Innovation can occur in the production, processing and recycling of plastics. This chapter first reviews the overall trends in innovation for environmentally relevant plastics technologies, before investigating the empirical link between circular economy policies and innovation. Finally, the chapter focuses on recent trends in specific technologies and the related policy implications.
Global Plastics Outlook
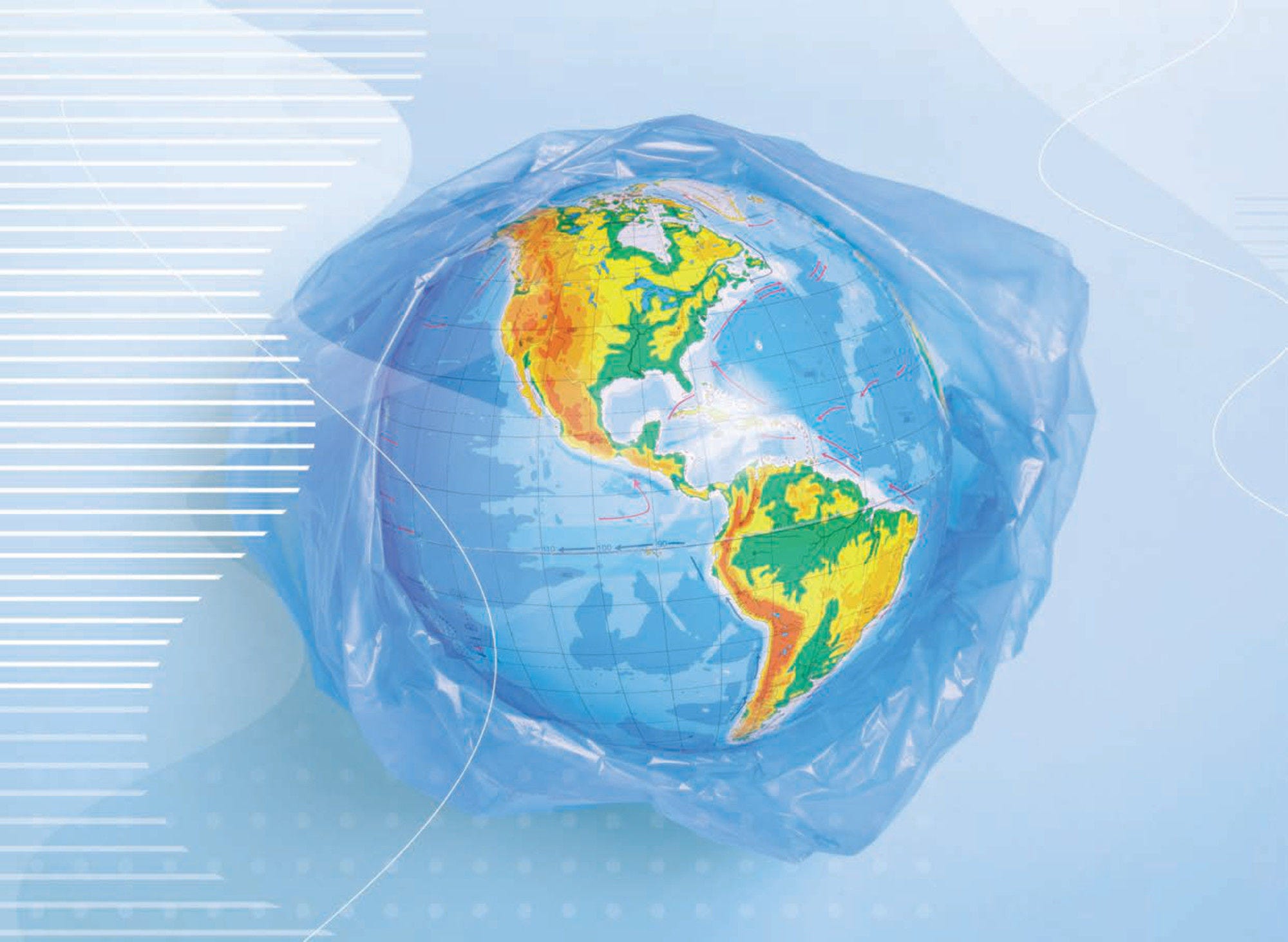
5. Innovation on plastics
Abstract
KEY MESSAGES
Innovation along the entire plastics value chain will be critical to reduce the environmental impacts of plastics. This chapter takes a novel approach to conceptualise and quantify this innovation on a global scale, drawing on patent and trademark data from the past three decades.
Innovation in environmentally relevant plastics technologies has steadily increased – the total number of patents in this field rose by a factor of 3.4 between 1990 and 2017. In addition, our analysis of trademarks, which measure low-technological innovations that are not necessarily reflected by patents, indicates that every year between 1995 and 2017 innovation for reusing plastics increased by 23%, while innovation for repairing plastics rose by 12%.
About half of all environmentally relevant innovations patented in 2017 focused on plastics circularity, i.e. on the prevention and recycling of plastic waste. One-third were related to biobased feedstock and the remainder were aimed at converting or disposing of waste as well as removing plastics leaked into the natural environment.
The focus of patenting activity is shifting away from waste prevention to waste recycling, potentially due to consumer priorities and the policy emphasis on plastics recycling.
Our quantitative evidence suggests that circular economy policies such as extended producer responsibility systems can encourage innovation in plastic waste recycling. However, innovation in plastic waste prevention and recycling remains limited, representing only 1.2% of total innovation on plastics in 2017 – largely unchanged since 1990 (1.1%). Much more ambitious policies are needed to direct technological change towards closing plastics loops and reducing leakage to the environment.
Innovation activity is concentrated in OECD countries and the People’s Republic of China, together accounting for more than 80% of all patents related to the circular use of plastics. Given the growing problems of plastic leakage in developing countries, there is a need to accelerate the international transfer of these technologies.
Environmental relevant plastics innovation is evolving rapidly. For example, innovation in biodegradable plastics grew rapidly during the last decade but has recently slowed likely due to concerns over poor biodegradation in natural environments. Plastic-to-plastic chemical recycling technologies, which aim to recycle waste that cannot be processed with mechanical recycling, are only emerging but face significant challenges. Many other innovations across the life cycle of plastics are also emerging.
Innovation will only lead to scalable solutions if the demand for circular plastics is strong. Consequently, investments in innovation should go hand in hand with education, environmental awareness, financial incentives for behavioural change and binding regulations that should be adapted to the local context.
5.1. What are the trends in environmentally relevant plastics innovation?
To answer this question, the present analysis measure environmentally relevant plastics innovation using patent and trademark data. A patent is an exclusive right granted to an inventor to use and sell his invention for a certain number of years. Patent counts are a common way to measure innovation because they capture detailed information on recent innovations of significant market value (Narin, 1995[1]). In contrast, trademarks measure low-technological innovations that are not necessarily reflected by patents.
Patent counts can be used to measure innovation in environmentally relevant plastics technologies. To do so, textual information in patent abstracts were searched in the PATSTAT database using various combinations of keywords. For example, inventions on mechanical recycling can be identified by a search string such as “shred plastic waste” or “recover polymer scrap”, while patents for plastic-to-plastic chemical recycling can be identified by words such as “hydrolysis” and “glycolysis”.
Following the database sweep, the identified inventions were scrutinised to extract the patent families related to innovation in environmentally relevant plastics technologies and were classified into three main groups (Figure 5.1):
1. Innovations for plastics prevention and recycling: “plastics prevention” is a broad term capturing not only plastic waste prevention innovations, but also the innovations for technologies or processes that will use fewer plastics in the first place – they will also be referred to as innovations for plastics circularity.
2. Innovations for converting or disposing of waste and removing leakages of plastic from the environment.
3. Innovations in biobased feedstock.
When conducting cross-country comparison, only patent families that have been granted by multiple national patent offices were counted in order to avoid the inflating the number of low-value patents.1 The analysis includes patent data from 1990 and goes up to 2017 since databases for more recent years may be incomplete due to the delay in patent registrations. Moreover, when focusing on international/regional patent families, the available years are pushed back further – from 2017 to 2014 – due to the time lag between the first patent application in a given country and the subsequent patent applications in other countries.
Figure 5.1. Classification of innovation in environmentally relevant plastics technologies
Innovation in some technologies is not necessarily captured well by patent data.2 This is the case for inventions for reusing plastics, which contain low-tech innovation and so are generally not patented; and for inventions for repairing plastics, which are more commercialised. The number of registered trademarks is a more appropriate indicator to capture innovation trends in these technologies. For example, trademarks are used to protect innovation in reusable plastic transport containers (e.g. cups); reusable plastic water bottles and distribution services for reusable plastic packing material. While reusable products may seem relatively low-tech, the logistics behind shared transport systems and containers involve innovation.
5.1.1. Environmentally relevant plastics innovation is growing but is still small scale
Innovation in environmentally relevant plastics technologies has increased rapidly over the last 30 years (Figure 5.2). The total number of patents rose by a factor of 3.4 between 1990 and 2017. Patented technologies for plastics prevention and recycling form the largest group and increased the most – by a factor four. In comparison, new technologies for biobased feedstock and for converting, disposing of or removing leakage of plastic waste rose by a factor of three.
Figure 5.2. Innovation in plastics prevention and recycling have grown the most
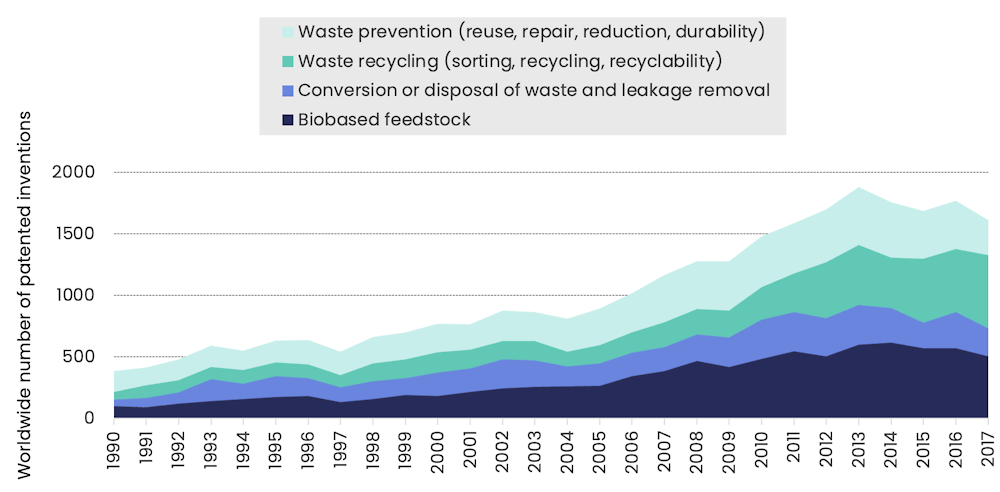
Source: Calculation based on textual analysis of data from the OECD, STI Micro-data Lab: Intellectual Property Database, http://oe.cd/ipstats, June 2020.
Between 2012 and 2017, the growth in patents for environmentally relevant plastics technologies levelled off. Since 2012, the number of new patents for prevention and recycling technologies has remained constant, while it has slightly declined for biobased feedstock technologies as well as for technologies converting or disposing of waste and removing leakages of plastics from the environment. However, public awareness of plastic waste leakage and political action to address the issue have increased following the 2015 launch of the G7 Action Plan against marine litter. Therefore, it is likely that patents will have increased since then, but the data are not yet available.
Trademarks data provide additional insights into low-tech plastics innovation. Every year between 2013 and 2017 there were on average 65 new registered trademarks related to plastics reuse technologies and 2 700 for plastics repair technologies. The number of trademarks related to plastics reuse increased by 23% every year between 1995 and 2017, while the number of trademarks related to plastics repair rose by 12% every year.
Since 2012, innovation in recycling technologies has outpaced plastic waste prevention technologies; they saw almost twice as many patents in 2017 (Figure 5.2). The shift towards recycling is probably driven by increased environmental awareness by consumers, who are willing to pay more for recycled content, and by policies such as extended producer responsibility (EPR) which make producers financially responsible for recycling waste, including packaging (Chapter 6).
Although innovation in plastic waste prevention and recycling has increased since 1990, its share in overall plastics innovation at the global level remains small. Innovation in preventing and recycling plastic waste accounted for only 1.1% of all plastics innovation in 1991 and only 1.2% in 2017. Therefore, the large-scale adoption of much stronger policies is needed to redirect innovation towards the circular use of plastics.
The remainder of this section focuses on prevention and recycling technologies, since biobased feedstock (Chapter 2) and technologies for converting or disposing of waste have been covered comprehensively in previous chapters (see Box 2.2 and Box 2.3, among others).
5.1.2. Plastics prevention and recycling innovation is concentrated in a few countries
Innovation in plastics prevention and recycling (innovation for plastics circularity) is not evenly distributed around the globe (Figure 5.3). The vast majority is concentrated in OECD countries and China. For instance, only ten countries accounted for 92% of plastic prevention and recycling patents between 2010 and 2014, with Japan, the United States, China, Korea, Germany and France alone accounting for 85%.
Figure 5.3. OECD countries and China lead on innovation in plastics circularity
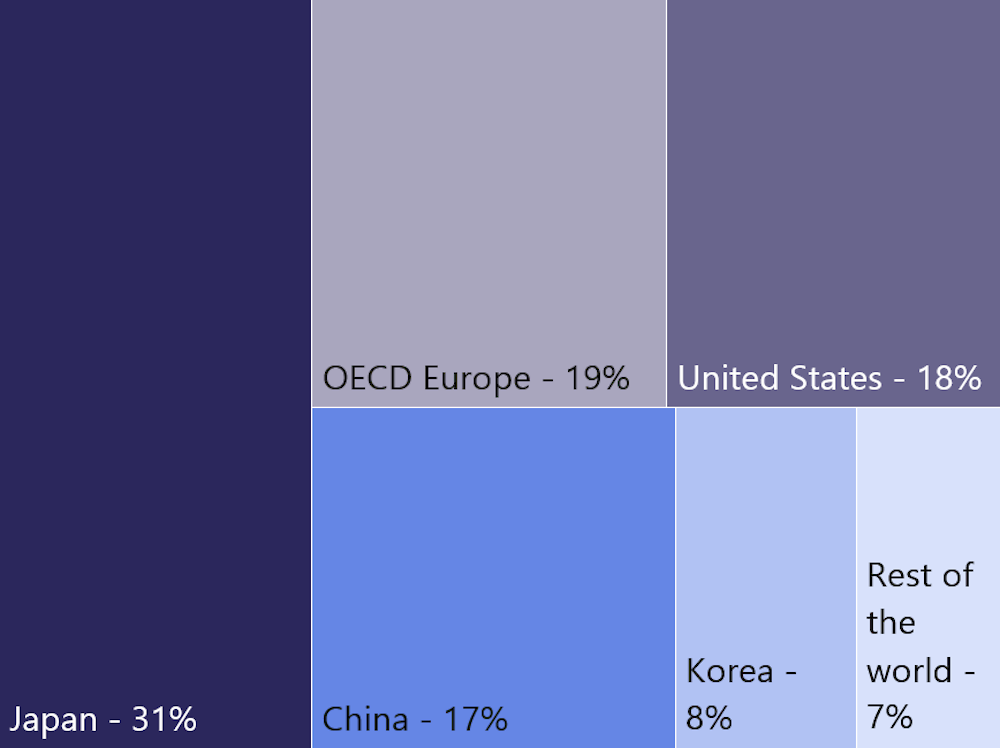
Source: Calculation based on textual analysis of data from the OECD, STI Micro-data Lab: Intellectual Property Database, http://oe.cd/ipstats, June 2020.
Figure 5.4 looks at the top ten innovating countries and compares their global share in patented inventions for plastics prevention and recycling with their global share in all plastics-related patents, environmental or otherwise. Unsurprisingly, countries that innovate in plastics in general also tend to innovate in plastics circularity technologies. Nevertheless, some countries invest relatively greater effort in plastics prevention and recycling technologies. For instance, France, Japan, Korea, the United Kingdom and United States invested a relative large share of their innovation efforts in plastics circularity between 2010 and 2014. In contrast, Germany, China and Australia were relatively less focused on plastics circularity during the same period. Nonetheless, due to their high number of plastic-related innovations, these three countries are leaders when considering the absolute number of circular plastics patents filed. In addition, the focus on circular plastics technologies is likely to increase in several countries. For example, a full waste export ban will come into effect in Australia by mid-2024 and will significantly increase the domestic demand for plastic recycling. The growing Australian market, also supported by a public investment of AUD 190 million in recycling modernisation, will likely spur innovation in plastic waste recycling.
This large concentration of plastics prevention and recycling innovations highlights the need to accelerate the international transfer of these technologies towards developing countries where plastics use and problems of plastic leakage are growing rapidly.
Figure 5.4. Some countries specialise in circular plastics innovation
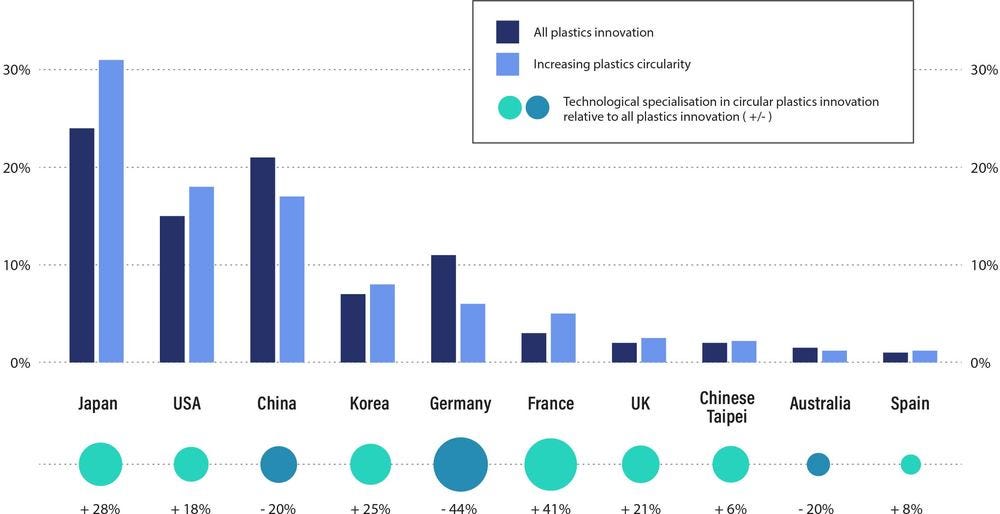
Source: Calculation based on textual analysis of data from the OECD, STI Micro-data Lab: Intellectual Property Database, http://oe.cd/ipstats, June 2020.
5.2. What role for policies in driving circular plastics innovation?
The previous section showed that there are significant differences across countries in circular plastic innovation. This section explores how circular economy policies contribute to these differences, using the case study of the German packaging ordinance – the first EPR system for the recycling and recovery of sales packaging in the world.
The 1991 packaging ordinance, replaced by the German Packaging Act (Verpackungsgesetz) in 2019, required retailers and producers to take back and recycle a fixed but annually increasing percentage of packaging materials. The take-back system was deemed to be effective as the volume of packaging materials declined by 500 000 tonnes between 1992 and 1993.
Three years after the introduction of the Packaging Ordinance, plastic recycling capacities had increased by a factor of four (OECD, 1998[3]). Qualitative evidence suggests that this significant increase in plastic recycling capacity was due to the sizeable positive impact of the ordinance on plastic recycling innovation. To meet the quotas set by the take-back system, producers had to find new processes to convert plastic waste and create new markets for secondary materials. Among the new technologies developed at the time were new recycling processes, such as those using hydrocyclones and centrifuges to separate individual plastics (OECD, 1998[3]).
To provide some quantitative evidence of the effect of the German packaging ordinance on plastic recycling innovation and go further than correlation, a synthetic control method that builds a counterfactual or control group for Germany is employed.3 This control group is composed of Canada, Japan, the Netherlands, Korea and the United States. These are all similar to Germany in terms of recycling patents but none had introduced a similar recycling policy around 1991.4 Using several predictors, patent filing in recycling technologies in Germany before the regulation is introduced is successfully replicated in the control group (Figure 5.5).5 It is found that five years after its introduction in Germany, the packaging ordinance increased the stock of recycling innovation by 190% compared to the control group for Germany in which the regulation is not introduced.6
Figure 5.5 also shows that there had already been a spurt in plastic recycling innovation between 1989 and 1991 in Germany. This cannot be fully explained by strategic patenting prior to the ordinance taking effect, since it was introduced within a very short timeframe making it difficult to anticipate for most stakeholders to anticipate. However, it could be explained by the first German Ministry of the Environment issuing a regulation in 1988 for the take back of plastic drinks packaging in reaction to the massive use of single-use polyethylene terephthalate (PET) bottles. This might explain the observed difference between Germany and the control group.
Figure 5.5. The German packaging ordinance prompted innovation in plastic recycling
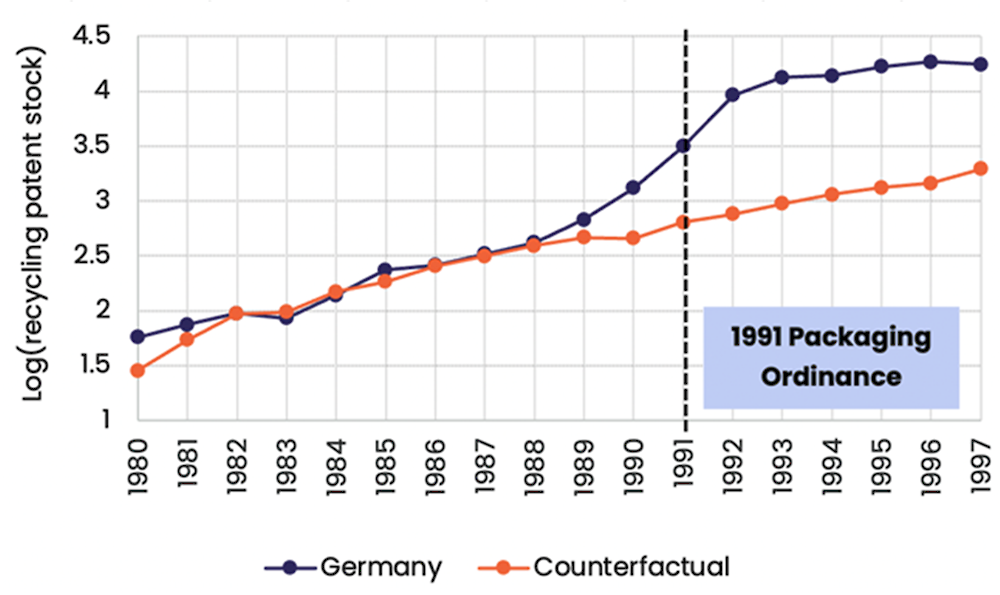
Note: The control group for Germany is composed of Canada (28%), Japan (12%), Netherlands (24%), Korea (13%) and the United States (23%). The predictors used are log (patent stock in all plastic innovation), log(GDP per capita), log(energy use per capita), log(recycling patent stock) in 1982, 1984 and 1988. An invention corresponds to a single patent family. The year of the invention corresponds to the application date of the priority patent. Only granted patents are considered. Plastic recycling patents include mechanical recycling, plastic-to-plastic chemical recycling, sorting and other pre-treatments. Data before 1990 includes only the Federal Republic of Germany (FRG).
Source: Calculation based on textual analysis of data from the OECD, STI Micro-data Lab: Intellectual Property Database, http://oe.cd/ipstats, June 2020.
This case study suggests that plastic recycling policies successfully triggered innovation in plastic recycling. However, the effect of current and future circular economy policies on the emergence of new technologies has yet to be fully explored. The next sections take a step back from these aggregated analyses to present recent trends in specific environmentally relevant plastics innovation and derive policy implications.
5.3. What are the latest innovation challenges?
5.3.1. Biodegradable plastics innovation is slowing after decades of sustained growth
Biodegradable plastics gained some popularity with the idea they would degrade in the natural environment into carbon dioxide, water and biomass.7 They are currently used for packaging, agriculture, horticulture, textiles and consumer goods. Global production capacities of biodegradable plastics was 1.2 Mt in 2019 or less than 0.3% of total plastics (Crevel, 2016[4]; European Bioplastics, 2019[5]).
Innovation in biodegradable plastics, measured by the number of patented inventions, doubled between 1995 and 2017. Between 2013 and 2017, 228 patent families for biodegradable plastics were granted every year (Dussaux and Agrawala, forthcoming[2]). However, since 2013, the speed of innovation has slowed down, probably because the environmental impact of compostable plastics has become controversial due to the issues related to biodegradation in natural environments (Box 5.1).
Box 5.1. “Biodegradable” plastics have become controversial
Microbial degradation of biodegradable polymers is not easily achieved in a natural environment (Wierckx et al., 2018[6]). For example, to biodegrade in a reasonable time, some polymers such as polylactic acid (PLA) require the temperature used in industrial composting to be above 60°C (Farah, Anderson and Langer, 2016[7]). Biodegradation also requires optimal conditions, such as the concentration of enzymes, strains of microorganisms, temperature, pH value, humidity, oxygen supply and light. These optimal conditions are rarely present in natural environments (Laycock et al., 2017[8]; Haider et al., 2019[9]).
Napper and Thompson (2019[10]) compared the deterioration of conventional polyethylene bags with bags that claimed biodegradable, oxo-degradable or compostable properties. The study included various environments, including open air, buried in soil and submersed in the marine environment. Apart from the compostable bag in the marine environment, fragments of all bags were present in all environments after 27 months. Moreover, some bags were still functional as grocery bags after three years in the soil or a marine environment. Strikingly, the “biodegradable” bags used in this experiment did not deteriorate faster than conventional polyethylene.
Although most ecotoxicity studies do not find evidence of harmful effects of degradation products (Haider et al., 2019[9]), when it comes to PLA there are two notable exceptions. Souza et al. (2013[11]) found cytotoxic and genotoxic effects on common onions and Adhikari et al. (2016[12]) found that microbial activity was inhibited after 84 days of incubation in the soil. Another concern is the use of oxo-degradable plastics that contain metal salts that act as catalysers to speed up degradation (OECD, 2013[13]; Kershaw, 2015[14]). The degradation of oxo-degradable plastics can release micro fragments of plastics and metals into the environment, but the effects on soil, water, flora and fauna are still not well understood. Several countries have already taken regulatory action on oxo-degradable plastics. For example, since 2015, France has banned bags and packaging made of oxo-degradable plastics and the European Union has prohibited products made from oxo-degradable plastic since 2019.
In addition to biodegradation issues, there are other concerns. First, some biodegradable plastics are produced from biobased polymers, which whose have mixed environmental impacts are mixed. Second, if collection and recycling conditions are not suitable, biodegradable plastics may compromise the quality of the recycled resources (Hornitschek, 2012[15]; Hann et al., 2015[16]). In addition, consumers may become confused, and put non-compostable plastics in the kitchen waste to be collected for composting. Furthermore, labelling plastics as biodegradable may suggest that littering is acceptable (Kershaw and Rochman, 2015[17]).
Despite these drawbacks, making plastics compostable can be an advantage for specific applications. For example, plastics in a restaurant can be directly composted together with all kitchen waste without further processing. Also, many different compostable plastics exist, so more innovation in this field could lead to better polymers and new applications.
5.3.2. Chemical recycling is only emerging but is facing significant challenges
Chemical recycling involves chemical processes that break down the waste polymers into feedstock for the production of fuels or into new polymers (Thiounn and Smith, 2020[18]). Chemical recycling is different from incineration, which essentially recovers heat from the combustion of plastic waste. As with mechanical recycling, sorting and pre-treatment are essential to optimise chemical recycling.
Chemical recycling can be divided in two categories (Figure 5.6). The first category is the transformation of plastic waste mainly into fuels via thermolysis, also referred to as “plastic-to-fuels” technologies. Examples of thermolysis processes include thermal cracking, pyrolysis, gasification, hydrothermal liquefaction and catalytic pressureless depolymerisation (Rahimi and García, 2017[19]).8
Figure 5.6. Chemical recycling covers a wide range of chemical processes
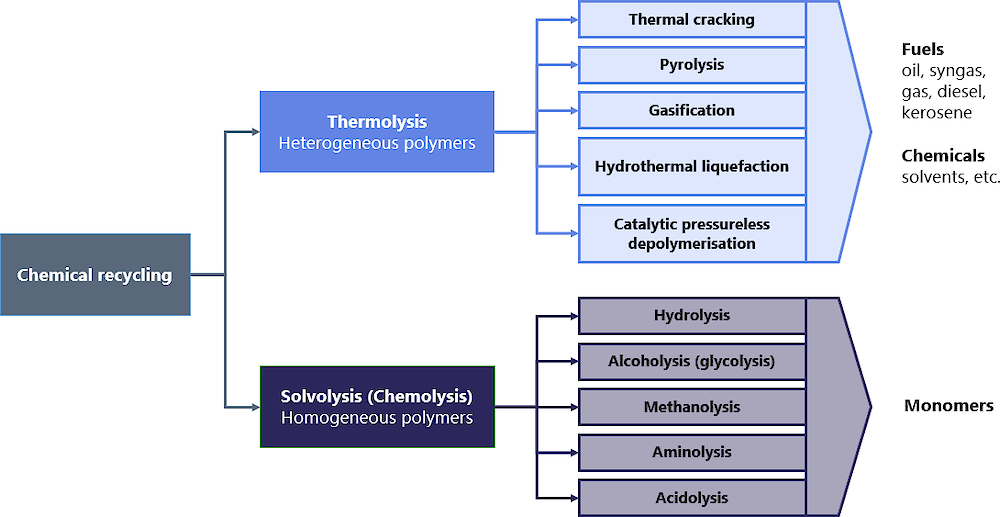
Thermolysis of plastic waste operates at high temperatures that consume a large part of the energy embodied in the plastics. For instance, pyrolysis typically occurs at 550°C and theoretically requires 1 328 megajoules (MJ) per kg of polyethylene (PE) waste to generate fuels with a calorific value of around 40 MJ per kg of waste (Gao, 2010[20]; Ripley, 2014[21]; Rollinson and Oladejo, 2019[22]). These relatively high internal energy needs and the focus on the production of fuels, instead of materials, make the term recycling controversial for most thermolysis processes.
Each thermolysis method has different environmental impacts. For example, Khoo (2019[23]) argues that gasification of mixed plastic waste requires ten times more energy but emits less nitrogen oxides (NOx), sulphur dioxide (SO2) and particulate matter (PM) than pyrolysis. However, this result is based on old references and would benefit from further research. Using life cycle analysis (LCA), Demetrious and Crossin (2019[24]) find that pyrolysis and gasification have lower environmental impacts than landfilling, but comparable environmental impacts to incineration with energy recovery. Khoo (2019[23]) shows that pyrolysis and gasification have lower impacts on climate change than incineration with energy recovery, but significantly higher impacts in terms of terrestrial acidification and particulate matter formation. In the same study, mechanical recycling is found to vastly outperform these thermolysis processes in terms of environmental impacts.
The second category of chemical recycling technologies is called solvolysis or “plastic-to-plastic” chemical recycling. In this process, plastic waste is converted to monomers as feedstock for new polymers (Figure 5.6). These chemical depolymerisation technologies include hydrolysis, alcoholysis, methanolysis, glycolysis and aminolysis (BCG, 2019[25]; Das and Pandey, 2007[26]). Plastic-to-plastic chemical recycling is less controversial than thermolysis because it can potentially reduce the demand for new virgin polymers. Solvolysis or plastic-to-plastic chemical recycling aims to overcome the principal obstacle faced by mechanical recycling (downcycling9) by yielding polymers that are identical to the originals.
Plastic-to-plastic technologies are only just emerging and are less mature than the “plastic-to-fuels” conversion techniques. Between 1995 and 2017, innovation in plastic-to-plastic chemical recycling as measured by patents only increased by 5.2% every year (Dussaux and Agrawala, forthcoming[2]). This is not surprising given that plastic-to-plastic chemical recycling has only attracted interest recently, so the patent data are not yet fully available. In interviews for this study, market actors indicated that they expect the global capacity of pilot and demonstration plants for “plastic-to-plastic” to grow to at least 140 kilotonnes of waste per year in 2022.10
The environmental impact of solvolysis depends on the polymer, the method used and local elements such as the energy mix of for electricity production. One study found that solvolysis of expanded polystyrene (PS) saves 3.3 tonnes of carbon dioxide equivalent (CO2e) per tonne of input compared to incineration, which is currently the main route for expanded PS waste (CE Delft, 2019[27]). Conversely, De Andrade et al. (2016[28]) showed that mechanical recycling of PLA performs better than chemical recycling via hydrolysis and re-polymerisation, in terms of climate change, human toxicity and fossil fuel depletion. Mechanical recycling uses 2 649 kJ of electricity per kg of residual PLA, while solvolysis uses 11 211 kJ. However, a Dutch study found that mechanical recycling of expanded PS has the same climate impact as solvolysis of expanded PS (Netherlands Institute for Sustainable Packaging, 2018[29]). Another LCA study found that mechanical recycling of PET trays has lower greenhouse gas emissions than magnetic depolymerisation,11 but that the difference is not high and limited to 0.7 tonnes of CO2e per tonne of input (CE Delft, 2019[27]).
However, the results of these LCA studies should be treated with great caution. The environmental impact of “plastic-to-plastic” recycling is far from being fully understood. A recent review found that it is complicated to acquire data on environmental impacts of chemical recycling that are of good quality due to the limited maturity of the solvolysis concept on a commercial scale (Tabrizi et al., 2020[30]). In addition, some stakeholders warn against overly enthusiastic reactions to chemical recycling as it could reinforce the acceptance of single-use plastics, thereby delaying the development of waste prevention technologies (Tabrizi et al., 2020[30]).
5.3.3. Myriad innovations along the value chain of plastics are emerging
In addition to plastic-to-plastic chemical recycling, there are many other emerging innovations that aim to address the environmental issues associated with plastics. Some notable examples include:
Machine learning to sort plastic waste: To achieve a high level of purity, advanced techniques for sorting plastic waste now involve “deep learning”, in which a machine is trained to recognise different types of plastics and other materials. For example, machine learning is currently applied to sort plastic waste from electrical and electronic equipment, such as acrylonitrile butadiene styrene (ABS), high impact polystyrene (HIPS), polypropylene and crystal polystyrene. It is especially useful for sorting dark plastics that are difficult to identify using near infrared techniques due to the absorption of radiation. As computation power keeps increasing, many new algorithms and applications will be unlocked in the future (Tarun, Sreelakshmi and Peeyush, 2019[31]).
Blockchain tools to foster recycling: The uncertainty about the quality of sorted plastics is a barrier for plastics manufacturers (Chidepatil et al., 2020[32]). Blockchain is a digital technology that allows detailed tracking and the verification of information. Blockchain can make the information required by plastics manufacturers accessible when sorting is combined with multi-sensor-driven artificial intelligence and when a large share of actors in the supply chain are willing to collaborate. For example, Radical Innovations Group has developed a blockchain platform with smart contracts that contain validated data on the waste source, type, colour, quantity, origin and sorting process.
Filter washing bags to reduce microplastics release at source: Plastics or other textiles can be put into the Guppyfriend Washing Bag to reduce microfibre shedding during washing.12 After washing, the microfibres captured in the bag can be removed and disposed of in the residual waste bin. The laundry bag is produced from a plastic woven polyamide and is designed to maximise user-friendliness.
Autonomous leakage removal systems in rivers and oceans: the Ocean Cleanup Interceptor 2.0 contains barriers that guide floating plastic waste to a vessel that collects the waste. The aim is to capture leaked plastics in rivers before they enter the ocean.13 The vessel is solar-powered, works autonomously and can store up to 50 cubic metres of trash.
New delivery models: Innovation is about more than new technologies. Loop is an example of an organisational innovation in which the business model of a delivery service is changed to avoid single-use packaging and to reduce the overall environmental footprint.14 Loop delivers online orders to households in reusable containers that will afterwards be collected, cleaned and refilled.
5.4. How to transition from innovation to scalability?
With so many technologies to choose from, the question arises as to which ones should be developed and deployed more widely to address the environmental problems caused by the production and use of plastics. The usefulness of a technology depends strongly on the context of a country. For instance, massive deployment of proprietary filtration systems might not be cost effective for countries that have centralised wastewater treatment plants (WWTP) capable of capturing microplastics from wastewater installed all over the country.15 They may, however, be effective for other countries. In addition, capturing plastic debris from rivers makes more sense in countries where mismanaged waste is a major issue.
Innovation in products, processes and business models is essential, but is only part of what is needed. In order to prioritise technologies for a more circular use of plastics and to give compelling incentives to consumers and entrepreneurs, a comprehensive set of policies is required, including regulations, education and information campaigns to drive behavioural changes. Innovations will only be scaled up if there is strong market demand. Using regulatory obligations and financial incentives, policy makers can set market conditions that enhance the uptake of circular technologies in consumption and production patterns. Moreover, upstream policies will be needed to restrain the overall consumption of materials. These policy issues are the topic of the next chapter.
References
[12] Adhikari, D. et al. (2016), “Degradation of bioplastics in soil and their degradation effects on environmental microorganisms”, Journal of Agricultural Chemistry and Environment, Vol. 5/1, p. 23.
[34] Aguiar, A. et al. (2019), “The GTAP Data Base: Version 10”, Journal of Global Economic Analysis, Vol. 4/1, pp. 1-27, https://doi.org/10.21642/jgea.040101af.
[25] BCG (2019), A Circular SOlution to Plastic Waste.
[36] Benavides, P. et al. (2018), “Exploring Comparative Energy and Environmental Benefits of Virgin, Recycled, and Bio-Derived PET Bottles”, ACS Sustainable Chemistry & Engineering, Vol. 6/8, pp. 9725-9733, https://doi.org/10.1021/acssuschemeng.8b00750.
[41] Bown, C. (2020), COVID-19: China’s exports of medical supplies provide a ray of hope, Peterson Institute for International Economics, https://www.piie.com/blogs/trade-and-investment-policy-watch/covid-19-chinas-exports-medical-supplies-provide-ray-hope.
[27] CE Delft (2019), “Exploratory study on chemical recycling. Update 2019. What are the opportunities for climate policy, now in the future?”.
[33] Chateau, J., R. Dellink and E. Lanzi (2014), “An Overview of the OECD ENV-Linkages Model: Version 3”, OECD Environment Working Papers, No. 65, OECD Publishing, Paris, http://dx.doi.org/10.1787/5jz2qck2b2vd-en.
[32] Chidepatil, A. et al. (2020), “From Trash to Cash: How Blockchain and Multi-Sensor-Driven Artificial Intelligence Can Transform Circular Economy of Plastic Waste?”, Administrative Sciences, Vol. 10/2.
[4] Crevel, R. (2016), “Bio-based food packaging in Sustainable Development: Challenges and opportunities to utilize biomassresidues from agriculture and forestry as a feedstock for bio-based food packaging”, FAO.
[26] Das, S. and S. Pandey (2007), “Pyrolysis and catalytic cracking of municipal plastic waste for recovery of gasoline range hydrocarbons”, Doctoral dissertation, National Institute of Technology Rourkela.
[28] De Andrade, M. et al. (2016), “Life cycle assessment of poly (lactic acid)(PLA): comparison between chemical recycling, mechanical recycling and composting.”, Journal of Polymers and the Environment, Vol. 24/4, pp. 372-384.
[24] Demetrious, A. and E. Crossin (2019), “Life cycle assessment of paper and plastic packaging waste in landfill, incineration, and gasification-pyrolysis”, Journal of Material Cycles and Waste Management, Vol. 21/4, pp. 850-860.
[2] Dussaux, D. and S. Agrawala (forthcoming), “Quantifying innovation for a circular plastic economy”, Forthcoming OECD ENV Working Paper.
[5] European Bioplastics (2019), Bioplastics market data 2019 - Global production capacities of bioplastics 2019-2024.
[7] Farah, S., D. Anderson and R. Langer (2016), “Physical and mechanical properties of PLA, and their functions in widespread applications—A comprehensive review.”, Advanced drug delivery reviews, Vol. 107, pp. 367-392.
[20] Gao, F. (2010), “Pyrolysis of Waste Plastics into Fuels”, A Thesis, University of Canterbury..
[9] Haider, T. et al. (2019), “Plastics of the future? The impact of biodegradable polymers on the environment and on society”, Angewandte Chemie International Edition, Vol. 58/1, pp. 50-62.
[16] Hann, S. et al. (2015), “The Impact of the Use of “Oxo-degradable” Plastic on the Environment”, Final Report for the European Commission DG Environment. Project conducted under Framework Contract No ENV.A.2/FRA/2015/0008and 07.0201/2016/748104/ETU/ENV.B.3.
[15] Hornitschek, B. (2012), “Impact of degradable and oxydegradable plastics carrier bags on mechanical recycling”, Report by the Transfer Center for Polymer Technology (TCKT) on behalf of the European Plastic Converters (EuPC), p. 22.
[14] Kershaw, P. (2015), “Biodegradable Plastics & Marine Litter: Misconceptions, Concerns and Impacts on Marine Environments”, UNEP GPA.
[17] Kershaw, P. and C. Rochman (2015), “Sources, fate and effects of microplastics in the marine environment: a global assessment.”, Joint Group of Experts on the Scientific Aspects of Marine Environmental Protection (GESAMP), Vol. 93.
[23] Khoo, H. (2019), “LCA of plastic waste recovery into recycled materials, energy and fuels in Singapore”, Resources, Conservation and Recycling, Vol. 145, pp. 67-77.
[45] Law, K. et al. (2020), “The United States’ contribution of plastic waste to land and ocean”, Science Advances, Vol. 6/44, https://doi.org/10.1126/sciadv.abd0288.
[8] Laycock, B. et al. (2017), “Lifetime prediction of biodegradable polymers”, Progress in Polymer Science, Vol. 71, pp. 144-189.
[44] Merrington, A. (2017), “Recycling of Plastics”, in Applied Plastics Engineering Handbook: Processing, Materials, and Applications: Second Edition, Elsevier Inc., https://doi.org/10.1016/B978-0-323-39040-8.00009-2.
[10] Napper, I. and R. Thompson (2019), “Environmental deterioration of biodegradable, oxo-biodegradable, compostable, and conventional plastic carrier bags in the sea, soil, and open-air over a 3-year period”, Environmental science & technology, Vol. 53/9, p. 47.
[1] Narin, F. (1995), “Patents as indicators for the evaluation of industrial research output”, Scientometrics, Vol. 34/3, pp. 489-496.
[29] Netherlands Institute for Sustainable Packaging (2018), “Chemical recycling of plastic packaging materials. Analysis and opportunities for upscaling”.
[38] OECD (2021), Trends of ODA for a sustainable ocean economy, OECD, Paris, https://oecd-main.shinyapps.io/ocean/.
[37] OECD (2018), Improving Markets for Recycled Plastics: Trends, Prospects and Policy Responses, OECD Publishing, Paris, https://dx.doi.org/10.1787/9789264301016-en.
[13] OECD (2013), “Policies for Bioplastics in the Context of a Bioeconomy”, OECD Science, Technology and Industry Policy Papers, No. 10, OECD Publishing, Paris, https://dx.doi.org/10.1787/5k3xpf9rrw6d-en.
[3] OECD (1998), “Extended Producer Responsibility Phase 2: Case study on the German packaging ordinance”.
[46] OECD (n.d.), Intellectual Property Database, https://www.oecd.org/sti/intellectual-property-statistics-and-analysis.htm (accessed on 1 June 2020).
[19] Rahimi, A. and J. García (2017), “Chemical recycling of waste plastics for new materials production”, Nature Reviews Chemistry, Vol. 1/6, pp. 1-11.
[39] Ren, D. (2020), China boosts face mask production capacity by 450 per cent in a month, threatening a glut scenario, South China Morning Post, https://www.scmp.com/business/companies/article/3075289/china-boosts-face-mask-production-capacity-450-cent-month.
[21] Ripley, J. (2014), “Routine Caol and Coke Analysis: Collection, Interpretation, and Use of Analytical Data MNL57-2ND (2nd Edn), Chapter 5: Proximate Analysis”, ASTM International, West Conshohocken, pp. 29-48.
[22] Rollinson, A. and J. Oladejo (2019), “‘Patented blunderings’, efficiency awareness, and self-sustainability claims in the pyrolysis energy from waste sector”, Resources, Conservation and Recycling, Vol. 141, pp. 233-242.
[40] SCIO (2020), Fighting COVID-19: China in Action, The State Council, The People’s Republic of China, http://english.scio.gov.cn/whitepapers/2020-06/07/content_76135269.htm.
[11] Souza, P. et al. (2013), “PLA and organoclays nanocomposites: degradation process and evaluation of ecotoxicity using Allium cepa as test organism”, Journal of Polymers and the Environment, Vol. 21/4, pp. 1052-1063.
[35] Stefanini, R. et al. (2020), “Plastic or glass: a new environmental assessment with a marine litter indicator for the comparison of pasteurized milk bottles”, The International Journal of Life Cycle Assessment, Vol. 26/4, pp. 767-784, https://doi.org/10.1007/s11367-020-01804-x.
[42] Subramanian, S. (2020), How the face mask became the world’s most coveted commodity, The Guardian, https://www.theguardian.com/world/2020/apr/28/face-masks-coveted-commodity-coronavirus-pandemic.
[30] Tabrizi, A. et al. (2020), “Understanding the Environmental Impacts of Chemical Recycling – ten concerns with existing life cycle assessments”, Zero Waste Europe, Brussels.
[31] Tarun, K., K. Sreelakshmi and K. Peeyush (2019), “Segregation of Plastic and Non-plastic Waste using Convolutional Neural Network”, IOP Conference Series: Materials Science and Engineering, Vol. 561/1, p. 012113.
[18] Thiounn, T. and R. Smith (2020), “Advances and approaches for chemical recycling of plastic waste”, Journal of Polymer Science, Vol. 58/10, pp. 1347-1364.
[43] Wang, C. et al. (2020), “Structure of the global plastic waste trade network and the impact of China’s import Ban”, Resources, Conservation and Recycling, Vol. 153, https://doi.org/10.1016/j.resconrec.2019.104591.
[6] Wierckx, N. et al. (2018), “Plastic biodegradation: Challenges and opportunities”, Consequences of Microbial Interactions with Hydrocarbons, Oils, and Lipids Biodegradation and Bioremediation, pp. 1-29.
Notes
← 1. A patent family is a collection of patent applications covering the same or similar technical content. See Dussaux and Agrawala (forthcoming[2]) for more information on the methodology and on the advantages and drawbacks of patent data.
← 2. However, use of patent data remains the favoured option since it captures all categories of innovation; trademark data can only recover innovation in a limited number of fields.
← 3. See Dussaux and Agrawala (forthcoming[2]) for more details on the methodology.
← 4. We exclude Austria and France because they introduced an EPR scheme for packaging in 1993, and we exclude Sweden as it introduced one in 1994.
← 5. The predictors used are log (patent stock in all plastic innovation), log (GDP per capita), log (energy use per capita), log (recycling patent stock) in 1982, 1984 and 1988.
← 6. The predictors are well balanced between Germany and the control group. The result is robust when backdating the treatment five years in advance. Permutation distribution of the placebo indicates that the increase is significantly different to zero. The result is also robust when excluding all donor countries separately (leave-one-out) except Canada. A similar analysis shows that the packaging ordinance had no statistically significant effect on all plastic innovation. See Dussaux and Agrawala (forthcoming[2]) for more details.
← 7. A European standard, EN 13432, defines biodegradability as a biodegradation level of at least 90% that must be reached in less than six months. For disintegrability, the mass of test material residues greater than 2 mm must be less than 10% of the initial mass.
← 8. Catalytic pressureless depolymerisation is also known as the Katalytische Drucklose Verölung (KDV) process.
← 9. Downcycling occurs during mechanical recycling when contaminated plastic waste is melted into a new raw material the properties of which are poorer than the original plastic products. For example, the plastic waste from a jug of milk is not used again for the production of a new jug of milk but can only be used for less demanding applications such as a layer of a detergent packaging.
← 10. As not all actors were interviewed, this estimate should be taken with caution.
← 11. Magnetic depolymerisation is a technology developed by the Dutch start-up Ioniqa, and consists of chemically depolymerising PET under the influence of a magnetic liquid. Magnetic depolymerisation of PET removes various dyes and contaminants from PET waste and produces virgin equivalent BHET that can used as raw material for PET production (https://ioniqa.com/).
← 12. Guppyfriend was registered as a EUIPO trademark in December 2018 and as a USPTO trademark in May 2020. An EPO patent was granted in November 2019. Patents were also filed in Japan and in the United States.
← 13. The Interceptor was registered as a EUIPO trademark in April 2019.
← 14. For details see https://loopstore.com/how-it-works.
← 15. Nevertheless, the management of microplastics captured in WWTP sludges that are mostly used for agricultural land is still problematic (see Chapter 2).