To maximise the potential of space-based services in tackling global challenges, the orbital environment needs to remain accessible for multiple users and future generations. This chapter tracks recent and significant changes in the use and distribution of resources such as orbital slots and the electromagnetic spectrum and discusses how this could affect the vitality of the space innovation ecosystem and future growth.
The Space Economy in Figures
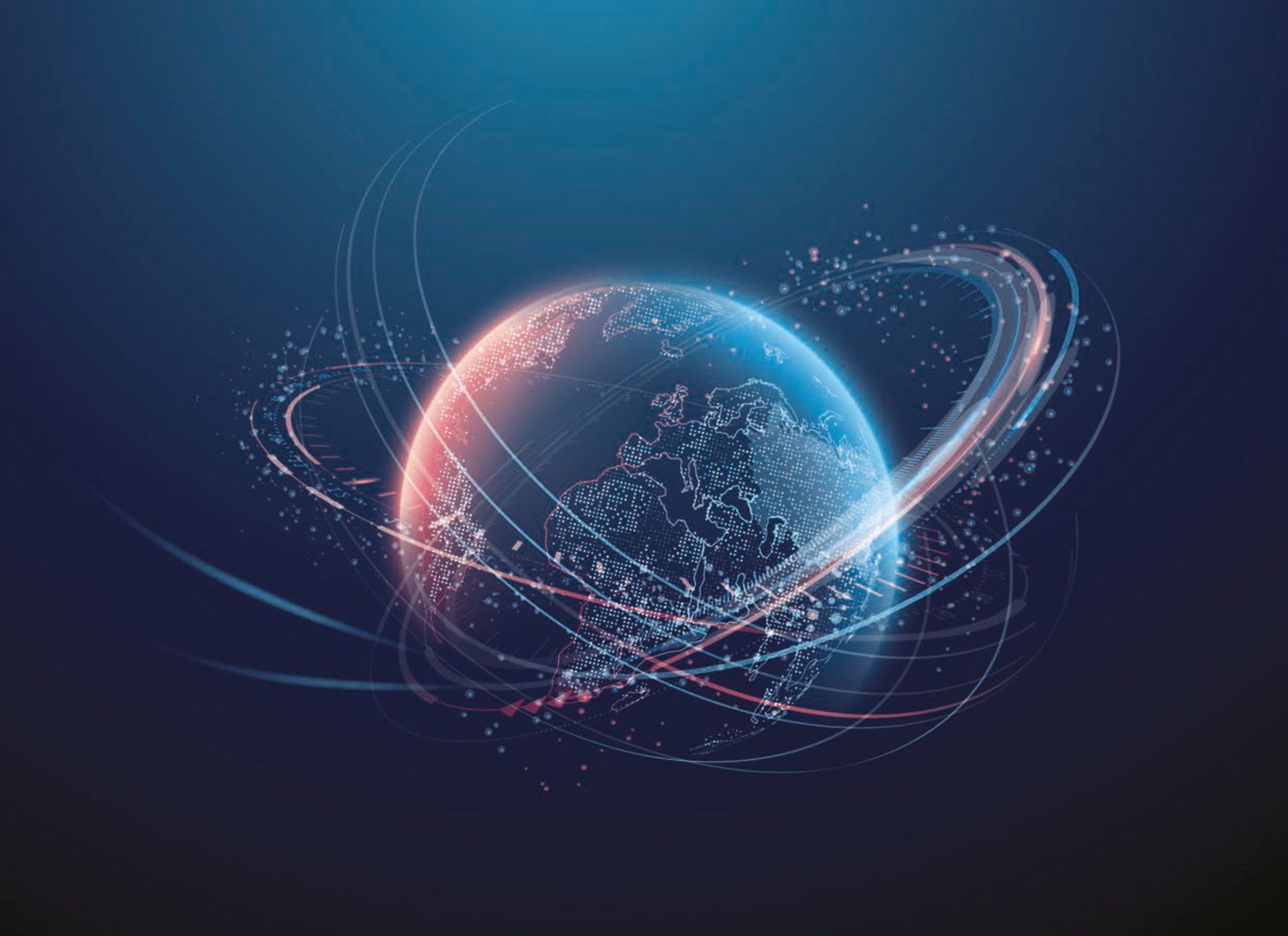
3. Managing a growing space economy
Abstract
Introduction
As described in the previous chapters, space technologies and space-based infrastructure have today unprecedented potential to contribute to managing global challenges. However, for this to happen, existing barriers to entry to space must be further lowered, and a healthy level of competition between actors needs to be maintained, to ensure that the benefits of space activities are distributed as widely as possible.
Space is unlike any other natural environments accessible to humankind. Parallels are sometimes drawn with airspace or the high seas, but space is in most aspects unique, in terms of difficulty of access, remoteness, size, and strategic and geopolitical significance. As a result, doing business in space is generally expensive, technologically challenging, risky and associated with a significant amount of government regulations and red tape.
These elements have shaped the space industry in OECD countries, traditionally characterised by a limited number of private firms working alongside government agencies, with strategic technologies and knowhow controlled by national interests (Undseth, Jolly and Olivari, 2021[1]). After decades of government-controlled activities, the first wave of commercialisation took place in the 1980s and 1990s, with the privatisation of satellite operations and the emergence of geostationary telecommunications services. Further commercial telecommunication projects appeared (and crashed) as part of the dot.com bubble towards 2000, followed by the first commercial or public/private earth observation missions (Undseth and Jolly, 2022[2]).
The current phase of commercial investments took off in the early 2010s, closely linked to the growth of the “new space” ecosystem described in Chapter 1 and boosted by product innovations reducing the costs of access to space, government policies favouring commercialisation and improved access to equity finance (OECD, 2023[3]). At the same time, a growing number of economies are mastering sophisticated space technologies. All this has led to unprecedented levels of launch frequency and orbit occupancy.
Although space is vast, there are definitive first mover advantages in occupying specific orbital slots or electromagnetic frequencies, which can then be renewed indefinitely. There can also be other economic or military advantages (McClintock, Langeland and Spirtas, 2023[4]). All this is leading to several ongoing “space races” – between countries and between conflicting commercial interests – not only for orbital space and the electromagnetic spectrum but also for space “real estate” on celestial bodies, all of which have knock-on effects on launch activity and capacity.
As a result, policymakers have several issues to consider:
How to balance the efficient use of resources with equitable access, not only from a socio-economic and geographic standpoint but also for future generations?
How to optimise innovation performance, entrepreneurship, and intensity of competition in the space sector?
How to address resource extraction and property rights in space?
This chapter looks at recent trends in accessing and using the space environment and discusses these questions in greater detail.
An increasingly diverse population of space actors with growing capabilities
One of the key legacies of “new space” is the democratisation of space technologies and resource exploitation, both in the geographic sense and from a user perspective, thus maximising the potential gains of space exploitation. There are more opportunities for small, innovative actors, such as universities and start-ups, and commercial operators now dominate in both the geostationary and low-earth orbits (UCS, 2023[5]).
Figure 3.1. Almost 100 countries having had a satellite in orbit
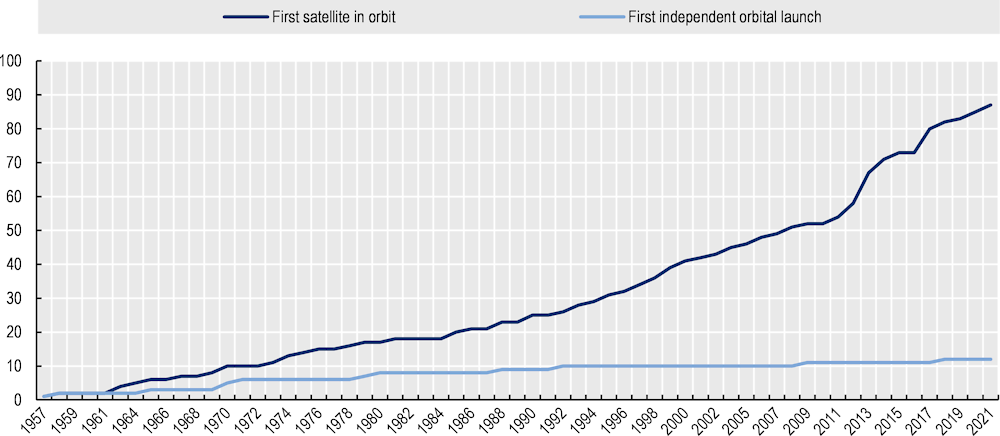
Source: Updated from OECD (2022[6]), OECD Handbook on Measuring the Space Economy, 2nd Edition, http://doi.org/10.1787/8bfef437-en.
Furthermore, geographic diversity has never been higher. By late 2023, almost 100 countries on four continents had operated a satellite at some point in time, with a distinct jump after 2012 (Figure 3.1). Lower-income countries are also better represented than before, with 12 new lower-middle and two low-income countries having operated their first satellite since 2012 (UCS, 2023[5]).
This diversity is also reflected in exploration and science missions, with increasingly ambitious projects pursued by emerging and commercial actors (see Table 3.1).
Table 3.1. Selected “firsts” in lunar exploration
Years of first successful missions. Selected unsuccessful attempts are in brackets
Country/region |
Flyby |
Orbiter |
Impactor |
Lander |
Robotic sample return |
Rover |
Crewed lander |
---|---|---|---|---|---|---|---|
United States |
|
1966 |
1964 |
1966 |
1971 |
1969 |
|
Russia/USSR |
1959 |
1966 |
1959 |
1966 |
1970 |
1970 |
|
Japan |
1998 |
1990 |
(2022) |
(2022) |
|||
Europe |
2003 |
||||||
China |
2007 |
2013 |
2020 |
2013 |
|||
India |
2008 |
2008 (first to impact on the lunar South Pole) |
2023 (first to land in the lunar polar region) |
2023 |
|||
Luxembourg |
2014 |
||||||
Israel |
(2019) First privately funded lander mission |
||||||
Italy |
2022 |
||||||
Korea |
2022 |
||||||
United Arab Emirates |
(2022) Lost with Japanese lander |
Take for example lunar exploration, where several newcomers have launched independent missions or contributed landers and rovers in the last two decades. The strategic objectives of these actors are mixed, including prestige, science, technology demonstration and the preparation of steps for further exploration and resource exploitation. India’s 2009 mission found traces of water on the lunar poles, which spurred additional research efforts in the following years.
Japan was the first country after the United States and the Russian Federation [hereafter ‘Russia’]/USSR to carry out a successful mission to the Moon (orbiter) in 1990. The European Space Agency, the People’s Republic of China [hereafter ‘China’] and India followed in 2003, 2007 and 2008, respectively. A Luxembourg flyby mission was successfully launched by a Chinese spacecraft in 2014, while Israel had an unsuccessful landing attempt in 2019 – this was the first-ever privately funded lunar lander mission. Also in 2019, the Chinese Chang’e 4 spacecraft made a historic first landing on the “dark side” of the Moon, a technological feat requiring a relay satellite and considerable system autonomy, as the lunar surface blocks the line-of-sight and direct communication lines with Earth. In 2022 there were missions by three country newcomers: Italy, Korea and the United Arab Emirates, as well as several commercial participants in NASA’s Commercial Lunar Payload Services programme. The Emirate rover was lost with its (commercial) Japanese landing craft.
Established actors are also taking a renewed interest in the Moon. The US Artemis programme, including both robotic and human exploration, was launched in 2017. Russia launched a lunar mission in 2023 for the first time in 45 years (which failed) and is planning a crewed mission by 2030, as are the United States and China.
Figure 3.2. Greater geographic spread in scientific excellence in space and planetary science
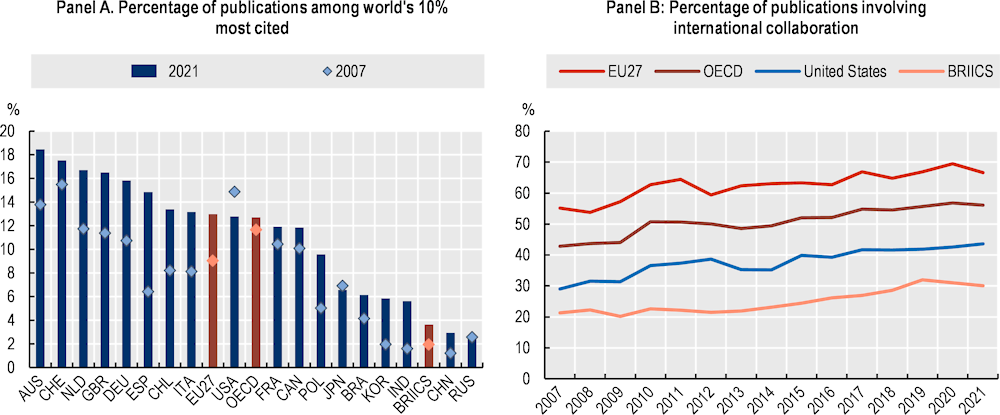
Notes: For countries with 100 or more scientific publications in 2021, fractional counts. Publications are attributed to countries based on the authors’ institutional affiliations. BRIICS: Brazil, Russia, India, Indonesia, China and South Africa.
Source: OECD calculations based on Scopus Custom Data, Elsevier, Version 1.2023.
As for the exploration of Mars, the European Space Agency became the third country/country grouping with a successful Mars orbiter mission in 2003 (the accompanying lander, Beagle-2, failed), after the United States and Russia, and was followed by orbiters of India (Mars Orbiter Mission orbiter in 2013) and the United Arab Emirates (Hope, 2020). China had its first successful Mars mission in 2021, including an orbiter, lander and rover, making it the second country to successfully deploy a rover on Mars. Japan plans a mission in 2024 to explore Mars’ moons, including a sample return. The European ExoMars Rosalind Franklin mission, including the first European rover on Mars, is currently scheduled to launch in 2028, after delays caused by COVID-19 and the war in Ukraine. Meanwhile, NASA’s Ingenuity helicopter, part of its Mars 2020 mission, had completed more than 50 flights by 2023 and is the first aircraft to achieve powered, controlled flight on a different planet.
In astronomy and astrophysics, the US James Webb telescope, with contributions from Canada and Europe, was finally launched in 2022 and has already produced imagery of unprecedented detail. Other space-based telescopes from Japan (XRISM) and Europe (Euclid) will be launched in 2023. China’s Xuntian telescope is scheduled for launch in 2024.There are also several recent and future missions to study the Sun and the heliosphere, such as the US Parker Solar Probe and the Chinese ASO-S observatory launched in 2022.
Finally, several powerful Earth-based observatories were near finalisation in 2023, including the optical/near-infrared Extremely Large Telescope in Chile, operated by the European Southern Observatory, and the Square Kilometre Array in Australia and South Africa, a network of thousands of antennas simulating one giant radio telescope. It is worth noting that the increased number of satellites in low-earth orbit leads to more light pollution and radio interference, which could be harmful to optical and radio astronomy (more on this in Chapter 4).
Figure 3.3. Evolution of space-related patents
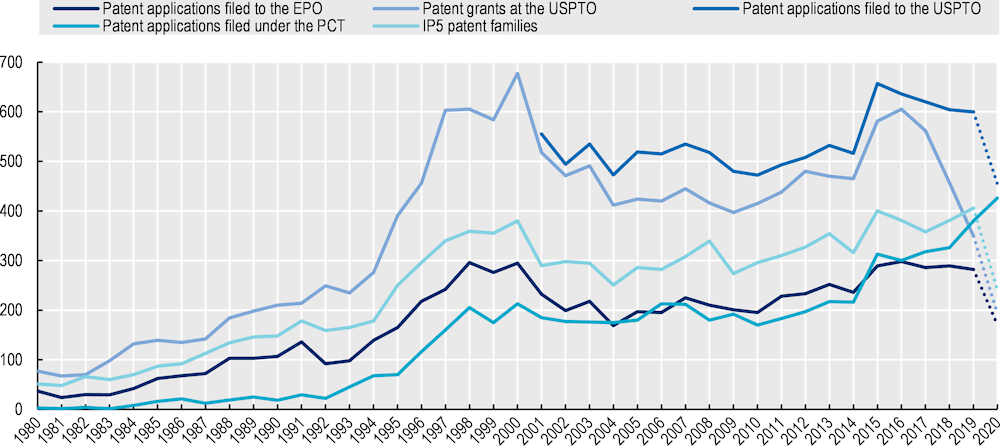
Notes: Partial information for patent applications filed to the EPO and for IP5 patent families from priority year 2019 and partial information on USPTO patents for the latest years. IP5 patent families correspond to patent families filed in at least two offices worldwide, including at least one of the Five IP largest offices (IP5, i.e. the European Patent Office, EPO; the Japan Patent Office, JPO; the Korean Intellectual Property Office, KIPO; The China National Intellectual Property Administration, CNIPA; and the US Patent and Trademark Office, USPTO).
Source: OECD, STI Micro-data Lab: Intellectual Property Database, http://oe.cd/ipstats, June 2023.
Increased international participation in space activities creates new opportunities further downstream, with new actors intervening now in space sciences and engineering, as well as research and development. Figure 3.2 shows how many countries and economies have seen an increase in their scientific publications that are among the world’s top-cited in space and planetary science since 2006 (Panel A). This could, among other things, be linked to the increase in international collaboration and co-authorships (Panel B). Among BRIICS economies (comprising Brazil, Russia, India, Indonesia, China and South Africa), the share of scientific publications in space and planetary science with international co-authors grew from 21% in 2007 to 30% in 2021. In the OECD area, more than half (56%) of scientific publications in this field had international co-authors in 2021. Publications in the European Union had the highest share of international collaboration (67%) among the groups of economies, reflecting the high share of international co-operation in European space activities more generally through the European Space Agency, as well as European participation in US science projects.
Figure 3.4. Patents for space-related technologies per economy
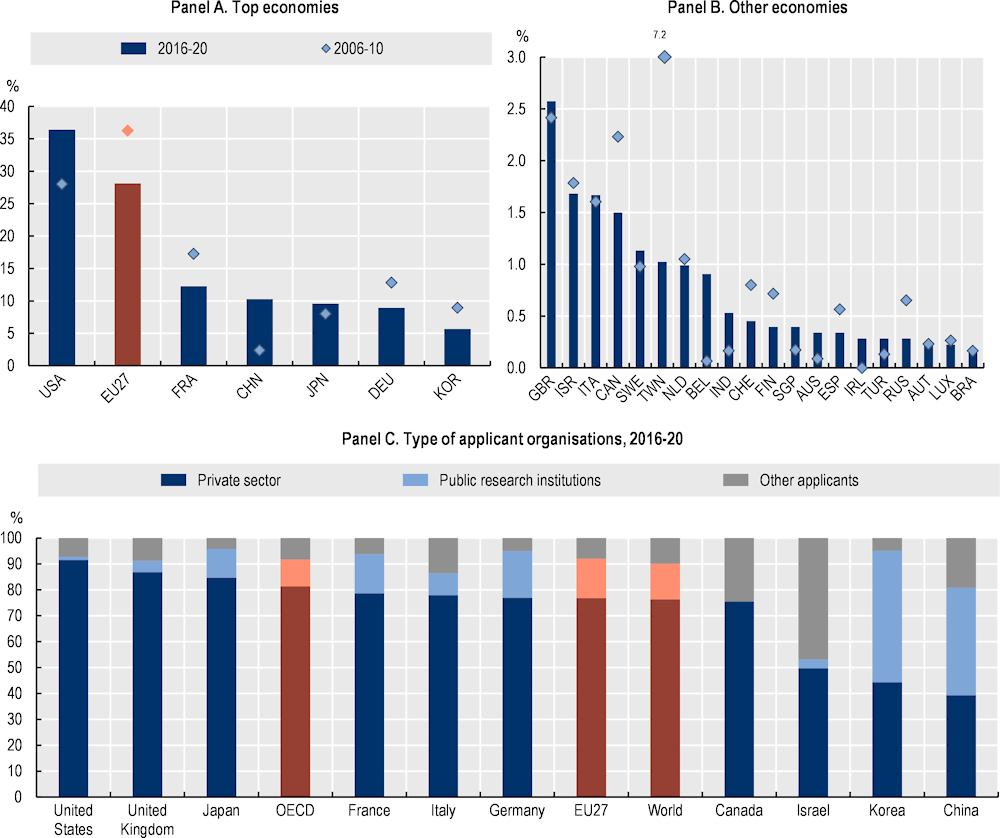
Notes: Patent families are compiled using the information on patent families within the Five IP offices (IP5). IP5 patent families correspond to patent families filed in at least two offices worldwide, including at least one of the Five IP largest offices (IP5, i.e. the European Patent Office, EPO; the Japan Patent Office, JPO; the Korean Intellectual Property Office, KIPO; The China National Intellectual Property Administration, CNIPA; and the US Patent and Trademark Office, USPTO). Figures are based on incomplete data from the year 2019. “Other applicants” include private individuals, universities and private non-profit organisations.
Source: OECD, STI Micro-data Lab: Intellectual Property Database, http://oe.cd/ipstats, June 2023.
Figure 3.3 tracks the number of space-related patent applications filed at patent offices worldwide between 1980 and 2020, reaching some 600 applications to the US Patent Office (USPTO) in 2019, as recorded by OECD analysis based on a combination of keyword and technical category searches (for the full search methodology, see (OECD, 2022[6])). There has been a notable increase in applications in the last 10-12 years (after a post-dot.com bubble slump in the early 2000s), coinciding with the growth in the “new space” innovation ecosystem and the expansion of space programmes in economies such as India and China. Other patent analyses that include national patent filings (giving only domestic patent protection) at the China National Intellectual Property Administration find explosive growth in Chinese space-related patents (Clarke et al., 2021[7]).
The share of applications in “cosmonautics” has grown considerably in the last decade, reaching 38-42% of applications in the 2016-20 period, up from 19-26% in 2006-10. Cosmonautics refer to technologies associated with spacecraft manufacturing, launch and control, such as propulsion systems and structures (Clarke et al., 2021[7]). Meanwhile, the share of applications associated with satellite navigation has more than halved, from 33% of space-related applications filed to the US patent office in 2006-10 to 12.6% in 2016-10.
Figure 3.5. Top 20 regions in space-related patents
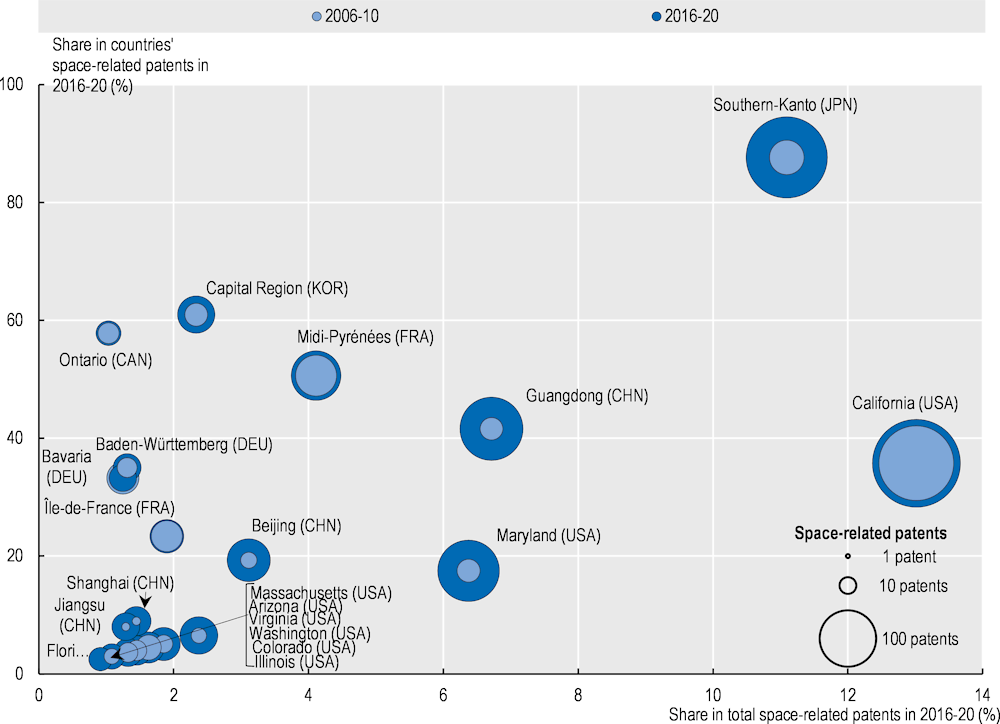
Note: Data refers to patent applications filed under the Patent Cooperation Treaty (PCT), by the inventor's region at Territory Level 2 (TL2) and priority date.
Source: OECD, STI Micro-data Lab: Intellectual Property Database, http://oe.cd/ipstats, June 2023.
At the national level (Figure 3.4), patenting activity has slowed down in Western Europe and Russia in particular, coinciding with strong growth in China, India and the United States. In the 2016-20 period, the majority of applicant organisations were business firms, but public research organisations accounted for a notable share in several economies, in particular in China and Korea. In addition to business firms and public research organisations, patent applications can also be filed by private individuals, universities and private non-profit organisations, accounting for the “other applicants” category in the figure.
Figure 3.5 shows patent applications at the regional level, notably their share in countries’ space-related patents (y-axis) and the total number of space-related patents (x-axis). Patent analysis at this level of granularity reveals important industrial clusters such as the French Southwest (Midi-Pyrénées) and Guangdong in China. In 2016-20, US regions accounted for nine out of the top 20 patent-filing regions, followed by China (4). Four European regions (in France and Germany) were among the top 20 in 2016-20, compared to eight in 2006-10. Other notable regions include Southern Kanto in Japan, Capital Region in Korea and Ontario in Canada, each home to inventors filing the majority of space-related patent applications in their respective countries.
First come – first served with Earth’s orbits up for grabs
Strategic competition in space activities is not new; it fuelled considerable innovation activity during the Cold War. However, in the last five years, there have been several profound changes in space launch activity with long-term impacts that are yet to be felt in the space community and beyond. First, in terms of launch frequency and the unprecedented volume of launched objects; second, in launches by country, which has important geopolitical implications; and finally, in the growth of commercial activity, posing new questions about space access and ownership.
Strong growth in launch activity among “new” actors
Whereas a growing number of countries have demonstrated orbital launch capability, China and the United States carried out by far the highest number of launches in 2022. The United States carried out 76 successful launches, followed by China (62) and Russia (21), breaking the record of launches per year (McDowell, 2023[8]).
Most US launches were carried out by a relative newcomer, the private company Space Exploration Technologies Corporation [hereafter SpaceX], which with a record 61 launches accounted for 80% of the US total. US launch activity now surpasses previous peak years in the 1960s and 1990s, as illustrated in Figure 3.6.
China, with its 64 successful launches also set a record in 2022, surpassing the previous one of 48 in 2021. This is part of the country’s ambitious space programme, comprising human spaceflight (its space station is completed and inhabited), exploration (it is the second country to successfully land a Mars rover) science, as well as various government applications. In addition, several Chinese commercial actors have emerged since the deregulation of Chinese space activities in 2015 (OECD, 2019[9]). Combined commercial and government launches may surpass 70 in 2023 (Jones, 2023[10]).
New Zealand is a recent addition to the international launch scene. Original home to the US-headquartered firm Rocket Lab, the country carried out nine successful launches from its North Island launch base in 2022.
In contrast, Europe, using the Guiana Space Centre in French Guiana, carried out only six launches in 2022, with one launch failure of the new Vega-C in December 2022. In July 2023, Ariane 5 had its final flight, closing a 27-year successful chapter of Europe’s access to space. The inaugural flight of Ariane 6 is now expected in 2024. Europe also lost access to the Russian Soyuz launcher following the invasion of Ukraine. As the European Space Agency prepares for Vega-C’s safe return after a launch failure, it is proceeding with launch campaigns for the Vega launcher (Vega-C’s more lightweight predecessor). In October 2023, Vega mission VV23 successfully lifted off the Guiana Space Centre, carrying Thailand’s THEOS-2 earth observation satellite, Chinese Taipei’s FORMOSAT-7R/Triton weather satellite and ten nanosatellites from various operators.
India carried out five launches, including the first flight of the small new satellite launch vehicle SSLV; and the Islamic Republic of Iran [hereafter Iran], Japan and Korea each carried out one launch. Korea’s launch was the first successful attempt of the new domestic Nuri launch vehicle to reach orbital velocity after a failure in 2021.
Figure 3.6. Recent and longer-term orbital launch trends
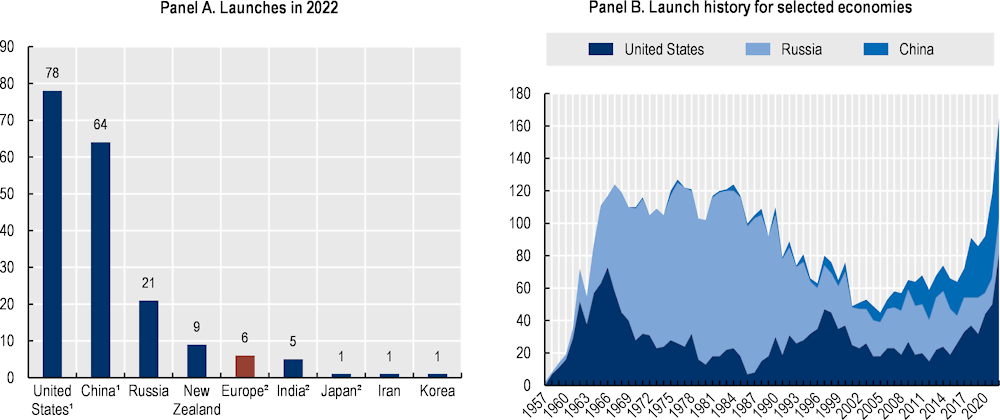
1. Includes two launch failures, 2. Includes one launch failure.
Sources: McDowell (2023[8]), “Space activities in 2022”, https://planet4589.org/space/papers/space22.pdf and (US Space Force, 2022[11])
Despite this diversity of players, just two countries – the United States and China – currently dominate launch activities, the bulk of which is devoted to the deployment of commercial broadband services (see Chapter 1). These satellites, while weighing more than 100kg, are still considered “small” and can be stacked within the launch vehicle and sent into orbit in batches, 40-50 satellites at a time.
Renewed interest in launch opportunities across the world
The intensified use of the low-earth orbit with constellations of dozens, hundreds or thousands of satellites that need to be regularly launched and replaced (see Chapter 1), is considerably expanding the potential market for launch services. The result has been a marked growth in commercial launcher developments in the last five years.
A growing number of countries (or regional authorities) also seek to develop spaceports to exploit a favourable geographic location and to get a share of this new economic opportunity.
Ever more spaceports
Between 2018 and mid-2023, 11 economies/regions have demonstrated orbital launch capabilities from one or several facilities: Six with only domestic (and mainly government) launches (Islamic Republic of Iran, Israel, the Democratic People’s Republic of Korea, Korea, Japan and the Russian Federation) and five with both domestic and international customers: China, India, Europe (in French Guiana), New Zealand and the United States.
Several countries on four continents are planning new or extending existing spaceports or military/scientific facilities, thus exploiting distinct geographic advantages such as latitude, stable weather conditions, and an east-facing, coastal and/or sparsely populated location. Locations near Equator are generally favourable for most launches, in particular to the geostationary orbit and interplanetary missions, due to Earth’s ellipsoidal shape (bulging at the middle), which increases rotational speed and gives additional momentum at launch. However, for several low-earth orbits (e.g. polar orbits, certain sun-synchronous orbits) and high-elliptical orbits, higher-latitude launch sites may be interesting.
In the Americas, the United States had 13 spaceports and launch/re-entry sites across nine federal states in 2023, including three for exclusive use by launch vehicle manufacturers SpaceX (2) and Blue Origin (FAA, 2023[12]). In Canada, the government announced in 2023 its intention to create a regulatory licensing framework for commercial launches (Transport Canada, 2023[13]). A spaceport is under construction in Nova Scotia. Meanwhile, Brazil is trying to attract international customers to its equatorial Alcântara Space Center (which is not yet operational for orbital flights). A Korean start-up carried out a suborbital launch at the site in 2023, and Brazil and the United States entered a technology safeguard agreement in 2019, which allows for US-licenced satellites or space vehicles to launch from Alcântara (US Department of State, 2019[14]).
In Western Europe, in addition to the existing equatorial Kourou Space Centre in French Guiana, some six countries (Germany, Norway, Portugal, Spain, Sweden, and the United Kingdom) are developing or considering commercial spaceports and building partnerships with different launch providers. In January 2023, the first orbital launch attempt from Western Europe (from Spaceport Cornwall, United Kingdom) failed, while another test flight, this time from the Andøya Space Centre in northern Norway, is also scheduled for 2023. This Norwegian launch site was officially opened in the second half of 2023.
In Asia, both China and India are strengthening their capabilities to attract commercial customers, both domestic and international. China opened for private space activities in 2014 and is building a new commercial launch facility next to its Wenchang spaceport, with activities set to commence in 2024 (Jones, 2023[15]). India has made several reforms in 2019/2020, enhancing the mandate of its NewSpace India Limited (NSIL) undertaking to commercialise Indian space services (including launch) and create the Indian National Space Promotion and Authorisation Centre (IN-SPACe) for facilitating private sector participation (ISRO, 2023[16]). India launched its first privately-made space rocket Prarambh (to 89.5km suborbital altitude) in 2022. In Southeast Asia, both Thailand and Viet Nam are studying the feasibility of domestic spaceports. In 2023, Thailand and Korea signed an implementation agreement for studying the feasibility of a Thai spaceport (Kim, 2023[17]), while private firm ThaiHoldings is considering building a spaceport for tourism on the Vietnamese island Phu Quoc by 2026 (Inoue and Shiga, 2023[18]).
Finally, in Oceania, New Zealand launched its first satellite in 2017 and is one of the spaceports of the operator Rocket Lab (which also has US operations). Australia launched its first satellite in 1967 and has multiple projects underway, including in the Northern Territory, Queensland and South Australia (BryceTech, 2023[19]).
A growing number of dedicated launchers for nanosatellites
In close connection to the development of spaceports, a remarkable number and variety of small launchers specifically dedicated to the low-earth orbit market are currently being developed (Kulu, 2023[20]). Projects range from purely government-backed to fully privately-funded, some employing airborne launch systems while others launch vertically (including one using a catapult) with different degrees of reusability; and some in their very early stages while others have 100+ employees and have raised several hundred USD million in venture capital. About 19 rockets have been launched at least once, while at least another 60 launchers are in various stages of development – counting only those to be launched by 2030. In Western Europe alone, there are at least 19 projects from France (5), Germany (4), Italy (1), Norway (1), Spain (2) and the United Kingdom (5) (Kulu, 2023[20]), as shown in Figure 3.7.
These projects fit into a broader ecosystem of actors exploiting the opportunities of low-earth orbits, with multiple nanosatellite operators and a growing number of spaceports at multiple locations (see the previous section). The objective is to provide flexible launch opportunities (compared to today’s situation where it may take several months if not years to book a slot on a suitable launch). This applies particularly to actors with limited nearby launch options, such as those that are based in Europe.
Figure 3.7. Selected microlauncher projects
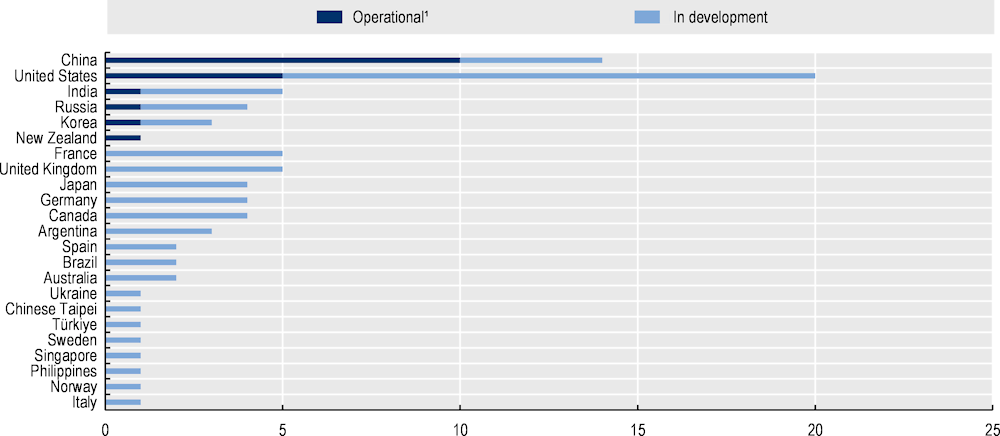
Notes: Microlaunchers” have a maximum launch capacity of 1 500 kg to sun-synchronous orbit (SSO); 1. Operational launchers have carried out at least one launch attempt (successful or unsuccessful).
Source: Adapted from Kulu, (2023[20]), “Small satellite launchers”, NewSpace Index website, www.newspace.im/launchers.
However, competition will be fierce from medium and heavy-lift launchers capable of launching several dozens of satellites at the same time (i.e. providing “rideshare” services). Both SpaceX’s Transporter rideshare programme and the Indian Polar Satellite Vehicle have launched more than 100 very small satellites (or payloads) in one go. In China, the China Great Wall Industry Corporation, which is the international and commercial branch of the China Aerospace Science and Technology Corporation (CASC), performed its third commercial rideshare mission in January 2023.
Growing pressure on medium- and heavy-lift launchers
The coming years’ deployment of mega-constellations will rely on medium- and heavy-lift launchers capable of carrying up to 20 000 and 50 000kg to orbit, respectively, but supply is uncertain as legacy launchers are retiring and new projects face delays. The war in Ukraine is also limiting the use of Russian launchers and launch services internationally (Undseth and Jolly, 2022[2]).
As it stands, three reliable launchers, the European Ariane 5 launcher, US Atlas V and Japanese H-2A retired in 2023 or are set to retire towards 2025. Their replacements, European Ariane 6 and US Vulcan Centaur are scheduled to have their first flight in 2024. Several US commercial launchers are scheduled to enter the market in 2024 (Table 3.2).
In Japan, the H3 rocket had a failed launch attempt in March 2023. The Indian Space Research Organisation (ISRO) has also announced the eventual retirement and replacement of its Polar Satellite Launch Vehicle by a next-general launch vehicle without giving a specific end date, with private industry able to continue the production if there is commercial demand (The Hindu, 2022[21]).
Table 3.2. Medium- and heavy-lift launchers
Country |
Organisation |
Launch vehicle and first flight |
Launcher category |
Launch capacity |
|
---|---|---|---|---|---|
Operational |
Under development (early 2023) |
||||
United States |
United Launch Alliance |
Atlas V (2002, retiring) |
|||
Delta IV Heavy (2004) |
|
Heavy |
28 790kg |
||
|
Vulcan Centaur (2024) |
Heavy |
25 000kg |
||
SpaceX |
Falcon 9 Full Thrust (2015) |
|
Heavy |
22 800kg |
|
Falcon Heavy (2018) |
|
Heavy |
38-45 000kg |
||
Blue Origin |
|
New Glenn (2024) |
45 000kg |
||
Relativity Space |
|
Terran R (2024) |
Medium |
20 000kg |
|
Rocket Lab |
|||||
China |
China Academy of Launch Vehicle Technology |
Long March 5/5B (2016) |
|
Heavy |
25 000kg |
Russia |
Khrunichev |
|
Proton M (2001) |
Heavy |
23 000kg |
Angara-A5V (2014) |
|
Heavy |
38 000kg |
||
Japan |
Mitsubishi Heavy Industries |
H2A (retiring) |
|||
H3 |
|||||
India |
ISRO |
|
HLV (under consideration) |
Medium |
20 000kg |
|
SHLV (under consideration) |
Heavy |
41 300kg |
||
Europe |
ArianeGroup |
Ariane 5 ECA (2002, retiring 2023) |
Heavy |
||
Ariane 6 (2024) |
Heavy |
21 650kg |
More heavy-lift launchers to support exploration and space infrastructure development
With the low-earth orbit increasingly dominated by commercial activity, several governments have ambitious plans for crewed space exploration and space infrastructure expansion. This requires powerful rockets, so-called “superheavy” or super heavy-lift launchers that can carry more than 50 000kg to orbit (Table 3.3). Launchers in different stages of development include NASA’s Space Launch System (first launched in 2022), which will be instrumental in deploying the agency’s Artemis programme for lunar exploration, and the Lunar Gateway space station that will orbit the Moon; SpaceX’s Starship, destined for exploring Mars by the end of the decade (another US company, Relativity Space, has announced plans to reach Mars with its Terran R launcher in 2024). Europe will eventually roll out its Ariane 6 launcher in 2024 and China plans several heavy-lift launch vehicles by 2030.
In addition to a dramatically increased ability to transport heavy and voluminous objects, these launchers are expected to bring down launch costs, as discussed in Chapter 1. Applications of superheavy launchers include the possibility to deploy entire constellations of small satellites in one single launch; or to carry larger space infrastructures, instruments and systems (e.g. space stations, solar power stations). They could also support other experimental activities mentioned in Chapter 1, such as in-space manufacturing and, eventually, point-to-point suborbital travel.
Table 3.3. Selected super heavy-lift launch vehicles
Country |
Organisation |
Launch vehicle and first flight |
Launch capacity |
|
---|---|---|---|---|
Operational |
In development (early 2023) |
|||
United States |
National Aeronautics and Space Administration |
Space Launch System (2022) |
95 000kg (Block 1)/ 105 000kg (Block 1B)/ 130 000kg (Block 2) |
|
Space X |
Starship (2024) |
150 000kg |
||
China |
China Academy of Launch Vehicle Technology |
Long March 10 (2027) |
70 000kg |
|
Long March 9 (2030) |
150 000kg |
|||
Russia |
JSC SRC Progress |
Yenisei (2028) |
103 000kg |
Orbit occupancy is becoming more diverse and commercial, but also more concentrated
The lowering of launch costs has to a certain extent democratised access to space and attracted the interest of commercial actors.
Figure 3.8. Selected economies’ share of operational satellites
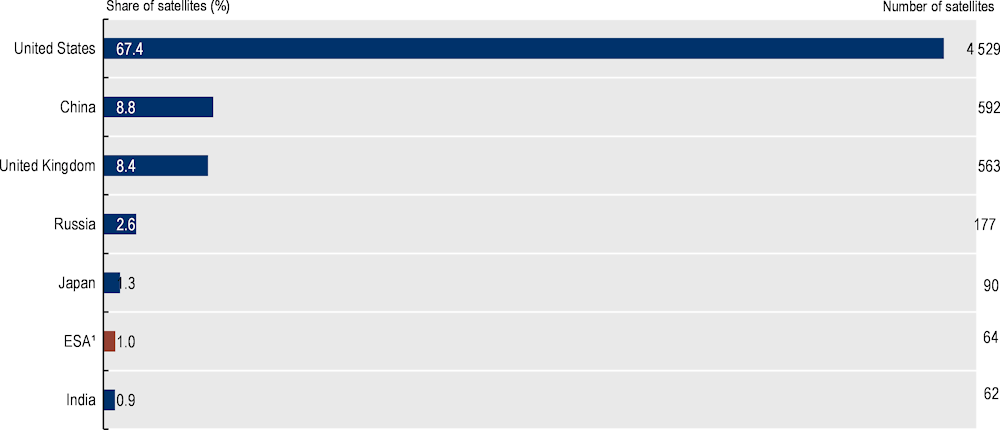
1. ESA=European Space Agency.
Source: Union of Concerned Scientists, “UCS Satellite Database: 01 January 2023 update”, https://www.ucsusa.org/nuclear-weapons/space-weapons/satellite-database, data accessed 07 August 2023.
As a result, upper-middle income economies now account for 13% of operational satellites (mainly from China and Russia), while 100 operational satellites (or 1.9%) belong to low- and lower-middle income economies, as recorded by the satellite database of the Union of Concerned Scientists (2023[5]). This latter group includes most notably India, but also more recent space-faring economies such as Bangladesh and Ethiopia.
Still, big and mainly high-income economies remain the most important actors. As of the end of 2022, the United States accounted for more than 67% of the 6 700 operational satellites in orbit, followed by China (8.8%) and the United Kingdom (8.4%) (UCS, 2023[5]), as is shown in Figure 3.8.
As for the distribution of public and private applications, the satellites of commercial operators dominate in the low-earth and geostationary orbits (Figure 3.9), while government missions have a stronger presence in medium-earth and elliptical orbits.
The rollout of mega-constellations for satellite broadband in the low-earth orbit is leaving its marks. Two operators, SpaceX (United States) and OneWeb (United Kingdom) accounted by the end of 2022 for 74% of all operational commercial satellites. This is likely to even out somewhat when other operators start deploying their constellations (see Section on satellite telecommunications in Chapter 1). Still, first movers have important advantages in terms of slot occupancy, something that will be discussed in the next section.
Figure 3.9. Public and private uses of Earth’s orbits
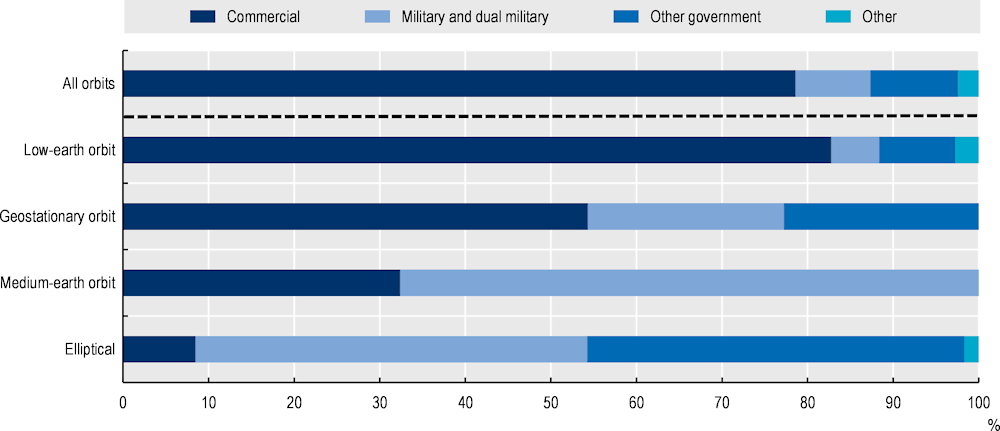
Note: Military and dual military include fully military satellite missions, as well as military-civil, military-government and military-commercial, or a combination of the three.
Source: Union of Concerned Scientists (UCS, 2023[5]), “UCS Satellite Database: 01 January 2023 update”, https://www.ucsusa.org/nuclear-weapons/space-weapons/satellite-database, date accessed 07 August 2023.
Growing competition for orbital slots and frequencies
Space is big, but the demand for specific orbits or slots can surpass supply. Congestion is particularly dire in the geostationary orbit at 35 786 kilometres altitude, where satellites occupy a single ring above the Equator and where the most coveted slots (near specific terrestrial longitudes) are filling up quickly (Ogden, 2022[22]). The geostationary orbit is home to many commercial telecommunications satellites, but it also has important applications in meteorology and air traffic management.
The low-earth orbits have more available space, but also here some orbits are more advantageous than others, for example as regards proximity to Earth and latency of signals (NAPA, 2020[23]), revisit periods, etc.
The allocation of electromagnetic spectrum and access to orbits is managed by the International Telecommunication Union (ITU) at the international level and by various government agencies at the national level, such as the US Federal Communications Commission (FCC), UK communications regulator Ofcom, the Norwegian Communications Authority (Nkom), and so on. Two main principles guide these efforts: efficient use and equitable access (ITU, 2023[24]). Equitable access to resources for future use is ensured by a priori planning procedures, which include
the Allotment Plan for the fixed-satellite service using part of the 4/6 and 10-11/12-13 gigahertz frequency bands (see Box 3.1 for an overview of satellite frequency bands)
the Plan for the broadcasting-satellite service in the frequency band 11.7-12.7 gigahertz
the associated Plan for feeder links in the 14 gigahertz and 17 gigahertz frequency bands.
The efficient use of existing resources is ensured by co-ordination procedures, notably
advance publication and coordination procedures for geostationary-satellite networks (in all services and frequency bands) and non-geostationary-satellite networks in certain frequency bands governed by the No. 9.11A procedure
an advance publication procedure before the notification for other non-geostationary-satellite networks (all pertinent services and certain frequency bands).
Box 3.1. Satellite frequency bands
The frequencies of the radio spectrum range from tremendously low (1-3 hertz) to tremendously high (300-3 000 gigahertz). Satellites use the 1-40 gigahertz super-high frequency portion of the spectrum to transmit signals, with bands designated by letters. For instance, navigation satellites use the L-band, with radio waves that can penetrate clouds, rain and vegetation (but not concrete buildings).
With the growth in space activities, these bands are in increasingly short supply, and congestion has become a significant issue in the lower frequency bands (e.g. the C-band). Higher frequency bands can give access to wider bandwidths but are also more susceptible to signal degradation (signals absorbed by rain, snow or ice). New technologies are being investigated to find ways to use even higher bands, within the extremely high frequency area of the spectrum, such as Q- and V-bands.
Source: ESA (2023[25]) “Satellite frequency bands”, https://www.esa.int/Applications/Telecommunications_Integrated_Applications/Satellite_frequency_bands.
ITU has taken several measures to make more space available in the geostationary orbit. First, it has made efforts to maximise the efficiency of existing slots, with changes in spacing between satellites from three to two degrees of longitude to make it possible to accommodate more satellite slots (up to 180 from 120) (Holmes, 2008[26]). Other technological advances regard ground station performance, satellites’ abilities to exploit available spectrum, etc.
Second, to limit the occurrence of “warehousing” and “paper satellites” where companies file for slots and frequencies to prevent access by competitors, the 2019 World Radio Communications Conference introduced time limits for deployment. It was established that 10% of any constellation in the fixed, mobile, and broadcasting satellite services in the traditional Ku and Ka frequency bands, as well as in the higher Q- and V-bands, must be in orbit within the first two years after the start of deployment, followed by 50% in five years and 100% in seven years (ITU, 2019[27]).
Inequitable distribution of slots and frequencies?
The introduction of measures should ensure more efficient use of Earth’s orbits, but there is a risk that this comes at the expense of the access to space of new (small) entrants and lower-income countries. Indeed, there are considerable first mover advantages – slot occupation is free of charge and once operators obtain a slot, they tend to keep it indefinitely by refiling and replacing old satellites with new ones. Newcomers are faced with both technological and economic obstacles, and the regulatory process can be an additional hurdle. Depending on the country and operator, it can take almost a year to get regulatory approval for spectrum allocation (Bernstein, 2022[28]). It is worth noting that several government agencies in OECD countries are making efforts to simplify and accelerate this process, e.g. the Federal Communications Commission in the United States (Rainbow, 2023[29]).
Furthermore, policies that set time limits between filing and launching satellites are accused of being harmful for actors that do not have the technological abilities, knowhow, or capital to deploy satellites within the allotted time, and which are often based in lower-income countries. These actors furthermore tend to rely on specific frequencies (e.g. C-band for areas with frequent and heavy rainfall), that require more expensive and unwieldy equipment (Purity, 2020[30]). In 2020, 31 African countries launched a bid to protect their orbital slots.
Figure 3.10 shows satellite filings in the geosynchronous (geostationary) and non-geostationary orbits in 2022, showing a notable growth in filings for non-geostationary (referred by ITU as geosynchronous) orbits. The drop in filings for geostationary satellites may be linked to decreases in demand for commercial services in this orbit (see Chapter 1), but it may also be linked to the satellite filing restrictions introduced by WRC-2019.
Figure 3.10. Satellite filings by orbit
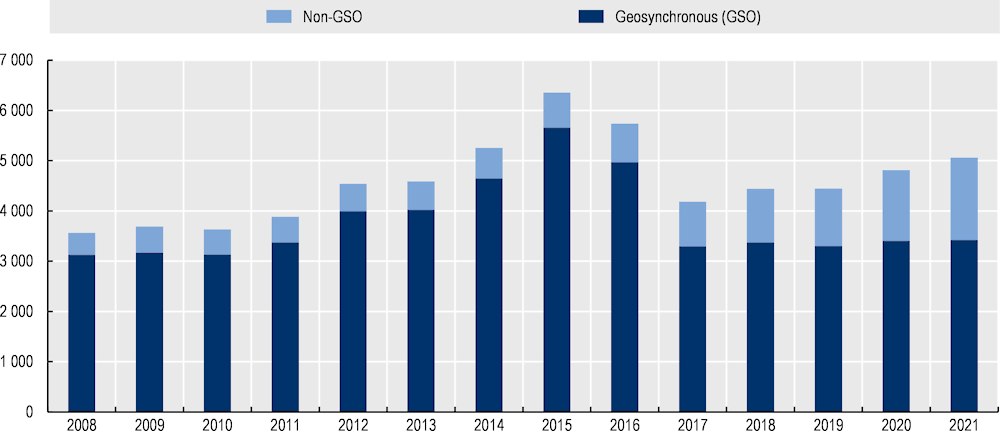
Source: (ITU, 2022[31]), “TU Radiocommunication Bureau (BR) 2021 Annual Space Services Report to the STSC 2022 Session on the use of the Geostationary-Satellite Orbit (GSO) and other orbits”, https://www.itu.int/en/ITU-R/space/snl/SNLReport/SNS-ref-list-2021_e.pdf.
Fears of interference and terrestrial competition for spectrum
There is also the risk of radio frequency interference between satellites in similar orbits, between satellites in different orbits, and with ground stations. Furthermore, terrestrial, and space-based technologies are pitted against each other in the overall distribution of radio spectrum.
The current and planned deployment of communications satellites in the low-earth orbit (LEO) by the thousands is unprecedented, and proposed solutions for avoiding interference are untested. Problems may arise when LEO satellites traverse the signal beam between geostationary satellites and their terrestrial ground stations, and the higher density of satellites in similar orbits may also cause increased interferences between different constellations (Eves, 2021[32]).
There is also growing competition for spectrum from terrestrial operators. One of the items to be discussed during the 2023 World Radiocommunication Conference (WRC-22) is whether to make the upper 6GHz band – currently allocated to fixed satellite services among others – available to new 5G and 6G mobile systems by identifying it for International Mobile Telecommunications (IMT). Mobile telecommunications operators argue that most of the satellite uplink spectrum in C-band is currently not used, and that demand is declining (Euroconsult, 2022[33]). This frequency band is particularly interesting for mobile operators because they should be able to reuse their base stations while shifting from the current 3.7GHz frequency band (Mohyeldin, 2022[34]).
The satellite industry counters that sharing the 6Ghz frequency band with terrestrial operators would increase the risk of harmful interference for several critical space-based services. Affected applications include for instance satellite uplinks for maritime communications, satellite-based navigation augmentation systems and communications with spacecraft for station-keeping and manoeuvring (GSOA, 2022[35]). The upper 6GHz band is also important for radio astronomers, who use it to observe methanol spectral lines, and studies are underway to assess compatibility with mobile telecommunications (CRAF, 2021[36]). Signal interference for radio astronomy is further treated in Chapter 4.
At WRC-23, delegates will further decide whether to allocate additional radio spectrum for earth stations in motion, as well as evaluate progress on spectrum use by non-geostationary satellite constellations and on narrow-band transmissions for the satellite component of the Internet of Things (IoT).
Increased appetite for space-based “real estate” and resources
The intensification of space activities further challenges the principles of space ownership. Article II of the Outer Space Treaty (UNOOSA, 1967[37]) stipulates that:
“Outer space, including the moon and other celestial bodies, is not subject to national appropriation by claim of sovereignty, by means of use or occupation, or by any other means.”
It is therefore not possible to make territorial claims in space. Objects launched into space, on the other hand, are owned by the launching party or state, which would include satellites most obviously, as well as orbiting space stations and lunar bases, for instance.
What then about resources extracted from space? Ambiguity in international law opens for unilateral action. By 2022, the United States, Luxembourg, the United Arab Emirates and Japan had voted laws concerning resources extracted from celestial objects. In November 2022, Japan granted its first commercial license to ispace, a Japanese company, under the country’s Space Resources Act. The license allows ispace to acquire regolith from the lunar surface and sell it to the US National Aeronautics and Space Administration (NASA) through an “in-place” ownership transfer (ispace, 2022[38]). As a result, more commercial actors become increasingly involved in space exploration (e.g. NASA’s Commercial Lunar Payload Services initiative had signed up 14 commercial service providers in 2022).
The 1979 Moon treaty tried to address the exploitation of natural resources in space, but India is the only bigger space nation that has signed (but not ratified) the treaty. In May 2020, as part of the Artemis program, NASA announced the Artemis Accords, a set of principles grounded in the Outer Space Treaty of 1967, to create a safe, sustainable and transparent environment which facilitates exploration, science, and commercial space activities. The non-legally binding Artemis Accords was launched in October 2020 and has 29 signatories as of October 2023 (which does not include China and Russia).
Policy implications
Although the space sector is more diverse in 2023 than in previous decades, it is also more concentrated, with a small number of actors and economies dominating space launch activity, orbit occupancy and venture capital funding. As discussed in this chapter and elsewhere in the book, the space environment needs to be managed responsibly and sustainably for the benefit of all parts of society as well as for future generations.
Ensuring “healthy” levels of competition
An important contribution of “new space” to society is the rise in entrepreneurial activity and ensuing innovation in the global space sector. But this trend is fragile and could easily be reversed. The continuously high economic and regulatory barriers of certain space industry segments increase the risks of entrenchment. Furthermore, as the space sector becomes more digitised, the scalability of intangible digital assets makes “winner-takes-most” scenarios more probable (OECD, 2019[39]).
To sustain adequate levels of competition (see Box 3.2), policy makers are encouraged to make sure national regulatory regimes and procurement processes are favourable for market entrants and not giving incumbents undue advantages. One example is the growing use of Other Transaction Authority agreements by US government agencies (such as Space Act Agreements used for the COTS programme), which are exempt from the administrative requirements of federal procurement laws and regulations and therefore potentially remove some of the “red tape” and possible comparative administrative advantages of larger organisations with more resources and experience than smaller and younger firms (Undseth, Jolly and Olivari, 2021[1]). The US Defense Innovation Unit in the Department of Defense, which was established in 2015 to help young firms navigate the military procurement process, has prototype deals with several earth observation start-ups like BlackSky, Capella and Planet (Kinder, 2023[40]).
Box 3.2. The bidirectional link between innovation and competition
Competition affects innovation and vice versa.
First, one may assume an inverted U relationship between competition and innovation. When the degree of competition is low, there is a positive impact of increased competition on innovation efforts, as it pushes firms to differentiate, gain a competitive advantage and earn profits. However, at a certain level of competition, a further increase may lead to short-term thinking and discourage investments in longer-term riskier research and development, as it decreases laggard firms’ short-term extra profit from catching up with the leader. The link between competition and innovation is further affected by the following factors:
the market needs to be contestable, i.e. with low entry barriers and favourable business conditions
firms need to be able to appropriate the benefits of their innovations
the existence of synergies, i.e. the possibility of combining assets to produce greater benefits and enhance abilities to innovate.
Second, it is increasingly recognised that innovation drives competition as much as competition drives innovation. The extent of the innovation plays a key role as breakthrough innovations guarantee fewer threats of competition, while constant rates of innovation could be a method to secure competitive advantage in rapidly changing environments when the innovations are not as structural.
Source: OECD (2023[41]), Competition and Innovation: A Theoretical Perspective, OECD Competition Policy Roundtable Background Note, www.oecd.org/daf/competition/competition-and-innovation-atheoretical-perspective-2023.pdf.
Another interesting initiative is the UK “sliding scale” policy, which reduces or waives requirements to hold in-orbit third-party liability insurance for low-risk missions (UK Space Agency, 2018[42]). Funding agencies are also increasingly proposing new types of contractual arrangements for R&D procurement and collaboration that impose lesser burdens on firms (e.g. US Other Transaction Authority agreements and simplified contracts). In the United States, the Federal Communications Commission is streamlining its licensing rules for small satellites to make the process easier, faster and less expensive. Similarly, the National Oceanic and Atmospheric Administration now takes 15 days to issue a remote sensing licence, compared to 50-100 days in 2020, thanks to a more standardised treatment of companies and capabilities (Rainbow, 2023[29]).
Reinforcing efforts to better identify and track the outcomes and impacts of space activities
In an increasingly competitive environment where activities, sectors and countries are often pitted against each other – timely and high-quality data and statistics are more important than ever to underpin policy decisions and to understand the value that space brings to society.
Important efforts are underway to better document the role of space activities and space-based infrastructure in the overall economy and common measurement practices are beginning to emerge. Increasing the numbers of solid space economy surveys, for example, means that the quality and coverage of publicly available data and analysis are improving. However, key measurement challenges remain, and beyond strategic and short-term economic benefits, major long-term impacts such as those generated by space science advances in fundamental science, should not be ignored.
The OECD Handbook on Measuring the Space Economy (2022[6]) provides valuable guidance, with revised definitions of space economy terms and concepts, principles for space economy surveys and pointers to conduct impact assessments.
References
[28] Bernstein, L. (2022), Digitalization of Ground Service, Market Changes: What’s Next for GSaaS?, 14 December, https://www.kratosdefense.com/constellations/articles/digitalization-of-ground-service-market-changes-whats-next-for-gsaas.
[19] BryceTech (2023), Orbital and suborbital launch sites of the world, https://brycetech.com/reports/report-documents/Bryce_Launch_Sites_2023.pdf.
[7] Clarke, N. et al. (2021), “Cosmonautics: The development of space-related technologies in terms of patent activity”, European Patent Office, https://documents.epo.org/projects/babylon/eponet.nsf/0/E1DF0B13D852BB7BC12586FE0049DC4A/$FILE/patent_insight_report-cosmonautics_en.pdf.
[36] CRAF (2021), Compatibility studies initiated for the radio astronomy 6.6GHz band at ITU_R working party 7D, 25 October, Committee on Radio Astronomy Frequencies, https://www.craf.eu/compatibility-studies-initiated-for-the-radio-astronomy-6-6-ghz-band-at-itu-r-working-party-7d/.
[25] ESA (2023), Satellite frequency bands, European Space Agency, https://www.esa.int/Applications/Telecommunications_Integrated_Applications/Satellite_frequency_bands.
[33] Euroconsult (2022), The use of extended C-band, planned C-band and the 7025-7075 MHz band for satellite services, Study commissioned by Ericsson, Huawei Technologies Co. Ltd. and Nokia Corporation, https://www.euroconsult-ec.com/connectivity-expertise/.
[32] Eves, S. (2021), Congested, contested... under-regulated and unplanned, issue 3(29), https://room.eu.com/article/congested-contested-under-regulated-and-unplanned.
[12] FAA (2023), Office of Spaceports website, US Federal Aviation Authority, https://www.faa.gov/space/office_spaceports.
[35] GSOA (2022), “Ensuring optimal use of the upper 6Ghz band”, 24 November, Global Satellite Operators Association, https://gsoasatellite.com/news/2619/#_ftn2.
[26] Holmes, M. (2008), Hot Orbital Slots: Is There Anything Left?, 1 March, https://www.satellitetoday.com/uncategorized/2008/03/01/hot-orbital-slots-is-there-anything-left/.
[18] Inoue, K. and Y. Shiga (2023), “Southeast Asia’s space race chases wins in tourism, communications”, Nikkei Asia, 11 May, https://asia.nikkei.com/Business/Aerospace-Defense-Industries/Southeast-Asia-s-space-race-chases-wins-in-tourism-communications.
[38] ispace (2022), ispace Receives License to Conduct Business Activity on the Moon from Japanese Government, 8 November, https://ispace-inc.com/news-en/?p=3829.
[16] ISRO (2023), Annual Report 2022-23, Indian Space Research Organisation, https://www.isro.gov.in/media_isro/pdf/AnnualReport/Annual_Report_2022_23_Eng.pdf.
[24] ITU (2023), WRS-22: Regulation of satellites in Earth’s orbit, 2 January International Telecommunication Union, https://www.itu.int/hub/2023/01/satellite-regulation-leo-geo-wrs/.
[31] ITU (2022), ITU Radiocommunication Bureau (BR) 2021 Annual Space Services Report to the STSC 2022 Session on the use of the Geostationary-Satellite Orbit (GSO) and other orbits, International Telecommunication Union, https://www.itu.int/en/ITU-R/space/snl/SNLReport/SNS-ref-list-2021_e.pdf.
[27] ITU (2019), Resolution 35 (WRC-19), International Telecommunication Union, http://www.itu.int.
[10] Jones, A. (2023), “China launch plans more than 70 launches in 2023”, in Space News, 17 January, https://spacenews.com/china-launch-plans-more-than-70-launches-in-2023/.
[15] Jones, A. (2023), New Chinese commercial spaceport to host first launch next year, 24 February, https://spacenews.com/new-chinese-commercial-spaceport-to-host-first-launch-next-year/.
[17] Kim, B. (2023), “S. Korea, Thailand sign deal to cooperate for feasibility study for space launch site”, Yonhap News Agency, 13 February, https://en.yna.co.kr/view/AEN20230213008000320.
[40] Kinder, T. (2023), “How Silicon Valley is helping the Pentagon in the AI arms race”, in Financial Times, 31 July, https://www.ft.com/content/2ed278cc-6c3f-4569-b73c-64ad378f3ea8?segmentId=b385c2ad-87ed-d8ff-aaec-0f8435cd42d9#comments-anchor.
[20] Kulu, E. (2023), Small satellite launchers, https://www.newspace.im/launchers.
[4] McClintock, B., K. Langeland and M. Spirtas (2023), First Mover Typology for the Space Domain: Building a Foundation for Future Analysis, RAND Corporation, https://doi.org/10.7249/rra2208-1.
[8] McDowell, J. (2023), Space Activities in 2022, Rev. 1.10, https://planet4589.org/space/papers/space22.pdf.
[34] Mohyeldin, E. (2022), “Society cannot cope with the growing demands on 5G without 6GHz”, 7 June 2022, Nokia website, https://www.nokia.com/blog/society-cannot-cope-with-the-growing-demands-on-5g-without-6ghz/.
[23] NAPA (2020), Space Traffic Management: Assessment of the Feasibility, Expected Effectiveness, and Funding Implications of a Transfer of Space Traffic Management Functions, Report commissioned by the US Office of Space Commerce, Department of Commerce, https://s3.us-west-2.amazonaws.com/napa-2021/studies/united-states-department-of-commerce-office-of-space-commerce/NAPA_OSC_Final_Report.pdf.
[41] OECD (2023), Competition and innovation: A Theorectical Perspective, OECD Competition Policy Roundtable Background Note, https://www.oecd.org/daf/competition/competition-and-innovation-a-theoretical-perspective-2023.pdf.
[3] OECD (2023), Harnessing “New Space” for Sustainable Growth of the Space Economy, OECD Publishing, Paris, https://doi.org/10.1787/a67b1a1c-en.
[6] OECD (2022), OECD Handbook on Measuring the Space Economy, 2nd Edition, OECD Publishing, Paris, https://doi.org/10.1787/8bfef437-en.
[39] OECD (2019), Going Digital: Shaping Policies, Improving Lives, OECD Publishing, Paris, https://doi.org/10.1787/9789264312012-en.
[9] OECD (2019), The Space Economy in Figures: How Space Contributes to the Global Economy, OECD Publishing, Paris, https://doi.org/10.1787/c5996201-en.
[22] Ogden, T. (2022), Wealthy nations are carving up space and its riches – and leaving other countries behind, 11 May, https://theconversation.com/wealthy-nations-are-carving-up-space-and-its-riches-and-leaving-other-countries-behind-182820.
[30] Purity, N. (2020), “Spectrum & Orbital Slotting – A case for African Countries”, Africanews website, 3 December, https://africanews.space/spectrum-orbital-slotting-a-case-for-african-countries/.
[29] Rainbow, J. (2023), “Speed and safety are top priorities for regulators”, Space News, 17 April, https://spacenews.com/speed-and-safety-are-top-priorities-for-regulators/.
[21] The Hindu (2022), ISRO’s Next-Gen Launch Vehicle may assume PSLV’s role, 14 October, https://www.thehindu.com/sci-tech/science/isros-next-gen-launch-vehicle-may-assume-pslvs-role/article66005152.ece.
[13] Transport Canada (2023), Government of Canada supports commercial space launches in Canada, 20 January, https://www.canada.ca/en/transport-canada/news/2023/01/government-of-canada-supports-commercial-space-launches-in-canada.html.
[5] UCS (2023), “UCS Satellite Database: 01 January 2023 update”, Union of Concerned Scientists, https://www.ucsusa.org/nuclear-weapons/space-weapons/satellite-database (accessed on 20 March 2019).
[42] UK Space Agency (2018), “Fact Sheet: The UK Space Agency’s new requirements for in-orbit third-party liability insurance”, UK Space Agency, https://assets.publishing.service.gov.uk/government/uploads/system/uploads/attachment_data/file/744408/TPL_Insurance_Fact_Sheetsw2.pdf (accessed on 30 June 2019).
[2] Undseth, M. and C. Jolly (2022), “A new landscape for space applications: Illustrations from Russia’s war of aggression against Ukraine”, OECD Science, Technology and Industry Policy Papers, No. 137, OECD Publishing, Paris, https://doi.org/10.1787/866856be-en.
[1] Undseth, M., C. Jolly and M. Olivari (2021), “Evolving public-private relations in the space sector: Lessons learned for the post-COVID-19 era”, OECD Science, Technology and Industry Policy Papers, No. 114, OECD Publishing, Paris, https://doi.org/10.1787/b4eea6d7-en.
[37] UNOOSA (1967), Resolution 2222 (XXI). Treaty on Principles Governing the Activities of States in the Exploration and Use of Outer Space, including the Moon and Other Celestial Bodies, https://www.unoosa.org/oosa/en/ourwork/spacelaw/treaties/outerspacetreaty.html.
[14] US Department of State (2019), Brazil (19-1216.1) – Agreement on Technology Safeguards Associated with U.S. Participation in Launches from the Alcantara Space Center, webpage, https://www.state.gov/brazil-19-1216.1.
[11] US Space Force (2022), Space-track.org website, Data extracted 16 December, 18th Space Defense Squadron, https://www.space-track.org.