 to 90% of the energy that is required to manufacture certain types of new products. The direct environmental effect associated with the emergence of a circular business model will depend both on this difference in footprints, as well as on the extent to which traditional production is displaced.
As discussed, most LCA and accounting assessments of the environmental impact of circular business models assume that output from an emerging circular business model will displace that from a traditional business model on a one-to-one basis. This may be an overly optimistic assumption from an economic viewpoint, especially if the respective products are not perfect substitutes. For example, in the context of metal recycling, it is well established that secondary metals are less substitutable for their primary equivalents in certain high performance applications (Koffler et al., 2013[65]). Similarly, for sharing models, it is unlikely that short term lodging á la Airbnb is a perfect substitute for hotel rooms; many business travelers continue to prefer the convenience of the latter. Limited substitutability may mean that some circular products will be produced in addition, rather than instead of, traditional products (Zink and Geyer, 2017[62]).19 If true, this implies that the overall direct environmental benefit of the growth of circular business models may be smaller than predicted.20
4.4.2. Indirect environmental impacts of circular business model scale up
The emergence of a circular business model in a particular sector cannot be considered in isolation. Increased activity will typically trigger changes in relative prices, thereby leading to spill-over and feedback effects elsewhere in the economy (Hertwich, 2005[66]). In terms of their environmental impact, these general equilibrium effects can act to “take back” the first order environmental gains associated with a particular business model.
Sorrell and Dimitripoulos (2008[67]) distinguish three types of indirect or general equilibrium economic effects:
Direct rebound effects occur when lower product prices, perhaps resulting from the emergence of a more resource efficient technology, mode of production, or product, lead to additional consumption. This often involves consumers. For example, although the fuel efficiency of vehicle engines has improved markedly over recent decades, the average fuel consumption per kilometre travelled has barely changed; consumers have opted to use the savings to buy larger and heavier vehicles. Direct rebound effects also involve firms. For example, increased penetration of heavy machinery remanufacturing will tend to reduce upstream resource extraction, but the resulting fall in prices may trigger additional demand for these materials from other production sectors.
Indirect effects occur when consumers allocate monetary savings, perhaps resulting from the emergence of a more resource efficient technology, mode of production, or product, to additional consumption of other goods and services (with an associated material and environmental footprint). For example, consumers may decide to allocate additional disposable income from sharing power tools (rather than buying them) to additional overseas travel.
Economy wide effects occur when the emergence of a more resource efficient technology, mode of production, or product triggers changes in the composition or size of the overall economy. For example, a number of modelling assessments indicate that resource efficiency and circular economy policies could generate significant economic growth (McCarthy, Dellink and Bibas, 2018[68]). This additional output will clearly have an associated material and environmental footprint.
The key message here is that gaining a better understanding of the overall environmental impact of circular economy business models requires considering the full range of dynamic and indirect economic feedbacks. Research in other fields has highlighted the potential importance of these mechanisms. Empirical assessments of the direct rebound effect in the context of energy efficiency improvements find that they are typically in the order of 15 to 30%, and occasionally as high as 50 to 60% (Hertwich, 2005[66]). Modelling assessments have also been undertaken, and often suggest that the size of the total rebound effect can exceed 50% (Dimitropoulos, 2007[69]; Koesler, Swales and Turner, 2016[70]; Parrado, Eni and Mattei, 2017[71]). The magnitude of these effects is quite striking given that they have been largely overlooked in assessments of the environmental impacts of circular business models. As stated in a recent review, “proponents of the circular economy have tended to look at the world purely as an engineering system and have overlooked the economic part of the circular economy” (Zink and Geyer, 2017[62]).
4.5. Conclusions
This chapter has highlighted the complexity involved in assessing the environmental impact of circular business models. Not only are there a wide range of business models involved, but there are also a variety of different approaches available to estimate their impacts. In addition, the impacts associated with each business model often emerge at different parts of the product lifecycle.21 Based on the material presented in this chapter, it is possible to draw four main conclusions.
First, results from the LCA literature indicate that the environmental footprint of the output from circular modes of production can be significantly smaller than that associated with traditional modes. As such, the emergence of circular business models, to the extent that this results in the displacement of traditional forms of production, is likely to have a positive direct impact on environmental outcomes. By closing and slowing resource loops, and by narrowing resource flows, these business models will lead to less extraction, transport, processing, and disposal of virgin resources, and thereby reduce the environmental pressures associated with these activities.22
Second, the LCA and accounting studies presented here do not account for indirect economic effects associated with the emergence of circular business models. This emergence, conditional on improved competitiveness of circular modes of production, will place downward pressure on prices and are likely to lead to a range of rebound effects. Households may direct new disposable income towards additional consumption, and this will have an associated environmental footprint. Further, reduced demand for primary materials, and the lower prices that this stimulates, may encourage manufacturing firms to use relatively more such inputs. In sum, indirect economic feedbacks are important, and potentially could at least partially, offset the direct environmental benefits associated with the emergence of a circular business models.23
Third, comparing the relative environmental potential of different circular business models is difficult. Making such an assessment is difficult given that a particular business model typically operates in multiple sectors, and has implications across a range of environmental impact categories, often in multiple political jurisdictions. Thus, while it might be possible to compare the environmental footprint of recycling or repairing an old vehicle, blanket statements about the relative desirability of resource recovery vs product life extension business models (for example) is fraught. Although the circularity ladder approach provides some insight on this issue, it is unclear to what extent the waste hierarchy ranking of waste management activities reflects environmental impacts higher in the product lifecycle. For example, some LCA studies suggest that reuse may not necessarily be preferable to recycling when the products involved are long lived, have an energy intensive use phase, and are experiencing rapid efficiency improvements.
Fourth, with respect to the product life extension, sharing, and product service system business models, there is a tension between changes in product lifetimes and the diffusion of relatively efficient new product designs. Put differently, this tension reflects a tradeoff between environmental impacts associated with different parts of the product lifecycle. Products that last longer will tend to reduce the extraction and processing of virgin resources (and the associated environmental impacts of these activities), but may also hinder the diffusion of relatively efficient new products. Product category is critical here; this issue is again of most concern for products that are long lived, have an energy intensive use phase, and are experiencing rapid efficiency improvements.
Therefore, while the lifecycle environmental impacts of circular goods and services are mostly significantly smaller than those of linear ones, uncertainty about rebound effects, product innovation and other factors, tend to muddy the picture and prevent from drawing more general conclusions across business models, sectors and product groups. The environmental outcomes of circular business models will therefore need to be carefully assessed on a case by case basis.
References
[102] ABREE (2016), Resources and Energy Statistics, https://industry.gov.au/Office-of-the-Chief-Economist/Publications/Pages/Resources-and-energy-statistics.aspx (accessed on 18 May 2018).
[75] Achterberg, E., J. Hinfelaar and N. Bocken (2016), The Value Hill Business Model Tool: identifying gaps and opportunities in a circular network, http://www.scienceandtheenergychallenge.nl/sites/default/files/multimedia/organization/sec/2016-06-16_NWO_Sc4CE/NWO%20Sc4CE%20-%20Workshop%20Business%20Models%20-%20Paper%20on%20Circular%20Business%20Models.pdf (accessed on 13 September 2018).
[39] ADEME (2016), Potentiels d'expansion de la consommation collaborative pour réduire les impacts environnemenaux, https://www.ademe.fr/potentiels-dexpansion-consommation-collaborative-reduire-impacts-environnementaux (accessed on 14 September 2018).
[109] Agrawal, V., A. Atasu and K. van Ittersum (2015), “Remanufacturing, Third-Party Competition, and Consumers' Perceived Value of New Products”, Management Science, Vol. 61/1, pp. 60-72, http://dx.doi.org/10.1287/mnsc.2014.2099.
[44] Agrawal, V. et al. (2012), “Is Leasing Greener Than Selling?”, Management Science, Vol. 58/3, pp. 523-533, http://dx.doi.org/10.1287/mnsc.1110.1428.
[112] AirBnb (2018), Night Limits: Frequently Asked Questions, https://www.airbnb.com/help/article/1628/night-limits--frequently-asked-questions (accessed on 13 September 2018).
[105] AmCham (2017), China (Ningbo) Remanufacturing Industry International Cooperation Forum, https://www.amcham-shanghai.org/en/article/china-ningbo-remanufacturing-industry-international-cooperation-forum-0?lang=en (accessed on 13 September 2018).
[132] Ashman (2017), The Internet of Things: paving the way for renewable energy? – Capgemini Worldwide, https://www.capgemini.com/2017/08/the-internet-of-things-paving-the-way-for-renewable-energy/ (accessed on 13 September 2018).
[16] Astrup, T. et al. (2015), “Life cycle assessment of thermal Waste-to-Energy technologies: Review and recommendations”, Waste Management, Vol. 37, pp. 104-115, http://dx.doi.org/10.1016/j.wasman.2014.06.011.
[122] BBC (2017), Beijing bans new bikes as sharing schemes cause chaos - BBC News, https://www.bbc.com/news/business-41197341 (accessed on 13 September 2018).
[6] Bernardo, C., C. Simões and L. Pinto (2016), “Environmental and economic life cycle analysis of plastic waste management options. A review Cost management for waste to energy systems using life cycle costing approach: A case study from Environmental and Economic Life Cycle Analysis of Plastic Waste Management Options. A Review”, China Journal of Renewable and Sustainable Energy, Vol. 10/123, pp. 140001-140002, http://dx.doi.org/10.1063/1.4958429.
[4] BIR (2008), Report on the Environmental Benefits of Recycling, http://www.mgg-recycling.com/wp-content/uploads/2013/06/BIR_CO2_report.pdf (accessed on 14 September 2018).
[28] Biswas, W. and M. Rosano (2011), “A life cycle greenhouse gas assessment of remanufactured refrigeration and air conditioning compressors”, International Journal of Sustainable Manufacturing, Vol. 2/2/3, p. 222, http://dx.doi.org/10.1504/IJSM.2011.042153.
[85] Bocken, N. et al. (2016), “Product design and business model strategies for a circular economy”, Journal of Industrial and Production Engineering, Vol. 33/5, pp. 308-320, http://dx.doi.org/10.1080/21681015.2016.1172124.
[35] Böcker, L. and T. Meelen (2017), “Sharing for people, planet or profit? Analysing motivations for intended sharing economy participation”, Environmental Innovation and Societal Transitions, Vol. 23, pp. 28-39, http://dx.doi.org/10.1016/J.EIST.2016.09.004.
[95] CDP (2016), Embedding a carbon price into business strategy, https://b8f65cb373b1b7b15feb-c70d8ead6ced550b4d987d7c03fcdd1d.ssl.cf3.rackcdn.com/cms/reports/documents/000/001/132/original/CDP_Carbon_Price_report_2016.pdf?1474899276 (accessed on 13 September 2018).
[53] Cervero, R., A. Golub and B. Nee (2007), “City CarShare: longer-term travel demand and car ownership impacts”, Transportation Research Record: Journal of the Transportation Research Board 1992, pp. 70-80.
[60] Chen, T. and K. Kockelman (2016), “Carsharing’s life-cycle impacts on energy use and greenhouse gas emissions”, Transportation Research Part D: Transport and Environment, Vol. 47, pp. 276-284, http://dx.doi.org/10.1016/j.trd.2016.05.012.
[43] Cleantech (2014), Airbnb says it's better for the environment than hotels, https://venturebeat.com/2014/07/31/airbnb-says-its-better-for-the-environment-than-hotels/ (accessed on 14 September 2018).
[14] Cleary, J. (2009), “Life cycle assessments of municipal solid waste management systems: A comparative analysis of selected peer-reviewed literature”, Environment International, Vol. 35/8, pp. 1256-1266, http://dx.doi.org/10.1016/J.ENVINT.2009.07.009.
[96] Climate Home (2016), Exxon, Shell, Total, Statoil renew clean energy drive, http://www.climatechangenews.com/2016/05/23/exxon-shell-total-and-statoil-make-clean-energy-plays/ (accessed on 13 September 2018).
[83] Cooper, T. (2004), “Inadequate Life?Evidence of Consumer Attitudes to Product Obsolescence”, Journal of Consumer Policy, Vol. 27/4, pp. 421-449, http://dx.doi.org/10.1007/s10603-004-2284-6.
[93] COWI (2008), Promoting Innovative Business Models with Environmental Benefits, http://www.cowi.com (accessed on 13 September 2018).
[2] De Groene, Z. and Ethica (2015), Boosting Circular Design for a Circular Economy, http://degroenezaak.com/wp-content/uploads/2015/03/Boosting_Circular_Design_for_a_Circular_Economy_FINAL.pdf (accessed on 14 September 2018).
[20] DEFRA (2011), “Longer Product Lifetimes: Life Cycle Impact of Nine Products”, http://sciencesearch.defra.gov.uk/Default.aspx?Menu=Menu&Module=More&Location=None&Completed=0&ProjectID=17047 (accessed on 14 September 2018).
[69] Dimitropoulos, J. (2007), “Energy productivity improvements and the rebound effect: An overview of the state of knowledge”, Energy Policy, Vol. 35/12, pp. 6354-6363, http://dx.doi.org/10.1016/J.ENPOL.2007.07.028.
[49] Doka, G. and S. Ziegler (2001), Complete Life Cycle Assessment for Vehicle Models of the Mobility CarSharing Fleet Switzerland, http://www.doka.ch/DokaMobilitySTRCproc01.pdf (accessed on 17 September 2018).
[12] DSEWPC (2012), The Australian Recycling Sector Report, https://www.environment.gov.au/system/files/resources/dc87fd71-6bcb-4135-b916-71dd349fc0b8/files/australian-recycling-sector.pdf (accessed on 14 September 2018).
[7] EEA (2006), “Paper and cardboard — recovery or disposal? Review of life cycle assessment and cost-benefit analysis on the recovery and disposal of paper and cardboard”, http://www.pedz.uni-mannheim.de/daten/edz-bn/eua/06/technical_rep_5_2006.pdf (accessed on 14 September 2018).
[18] Ekvall, T. et al. (2007), Limitations and amendments in life-cycle assessment on waste management, https://www.researchgate.net/publication/255823714_Limitations_and_amendments_in_life-cycle_assessment_on_waste_management (accessed on 14 September 2018).
[64] Ellen Macarthur Foundation (2013), Towards the Circular Economy, https://www.ellenmacarthurfoundation.org/assets/downloads/publications/Ellen-MacArthur-Foundation-Towards-the-Circular-Economy-vol.1.pdf (accessed on 17 September 2018).
[99] Ellen McArthur Foundation (2018), Eleven companies take major step towards a New Plastics Economy, https://www.ellenmacarthurfoundation.org/news/11-companies-take-major-step-towards-a-new-plastics-economy (accessed on 13 September 2018).
[89] Ellen McArthur Foundation (2015), GROWTH WITHIN: A CIRCULAR ECONOMY VISION FOR A COMPETITIVE EUROPE, https://www.ellenmacarthurfoundation.org/assets/downloads/publications/EllenMacArthurFoundation_Growth-Within_July15.pdf (accessed on 13 September 2018).
[31] ERN (2015), Remanufacturing Market Study, European Remanufacturing Network, http://www.remanufacturing.eu/assets/pdfs/remanufacturing-market-study.pdf.
[97] European Commission (2018), Plastic Waste: a European strategy to protect the planet, defend our citizens and empower our industries, http://europa.eu/rapid/press-release_IP-18-5_en.htm (accessed on 18 January 2018).
[37] European Commission (2016), Scoping the Sharing Economy: Origins, Definitions, Impact and Regulatory Issues, https://ec.europa.eu/jrc (accessed on 14 September 2018).
[128] European Commission (2016), Study on socioeconomic impacts of increased reparability of increased reparability - EU Law and Publications, https://publications.europa.eu/en/publication-detail/-/publication/c6865b39-2628-11e6-86d0-01aa75ed71a1 (accessed on 13 September 2018).
[123] Eurostat (2018), Eurostat - Trade in recyclable raw materials, http://ec.europa.eu/eurostat/tgm/table.do?tab=table&plugin=1&language=en&pcode=cei_srm020 (accessed on 28 March 2018).
[57] Firnkorn, J. and M. Müller (2011), “What will be the environmental effects of new free-floating car-sharing systems? The case of car2go in Ulm”, Ecological Economics, Vol. 70/8, pp. 1519-1528, http://dx.doi.org/10.1016/J.ECOLECON.2011.03.014.
[33] Frenken, K. and J. Schor (2017), “Putting the sharing economy into perspective”, Environmental Innovation and Societal Transitions, Vol. 23, pp. 3-10, http://dx.doi.org/10.1016/J.EIST.2017.01.003.
[80] Gaillochet, C. and P. Chalmin (2009), From waste to ressources : world waste survey 2009, https://www.researchgate.net/publication/41222409_From_waste_to_ressources_world_waste_survey_2009 (accessed on 13 September 2018).
[32] Gao, W. et al. (2017), “Investigation on the Comparative Life Cycle Assessment between Newly Manufacturing and Remanufacturing Turbochargers”, Procedia CIRP, Vol. 61, pp. 750-755, http://dx.doi.org/10.1016/j.procir.2016.11.214.
[124] Geyer, R., J. Jambeck and K. Law (2017), “Production, use, and fate of all plastics ever made”, Science Advances, Vol. 3/7, p. e1700782, http://dx.doi.org/10.1126/sciadv.1700782.
[26] Gutowski, T. et al. (2011), “Remanufacturing and Energy Savings”, Environmental Science & Technology, Vol. 45/10, pp. 4540-4547, http://dx.doi.org/10.1021/es102598b.
[66] Hertwich, E. (2005), Consumption and the Rebound Effect An Industrial Ecology Perspective, http://mitpress.mit.edu/jie (accessed on 17 September 2018).
[5] HPRC (2015), Environmental Impacts of Recycling Compared to Other Waste Disposal Methods, https://docs.wixstatic.com/ugd/49d7a0_6bb3ebb481ec49ceafef92f0b0ba010d.pdf (accessed on 28 March 2018).
[36] IDDRI (2014), The sharing economy: make it sustainable, http://www.iddri.org (accessed on 13 September 2018).
[76] IMSA (2015), Circular business models: an introduction to IMSA's circular business model scan, https://groenomstilling.erhvervsstyrelsen.dk/sites/default/files/media/imsa_circular_business_models_-_april_2015_-_part_1.pdf (accessed on 13 September 2018).
[48] Intlekofer, K., B. Bras and M. Ferguson (2010), “Energy Implications of Product Leasing”, http://dx.doi.org/10.1021/es9036836.
[131] Jagtap, S. (2017), IoT Concepts for Improving the Resource Efficiency of Food Supply Chains, http://www.manufacturingfoodfutures.com/documents/utilization-of-internet-of-things-concepts-to-improve-resource-efficiency-of-food-supply-chains-sandeep-jagtap.pdf (accessed on 13 September 2018).
[25] Kara, H. (2010), Comparative Carbon Footprint Analysis of New and Remanufactured Inkjet Cartridges, Centre for Remanufacturing and Reuse (CPR), http://ww.ebpgroup.com/wp-content/uploads/pdf/CCFreport.pdf (accessed on 19 October 2018).
[21] Kerr, W. and C. Ryan (2001), “Eco-efficiency gains from remanufacturing”, Journal of Cleaner Production, Vol. 9/1, pp. 75-81, http://dx.doi.org/10.1016/S0959-6526(00)00032-9.
[19] Kinnaman, T., T. Shinkuma and M. Yamamoto (2014), “The socially optimal recycling rate: Evidence from Japan”, Journal of Environmental Economics and Management, Vol. 68, pp. 54-70, http://dx.doi.org/10.1016/j.jeem.2014.01.004.
[70] Koesler, S., K. Swales and K. Turner (2016), “International spillover and rebound effects from increased energy efficiency in Germany”, Energy Economics, Vol. 54, pp. 444-452, http://dx.doi.org/10.1016/J.ENECO.2015.12.011.
[65] Koffler, C. et al. (2013), “Tackling the Downcycling Issue—A Revised Approach to Value-Corrected Substitution in Life Cycle Assessment of Aluminum”, Sustainability, Vol. 5/11, pp. 4546-4560, http://dx.doi.org/10.3390/su5114546.
[45] Kuo, T. (2011), “Simulation of purchase or rental decision-making based on product service system”, The International Journal of Advanced Manufacturing Technology, Vol. 52/9-12, pp. 1239-1249, http://dx.doi.org/10.1007/s00170-010-2768-2.
[78] Lacy, P. and J. Rutqvist (2015), Waste to wealth : the circular economy advantage.
[50] Lane, C. (2005), “PhillyCarShare: First-Year Social and Mobility Impacts of Carsharing in Philadelphia, Pennsylvania”, Transportation Research Record: Journal of the Transportation Research Board, Vol. 1927, pp. 158-166, http://dx.doi.org/10.3141/1927-18.
[13] Laurent, A. et al. (2014), “Review of LCA studies of solid waste management systems – Part I: Lessons learned and perspectives”, Waste Management, Vol. 34/3, pp. 573-588, http://dx.doi.org/10.1016/J.WASMAN.2013.10.045.
[108] Lavery, G. et al. (2013), The Next Manufacturing Revolution, http://www.2degreesnetwork.com (accessed on 13 September 2018).
[88] Le Moigne, R. and L. Georgeault (2016), “Remanufacturing: Une Formidable Opportunité Pour La France Industrielle de Demain”, https://www.wmaker.net/eco-circulaire/attachment/656994/ (accessed on 13 September 2018).
[77] Lewandowski, M. (2016), “Designing the Business Models for Circular Economy—Towards the Conceptual Framework”, Sustainability, Vol. 8/1, p. 43, http://dx.doi.org/10.3390/su8010043.
[29] Liu, Z. et al. (2014), “Life Cycle Assessment-based Comparative Evaluation of Originally Manufactured and Remanufactured Diesel Engines”, Journal of Industrial Ecology, Vol. 18/4, pp. 567-576, http://dx.doi.org/10.1111/jiec.12137.
[82] Long, X. et al. (2017), “Strategy Analysis of Recycling and Remanufacturing by Remanufacturers in Closed-Loop Supply Chain”, Sustainability, Vol. 9/10, pp. 1-29, https://ideas.repec.org/a/gam/jsusta/v9y2017i10p1818-d114402.html (accessed on 13 September 2018).
[56] Martin, E. and S. Shaheen (2011), “Greenhouse Gas Emission Impacts of Carsharing in North America”, IEEE Transactions on Intelligent Transportation Systems, Vol. 12/4, pp. 1074-1086, http://dx.doi.org/10.1109/TITS.2011.2158539.
[55] Martin, E., S. Shaheen and J. Lidicker (2011), “Impact of Carsharing on Household Vehicle Holdings”, http://dx.doi.org/10.3141/2143-19.
[68] McCarthy, A., R. Dellink and R. Bibas (2018), “The Macroeconomics of the Circular Economy Transition: A Critical Review of Modelling Approaches”, OECD Environment Working Papers, No. 130, OECD Publishing, Paris, http://dx.doi.org/10.1787/af983f9a-en.
[51] Millard-Ball, A. et al. (2005), Car-Sharing: Where and How It Succeeds, https://www.nap.edu/download/13559 (accessed on 17 September 2018).
[54] MOMO (2010), The State of European Car-Sharing, http://www.eltis.org/sites/default/files/tool/the_state_of_carsharing_europe.pdf (accessed on 18 October 2018).
[9] Morris, J., H. Matthews and C. Morawski (2012), “Review and meta-analysis of 82 studies on end-of-life management methods for source separated organics”, Waste Management, Vol. 33/3, pp. 545-551, http://dx.doi.org/10.1016/J.WASMAN.2012.08.004.
[120] Navigant Research (2015), Carsharing Programs, https://www.navigantresearch.com/reports/carsharing-programs (accessed on 13 September 2018).
[8] NCASI (2012), Summary of the Literature on the Treatment of Paper and Paper Packaging Products, https://twosidesna.org/US/NCASI-Summary-of-the-Literature-on-the-Treatment-of-Paper-and-Paper-Packaging-Products-Recycling-in-Life-Cycle-Assessment-2011/ (accessed on 14 September 2018).
[24] Neto, J. and J. Bloemhof-Ruwaard (2009), The Environmental Gains of Remanufacturing: Evidence from the Computer and Mobile Industry, ERIM Report Series Reference No. ERS-2009-024-LIS, https://ssrn.com/abstract=1410467 (accessed on 19 October 2018).
[61] Nijland, H. and J. Van Meerkerk (2017), “Mobility and environmental impacts of car sharing in the Netherlands”, Environmental Innovation and Societal Transitions, Vol. 23, pp. 84-91, http://dx.doi.org/10.1016/j.eist.2017.02.001.
[41] Nordic Council of Ministers, T. (2017), Environmental impacts and potential of the sharing economy, TemaNord, Nordic Council of Ministers, Copenhagen K, http://dx.doi.org/10.6027/TN2017-554.
[17] NSW EPA (2010), Environmental benefits of recycling, https://www.epa.nsw.gov.au/publications/recyclereuse/100058-benefits-of-recycling (accessed on 18 September 2018).
[116] OECD (2018), ONLINE PLATFORMS: A PRACTICAL APPROACH TO THEIR ECONOMIC AND SOCIAL IMPACTS, https://one.oecd.org/document/DSTI/CDEP(2018)5/en/pdf (accessed on 13 September 2018).
[47] OECD (2017), Economic Features of Chemical Leasing, https://one.oecd.org/document/ENV/JM/MONO(2017)10/en/pdf (accessed on 13 September 2018).
[103] OECD (2017), MAPPING SUPPORT FOR PRIMARY AND SECONDARY METAL PRODUCTION, https://one.oecd.org/document/ENV/EPOC/WPRPW(2016)2/FINAL/en/pdf (accessed on 13 September 2018).
[115] OECD (2017), THE POSSIBLE ROLE OF DIGITAL PLATFORMS IN THE COLLECTION OF VAT/GST ON ONLINE SALES, https://one.oecd.org/document/CTPA/CFA/WP9(2017)6/en/pdf (accessed on 13 September 2018).
[117] OECD (2016), PROTECTING CONSUMERS IN PEER PLATFORM MARKETS: EXPLORING THE ISSUES, https://one.oecd.org/document/DSTI/CP(2015)4/REV2/en/pdf (accessed on 13 September 2018).
[72] OECD (2012), OECD Environmental Outlook to 2050: The Consequences of Inaction, OECD Publishing, Paris, http://dx.doi.org/10.1787/9789264122246-en.
[119] OICA (2015), Vehicles in use, http://www.oica.net/category/vehicles-in-use/ (accessed on 13 September 2018).
[74] Osterwalder, A., Y. Pigneur and C. Tucci (2010), Clarifying Business Models: Origins, Present, and Future of the Concept, https://www.researchgate.net/publication/37426694_Clarifying_Business_Models_Origins_Present_and_Future_of_the_Concept (accessed on 13 September 2018).
[87] Parker, D. et al. (2015), Remanufacturing Market Study, http://www.remanufacturing.eu/assets/pdfs/remanufacturing-market-study.pdf (accessed on 13 September 2018).
[71] Parrado, R., F. Eni and E. Mattei (2017), The Economy-Wide Rebound Effect and Climate Policy Effectiveness in a Multiregional General Equilibrium Framework, https://www.gtap.agecon.purdue.edu/resources/download/6853.pdf (accessed on 17 September 2018).
[34] PWC (2015), The sharing economy, https://www.pwc.com/us/en/services/consulting/library/consumer-intelligence-series/sharing-economy.html (accessed on 14 September 2018).
[111] Recode (2017), Airbnb is on track to rack up more than 100 million stays this year — and that’s only the beginning of its threat to the hotel industry - Recode, https://www.recode.net/2017/7/19/15949782/airbnb-100-million-stays-2017-threat-business-hotel-industry (accessed on 28 August 2018).
[52] Ryden, C. and E. Morin (2005), “Mobility services for urban sustainability: Environmental assessment”, Moses Report WP6, Trivector Traffic AB, Stockholm, Sweden.
[113] San Francisco Business Portal (2018), Short-Term Rental, https://businessportal.sfgov.org/start/starter-kits/short-term-rental (accessed on 13 September 2018).
[1] SEI (2005), Rethinking the Waste Hierarchy, http://www.imv.dk (accessed on 14 September 2018).
[118] Shaheen, S. and A. Cohen (2016), Innovative Mobility Carsharing Outlook, https://cloudfront.escholarship.org/dist/prd/content/qt49j961wb/qt49j961wb.pdf?t=pa6fa3&v=lg (accessed on 13 September 2018).
[59] Shaheen, S. and E. Martin (2016), Impacts of car2go on Vehicle Ownership, Modal Shift, Vehicle Miles Traveled, and Greenhouse Gas Emissions, Transportation Sustainability Research Center, UC Berkeley, http://www.tsrc.berkeley.edu (accessed on 18 October 2018).
[38] Sierra Club (2017), Is the Sharing Economy Truly Green?, https://www.sierraclub.org/sierra/2016-2-march-april/green-life/sharing-economy-truly-green (accessed on 14 September 2018).
[22] Smith, V. and G. Keoleian (2004), “The Value of Remanufactured Engines: Life-Cycle Environmental and Economic Perspectives”, Journal of Industrial Ecology, Vol. 8/1-2, pp. 193-221, http://dx.doi.org/10.1162/1088198041269463.
[67] Sorrell, S. and J. Dimitropoulos (2008), “The rebound effect: Microeconomic definitions, limitations and extensions”, Ecological Economics, Vol. 65/3, pp. 636-649, http://dx.doi.org/10.1016/J.ECOLECON.2007.08.013.
[86] Spelman, C. and B. Sheerman (2014), Triple Win: The Social, Economic, and Environmental Case for Remanufacturing, http://www.policyconnect.org.uk/apmg (accessed on 13 September 2018).
[58] Stasko, T., A. Buck and H. Oliver Gao (2013), “Carsharing in a university setting: Impacts on vehicle ownership, parking demand, and mobility in Ithaca, NY”, Transport Policy, Vol. 30, pp. 262-268, http://dx.doi.org/10.1016/j.tranpol.2013.09.018.
[91] Statista (2017), Airbnb - Statistics & Facts, https://www.statista.com/topics/2273/airbnb/ (accessed on 13 September 2018).
[23] Steinhilper, R. (1998), Remanufacturing: The Ultimate Form of Recycling Fraunhofer IRB Verlag, Fraunhofer IRB Verlag, http://www.elitecreativesolutions.com/files/steinhilper_part1.pdf (accessed on 19 October 2018).
[90] STR (2017), Airbnb & Hotel Performance, http://www.str.com/Media/Default/Research/STR_AirbnbHotelPerformance.pdf (accessed on 13 September 2018).
[130] Stuart, E., C. Goldman and D. Gilligan (2014), The U.S. ESCO Industry: Recent Trends, Current Size and Remaining Market Potential, http://aceee.org/files/proceedings/2014/data/papers/3-319.pdf (accessed on 13 September 2018).
[81] Taranic, I., A. Behrens and C. Topi (2016), Understanding the Circular Economy in Europe, from Resource Efficiency to Sharing Platforms: The CEPS Framework, http://www.ceps.eu (accessed on 13 September 2018).
[126] Tech Crunch (2015), Upgrades Fueling Second Hand Smartphone Sales Boom, Says Gartner | TechCrunch, https://techcrunch.com/2015/02/18/reuse-dont-recycle/?guccounter=1 (accessed on 13 September 2018).
[129] The Guardian (2017), 'Screen fatigue' sees UK ebook sales plunge 17% as readers return to print | Books | The Guardian, https://www.theguardian.com/books/2017/apr/27/screen-fatigue-sees-uk-ebook-sales-plunge-17-as-readers-return-to-print (accessed on 13 September 2018).
[114] The Herald (2017), Major blow for Airbnb users: New law to restrict New York City apartment rentals | Newcastle Herald, https://www.theherald.com.au/story/4444330/major-blow-for-airbnb-users-new-law-to-restrict-new-york-city-apartment-rentals/?cs=34 (accessed on 13 September 2018).
[79] Thompson, A. et al. (2010), Benefits of a Product Service System Approach for Long-life Products: The Case of Light Tubes, http://www.ep.liu.se/ecp/077/011/ecp10077011.pdf (accessed on 13 September 2018).
[63] TNO (2013), Opportunities for a circular economy in the Netherlands (translated from: Kansen voor een circulaire economie in Nederland), https://www.rijksoverheid.nl/documenten/rapporten/2013/06/20/tno-rapport-kansen-voor-de-circulaire-economie-in-nederland.
[110] Travel Weekly (2016), Airbnb vs. the hotel industry, https://www.travelweekly.com/Danny-King/Airbnb-vs-hotel-industry (accessed on 13 September 2018).
[127] Trend Force (2017), Global Smartphone Production Volume Totaled 1.36 Billion Units; Samsung Held On as Leader While OPPO and Vivo Burst into Global Top Five, https://press.trendforce.com/node/view/2741.html (accessed on 13 September 2018).
[40] Trinomics (2017), What is the environmental impact of the sharing economy?, http://trinomics.eu/sharing-economy/ (accessed on 14 September 2018).
[46] Tukker, A. (2015), “Product services for a resource-efficient and circular economy – a review”, Journal of Cleaner Production, Vol. 97, pp. 76-91, http://dx.doi.org/10.1016/J.JCLEPRO.2013.11.049.
[92] Tukker, A. (2004), “Eight types of product–service system: eight ways to sustainability? Experiences from SusProNet”, Business Strategy and the Environment, Vol. 13/4, pp. 246-260, http://dx.doi.org/10.1002/bse.414.
[104] UN Combrade (2018), UN Comtrade | International Trade Statistics Database, https://comtrade.un.org/ (accessed on 05 June 2018).
[73] UNEP (2017), “RESOURCE EFFICIENCY: POTENTIAL AND ECONOMIC IMPLICATIONS”, http://www.resourcepanel.org/sites/default/files/documents/document/media/resource_efficiency_report_march_2017_web_res.pdf (accessed on 23 April 2018).
[100] UNEP (2013), Metal Recycling – Opportunitites, Limits, Infrastructure (Full report), United Nations Environment Programme, http://www.resourcepanel.org/sites/default/files/documents/document/media/e-book_metals_report2b_recyclingopportunities_130919.pdf (accessed on 18 May 2018).
[94] US EPA (2017), “U.S. EPA Sustainable Materials Management Program Strategic Plan”, https://www.epa.gov/sites/production/files/2016-03/documents/smm_strategic_plan_october_2015.pdf (accessed on 23 April 2018).
[106] US ITC (2012), Remanufactured Goods: An Overview of the U.S. and Global Industries, Markets, and Trade, http://www.usitc.gov (accessed on 13 September 2018).
[98] USGS (2016), USGS Minerals Information: Commodity Statistics and Information, https://minerals.usgs.gov/minerals/pubs/commodity/ (accessed on 18 May 2018).
[11] Valerio, F. (2010), Environmental Impacts of Post-Consumer Material Managements: recycling, biological treatments, incineration, http://www.federico-valerio.it/wp-content/uploads/2014/01/MPC-environ-impact.pdf (accessed on 14 September 2018).
[125] Van Ewijk, S., J. Stegemann and P. Ekins (2017), “Global Life Cycle Paper Flows, Recycling Metrics, and Material Efficiency”, Journal of Industrial Ecology, http://dx.doi.org/10.1111/jiec.12613.
[107] Wang, Y. (2016), Remanufacturing Mission to China, https://connect.innovateuk.org/web/remanufacturing/article-view/-/blogs/new-remanufacturing-standards- (accessed on 13 September 2018).
[27] Warsen, J., M. Laumer and W. Momberg (2011), “Comparative Life Cycle Assessment of Remanufacturing and New Manufacturing of a Manual Transmission”, in Glocalized Solutions for Sustainability in Manufacturing, Springer Berlin Heidelberg, Berlin, Heidelberg, http://dx.doi.org/10.1007/978-3-642-19692-8_12.
[42] WEF (2016), Understanding the Sharing Economy, http://www3.weforum.org/docs/WEF_Understanding_the_Sharing_Economy_report_2016.pdf (accessed on 14 September 2018).
[133] WEF (2016), Understanding the Sharing Economy, http://www3.weforum.org/docs/WEF_Understanding_the_Sharing_Economy_report_2016.pdf (accessed on 13 September 2018).
[30] Wilson, J. et al. (2014), “Remanufacturing of turbine blades by laser direct deposition with its energy and environmental impact analysis”, Journal of Cleaner Production, Vol. 80, pp. 170-178, http://dx.doi.org/10.1016/j.jclepro.2014.05.084.
[101] World Steel (2016), Crude Steel Production, https://www.worldsteel.org/statistics/statistics-archive/steel-archive.html.
[84] WRAP (2011), Realising the Reuse Value of Household WEEE, http://www.wrap.org.uk (accessed on 13 September 2018).
[3] WRAP (2010), Environmental benefits of recycling, http://www.wrap.org.uk (accessed on 14 September 2018).
[10] WRAP (2010), “Environmental benefits of recycling – 2010 update 1”, http://www.wrap.org.uk/sites/files/wrap/Environmental_benefits_of_recycling_2010_update.3b174d59.8816.pdf (accessed on 28 March 2018).
[15] WRAP (2006), Environmental benefits of recycling, https://cri.dk/sites/cri.dk/files/dokumenter/artikler/filef7e8.pdf (accessed on 14 September 2018).
[121] WRI (2015), Carsharing: A Vehicle for Sustainable Mobility in Emerging Markets, http://wrirosscities.org/sites/default/files/WRI_Carsharing_Vehicle_Sustainable_Mobility_Emerging_Markets.pdf (accessed on 13 September 2018).
[62] Zink, T. and R. Geyer (2017), “Circular Economy Rebound”, Journal of Industrial Ecology, Vol. 21/3, pp. 593-602, http://dx.doi.org/10.1111/jiec.12545.
Notes
← 1. Even the resource recovery business model, which upon first inspection is only relevant for the recycling sector, affects a number of extractive sectors via the different materials (metals, paper, plastics, biomass, etc) that it collects and processes.
← 2. And also relative to other intuitive approaches; some studies use estimates of avoided resource extraction as a proxy for the associated environmental benefits (e.g., Pothen (2017[84]).
← 3. When assessing the results of “comparative” LCAs, it is also important to keep in mind what the stated counterfactual is. For example, LCAs that focus on processes that extend product lives often assume that the counterfactual is product disposal. In reality, in many cases, not all of the end of life product will be disposed since certain components and materials may be recycled.
← 4. Further, any such displacement may well take place in jurisdictions other than the one in which the circular business model emerges. For example, if increased secondary metal output in country X leads to reduced domestic demand for primary raw materials, the reduction in resource extraction and processing, and any associated environmental benefits, may take place elsewhere.
← 5. An additional level of complexity is introduced by the fact that, for some materials, the degree of substitutability declines with each additional material recovery cycle. The static character of LCA makes such effects difficult to incorporate.
← 6. This is not particularly surprising. It is essentially a restatement of the waste hierarchy concept contained in the European Union’s Waste Framework Directive.
← 7. Defined as total municipal budgetary costs + resource (time) costs incurred by households + external costs associated with landfilling and incineration – (revenues earned from sale of recovered materials + external benefits associated with manufacturing final goods with recycled rather than primary materials less the external costs associated with collecting, transporting, and processing these materials)
← 8. See Cooper and Gutwoski (2017[130]) for a fuller discussion of these issues in the context of reuse.
← 9. IDDRI (2014[23]) use the example of cars and refrigerators.
← 10. As discussed in Section 2.2.3, returning a product to its original condition is central to all definitions of remanufacturing. However, that does not necessarily imply performance levels equal to contemporarily manufactured versions of the same product, even where remanufacturing is complemented with the installation of component upgrades.
← 11. One complication is that, due to increasingly interconnected global value chains, the upstream and use phase environmental impacts will often emerge in different geographic locations.
← 12. It has also been suggested that the term “sharing” has positive environmental connotations in and of itself. This is one explanation for why firms not strictly involved in sharing spare capacity seek to label themselves as sharing models (e.g., EU Observer (2017[96]) and European Commission (2016[85])).
← 13. Any difference in the transport required to use the good is also relevant, but unlikely to be of significant magnitude.
← 14. As discussed further in Section 4.3.4, one potentially positive implication of increased utilisation and more rapid product turnover is the removal of old, relatively inefficient products from the market.
← 15. In reality, only a proportion of these hotels would actually have been built in the absence of Airbnb or other sharing platforms. Increased prices resulting from supply shortages would have served to ration some of the excess demand.
← 16. The authors state that the energy efficiency of typical European and North American residences were compared to those of the most energy efficient hotels (those in the top 5 percentile).
← 17. Some studies take a different approach. Haefili et al. (2006) ask individuals without access to a car sharing scheme to describe their response to the hypothetical introduction of one. Firnkorn and Muller (2011[102]) ask individuals about their planned response to a recently introduced car sharing scheme.
← 18. Cervero, Golub and Nee (2007[107]) partially address these concerns by utilising a difference in difference approach. The behaviour of members and non-members is documented at two times: the introduction of a car sharing scheme and five years later. In theory, this allows changes in transport behaviour not associated with car sharing to be isolated.
← 19. The authors demonstrate this with the example of repaired or refurbished smartphones. According to them, second hand phones are seen as poor substitutes for new phones in developing countries. As such, they are often exported and sold in developing countries where the alternative is no phone at all.
← 20. Or, as stated by ADEME (2016[92]) in the context of sharing models, “collaborative consumption does not necessarily signify responsible consumption. Indeed, the environmental gain depends strongly on the traditional activity that the collaborative activity replaces”. The potential replacement of public (rather than private) modes of transport by car-pooling and sharing is one such example.
← 21. To further complicate matters, individual business models do not necessarily operate in isolation, but can interact with each other and, to some extent, become mutually reinforcing.
← 22. Some of these environmental benefits – particularly those associated with reduced natural resource extraction and processing – will probably accrue in third party jurisdictions (i.e., regions with large extractive sectors).
← 23. Some business models are probably less susceptible to rebound effects than others. For example, the underlying business case for adopting the classic long life business model is that manufacturers can charge a premium for products that are more durable. As the emergence of the business model is driven primarily by changes in consumer preferences, rather than increasing price competitiveness, there is less potential for rebound effects.