The health and productivity of fish stocks are key determinants of fisheries performance. Sustainably managing fish stocks is necessary to achieve the socio-economic objectives governments and stakeholders have for fisheries. This chapter analyses the health and productivity of assessed fish stocks in 32 countries and economies and discusses how commercially important fisheries are managed. It provides a reliable approximation of the status of fish stocks at the global level and detailed information at the country level, to better target management action. The chapter closes with a special focus on how to address ghost fishing gear, that is gear lost, abandoned, or otherwise discarded at sea, which can have a significant detrimental impact on fish stock health and the ocean more generally.
OECD Review of Fisheries 2022
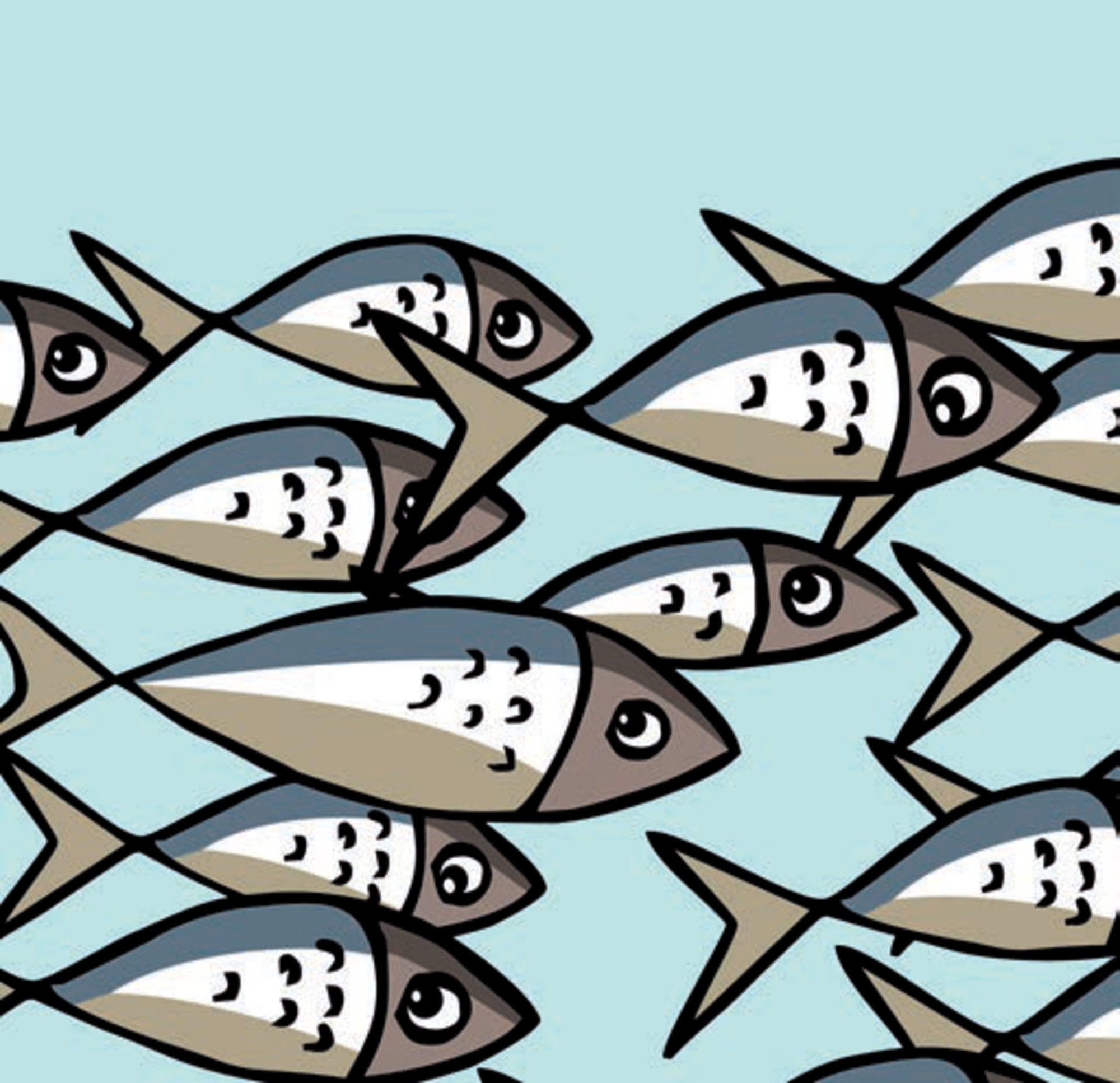
2. Managing fisheries
Abstract
Key messages and recommendations
The health of fish stocks is one of the main determinants of fisheries performance. Sustainably managing fish stocks is necessary to achieve the socio-economic objectives governments and stakeholders have for fisheries. According to the most recent stock assessments undertaken in the 32 countries and economies covered in this chapter, 64% of assessed stocks are in good health, 18% fall below sustainability standards, and for another 18%, assessments are not conclusive (and their health status remains undetermined).
Often, governments and stakeholders not only aim to ensure the sustainability of fish stocks, but also pursue higher management standards, for example optimising stock productivity by maximising the value or the volume of fish catches under sustainability constraints. Recent stock assessments tell us that just under half of the stocks that are in good health also meet higher management standards.
Effective fisheries management is key for maintaining fish stock heath and optimising their productivity. Data collected for this chapter on how commercially important fisheries are managed show that fisheries management typically involves a range of measures to control how much fish is caught and how, when and where it is caught. Management also varies considerably across fisheries. In 2021, about three quarters of the fish stocks making up the most valuable species for the countries and economies covered in this chapter were managed with limits to total allowable catch (TAC), that is, caps on the amount of fish that can be harvested. TACs are believed to be one of the most important tools for ensuring the health of fish stocks. In 2020, species covered entirely by TACs accounted for USD 9.2 billion in landings, or 61% of the value of landing for all the species in the data set. This equates to 12.6 million tonnes of fish, or 81% of all these landings by volume.
Governments should rebuild the 18% of stocks which fall below sustainability standards. This is needed to ensure their long-term health and will also improve their productivity and economic returns in the fisheries sector. Where rebuilding plans have not already been adopted, fisheries managers should consider reviewing their current management action to help rebuild stocks. Going further and ensuring the stocks already in good health are also fished optimally to maximise value or harvest volume will also lead to economic gains.
In addition, governments should invest in stock assessments for the stocks that remain unassessed. In some countries, this is still the case for many harvested and commercially important stocks. Investing in understanding the status of the 18% of assessed stocks with undetermined status, particularly where the species are of significant commercial importance (e.g. Norway lobster), would also likely improve fisheries’ sustainability and increase economic returns where overfishing is occurring but has not been detected or where stocks are underfished. Developing methods to assess stocks even where data are scarce and capacity limited will become increasingly important to inform adaptive management, as climate change impacts fish abundance and the location of stocks.
Finally, investing in linking information on stock management and stock health would help governments understand better where management is effective, optimise fisheries management plans, and, ultimately, improve the health and productivity of fish stocks further. To facilitate such analysis, governments should consider adopting an internationally agreed-upon naming convention for reporting information on stocks, which could include the use of ASFIS (Aquatic Sciences and Fisheries Information System) species codes where possible. Consistent stock naming is especially important for shared stocks, which are likely to increase in numbers due to climate change.
2.1. Benefits of healthy fish stocks and good fisheries management
Good fisheries management is fundamental for the long-term economic, social and environmental sustainability of the fisheries sector and its resilience to shocks. It is also important for protecting biodiversity and ensuring the provision of ocean ecosystem services such as climate regulation, food provision and nutrient cycling (Barbier, 2017[1]). The good news is that fisheries management generally works. Increasing management intensity is correlated with increasing biomass and reduced fishing pressure, suggesting that management action can effectively help maintain stock health (Hilborn et al., 2020[2]). Further, improving stock health can lead to significant gains in profitability for fisheries, improving the economic and social sustainability of the sector (Costello et al., 2016[3]).
Climate change, however, is driving change in marine ecosystems, becoming an increasingly important context in framing management decisions (Box 2.1). To be effective, fisheries management must adapt to the impacts of climate change on species diversity, abundance and distribution, which may require changes to the way fisheries are managed and the institutions that manage them (FAO, 2021[4]). Understanding the status of fish stocks and how to manage them effectively is, therefore, more important than ever.
The international community has recognised the importance of good fisheries management in promoting healthy fish stocks and ocean ecosystems as demonstrated through the Sustainable Development Goals (SDGs) and the Aichi Biodiversity Targets. Notably, SDG Target 14.4 calls for restoring fish stocks in the shortest time feasible, at least to levels that can produce maximum sustainable yield (MSY), and through Aichi Target 6, which aims to keep the impacts of fisheries on stocks, species and ecosystems within safe ecological limits.1 Further, as a key step towards meeting these goals, members of the World Trade Organization (WTO) have recently agreed to prohibit the provision of subsidies to the fishing of overfished stocks (see Chapter 3 for more details about the WTO Agreement on Fisheries Subsidies and its implications for fisheries support).
However, the health of fish stocks has not yet improved globally despite the growing understanding that good fisheries management is essential to fishers and ocean health more generally, and despite shared ambition to put it into practice. The Food and Agriculture Organization (FAO) (2022[5]) estimates the share of overfished stocks globally to be 35.4%. This global figure, however, masks significant regional variations in stock health. In the north-east Pacific, over 80% of stocks are fished sustainably. In comparison, more than 60% of stocks are in the Mediterranean and Black Seas are overfished. These regional numbers, themselves, hide significant variations across the stocks harvested by individual countries. However, they do indicate scope for improvement in all regions.
This chapter analyses a unique data set of information on the status of individual fish stocks assessed by 32 OECD countries and emerging economies. It uses these data to produce country-level indicators which not only inform about fish stock health but also their productivity. These indicators add further nuance to global and regional trends and help identify priorities for action.
The chapter starts by providing an overview of the health and productivity of assessed fish stocks and how the situation has evolved since the 2020 edition of the OECD Review of Fisheries (Section 2.2). It also illustrates the extent of available data on stock status and identifies where further investment in data collection and research is needed to inform science-based sustainable management.
To help governments achieve sustainable fisheries through effective management, this chapter then complements data on the status of fish stocks with data on the use of management tools. Based on these data, Section 2.3 describes the mix of tools governments are using to manage individual stocks of their most commercially valuable species. Section 2.4 then links management to stock status for a specific subset of stocks (combining information for those stocks for which both management and stock status were known and could be reconciled). Fisheries management is complex and stock health is impacted by a number of other factors (e.g. climate change), hence causal linkages between management and stock health are difficult to identify. However, it is hoped these data can begin to answer fundamental questions in fisheries management related to the effectiveness of management approaches and do so with increasing effectiveness as the combined data set develops over time.
Finally, to help governments comprehensively manage fisheries’ impact on resources and ecosystems, Section 2.5 addresses the issue of ghost fishing gear, that is, gear lost, abandoned or otherwise discarded at sea, which can account for a significant detrimental impact. The section presents the key findings of a recent OECD report, “Towards G7 action to combat ghost fishing gear” (OECD, 2021[6]), prepared in support of the UK presidency of the G7. The report highlights best practices for addressing ghost gear.
Box 2.1. Climate change and fisheries management
Impacts of climate change on fisheries
Climate change is having significant impacts on fisheries (Cheung, Watson and Pauly, 2013[7]; Barange et al., 2018[8]). Importantly, increasing ocean temperatures are expected to result in a reduction in primary productivity from the ocean (Tittensor et al., 2021[9]) and a large-scale redistribution of fisheries resources (Cheung et al., 2010[10]). These impacts, combined with changes in species composition and abundance, will impact fisheries and the communities which rely on them. The redistribution of fisheries resources will be uneven, with higher latitude regions expected to see an increase in catch potential and tropical regions a decrease of around 40% (Cheung et al., 2010[10]). Further, by 2030, 23% of transboundary stocks will have shifted, impacting 75% of the world’s economic exclusive zones (EEZs) and posing challenges for fisheries governance in many regions (Palacios‐Abrantes et al., 2022[11]). Ensuring fisheries can both mitigate greenhouse gas (GHG) emissions and adapt to the impacts of climate change are key challenges facing fisheries managers.
How fisheries management can mitigate greenhouse gas emissions
The contribution of capture fisheries to global emissions is relatively modest compared to its contribution to food security. Estimates put the total global GHG emissions from capture fisheries at 179 tonnes of carbon dioxide equivalent (t CO2-eq) in 2011, which equates to 4% of GHG emissions from food production in the same year (Parker et al., 2018[12]) and 207 t CO2-eq in 2016 (using a slightly different methodology) (Greer et al., 2019[13]). Generally, the emission intensity of production (t CO2-eq per tonne landed) for capture fisheries is lower than that of other animal products (except chicken) (Gephart et al., 2021[14]) and their nutritional value higher (Bianchi et al., 2022[15]). However, the emissions intensity of production increased by 21% between 1990 and 2011 (Parker et al., 2018[12]), leading to an increase in emissions from fisheries despite catches remaining relatively stable in the same period (OECD/FAO, 2022[16]). Since 1950, the emission intensity of production in small-scale fisheries has more than doubled but remains 10% lower than in industrial fisheries (Greer et al., 2019[13]).
Global figures for emissions and emissions intensity obscure important regional and local trends. In some cases, the increases are significantly more pronounced; for example, the emissions intensity of production increased 530% between 1966 and 1988 in New Bedford, Massachusetts (Mitchell and Cleveland, 1993[17]). The vast majority of emissions from capture fisheries are produced by burning fossil fuels on vessels and fuel is often the largest cost associated with fishing. Thus, reducing emissions from fisheries would benefit both the environment and fishers through reduced costs and enhanced profitability.
Both theoretical and empirical research shows that rebuilding fish stocks and removing excess fishing capacity through good fisheries management to maximise the catch per unit of effort (CPUE) is the most effective method for reducing emissions from fisheries (e.g. (Parker et al., 2015[18]; Waldo and Paulrud, 2016[19]; Driscoll and Tyedmers, 2010[20]). In simple terms, ensuring fishers can fish less for the same result will increase profitability and reduce emissions more effectively than technological interventions. Conversely, much of the growth in emissions from fisheries is driven by overcapacity, leading to lower CPUE. Management approaches, such as individual transferable quotas (ITQs), that incentivise the efficient allocation of inputs, can significantly reduce GHG emissions (Ziegler et al., 2016[21]). For example, in demersal fisheries in Iceland, the emissions per unit of catch fell by 40% between 1997 and 2008 after the introduction of ITQs (Kristofersson, Gunnlaugsson and Valtysson, 2021[22]). In this case, the rebuilding of stocks was a much more important driver of emissions reduction than technology improvement, oil price and fish price (Kristofersson, Gunnlaugsson and Valtysson, 2021[22]). The application of ITQs in more fisheries will likely lead to lower emissions (Waldo and Paulrud, 2016[19]).
In well-managed fisheries, where there is no excess capacity and CPUE is maximised, other interventions can help reduce emissions. For example, active gears are more emissions intensive than passive gears; hence, behavioral adaption can reduce emissions through changes in gear usage. Technology innovation also has the potential to reduce emissions in well-managed fisheries by increasing fuel efficiency. However, care is needed to avoid rebound effects whereby reduced fuel costs from increased efficiency lead to increased effort. Alternative fuel sources, such as liquid petroleum gas and hybrid propulsion or full electrification can also play a role reducing emissions (Gabrielli and Jafarzadeh, 2020[23]).
Challenges for fisheries management to adapt to climate change
The impacts of climate change are ongoing and likely to get worse in the coming years. Despite global studies indicating the magnitude of the impacts, there remains significant uncertainty at a local level (Tittensor et al., 2021[9]). Better data on the impacts of climate change on fisheries resources at local levels is the first priority for fisheries managers. Without accurate and recent information on fisheries resources, management plans risk becoming disconnected from the resources they are trying to manage. Several countries, such as the United States through its Climate and Fisheries Initiative, and Canada through its Aquatic Climate Change Science Program, are already investing in research to provide better data and information to fisheries managers on the impacts of climate change.
The next key challenge for fisheries managers is to ensure they can use the data effectively in management systems to create so-called climate-smart fisheries. The FAO (2021[4]) defines the four foundations of climate-smart fisheries as: 1) establishing effective fisheries management systems; 2) ensuring stakeholder participation in management systems; 3) taking a precautionary approach that allows for uncertainty and risk; and 4) ensuring management systems are adaptive to change. Adaptive fisheries management systems are particularly important under climate change if managers are to protect existing resources and take advantage of new opportunities that might occur as stocks move. In particular, the movement of transboundary stocks into new areas and the occurrence of new transboundary stocks could be problematic if existing collaborative management systems are not flexible enough to allow the inclusion of new stakeholders. In such cases, the reform of existing institutions may be required to ensure collaborative sustainable management of stocks and avoid resource conflicts. Regions where there are multiple EEZs in close proximity (such as the Caribbean) are likely to face greater issues than elsewhere and care must be taken to ensure the management of transboundary stocks continues to safeguard the sustainability of resources (Palacios‐Abrantes et al., 2022[11]).
2.2. The status of assessed fish stocks
Assessing the status of fish stocks is essential for good fisheries management. Setting appropriate harvesting levels requires information on how fishing practices impact the size and health of commercially important stocks. Without good data to guide fisheries management, the harvesting of stocks is likely to be suboptimal in terms of the sector’s economic, social and environmental sustainability. In extreme cases, overfishing can lead to severe declines in stock abundance, with devastating impacts on local communities and ecosystems. Regular stock status assessments are also vital for fisheries management, as they allow management regimes to adapt if they are not performing as expected or in response to exogenous factors impacting fish stocks (e.g. climate change).
To implement the appropriate management, stock assessments ideally need to determine where stocks sit with respect to key limit or target reference points – which may be quantified in terms of instantaneous fishing mortality (F) or stock biomass (B).2 Limit reference points identify sustainability thresholds that should not be crossed as, beyond these, the long-term biological viability of a stock is likely to be threatened. Target reference points, on the other hand, are optimal levels to be reached, determined by the management objective for the stock. Indeed, good fisheries management can deliver even greater benefits, along with fewer environmental impacts, when it ensures stocks are not only biologically sustainable, but also abundant enough to allow catch volume or value to be maximised. A commonly used objective to define target reference points is MSY; that is, to produce the largest long-term average (sustainable) level of catch. Maximum economic yield (MEY) is another common objective, which aims to maximise economic productivity as opposed to the quantity of fish being produced.
To meet the growing need for more accessible and comparable information on the status of fish stocks worldwide (e.g. to ensure compliance with the new WTO Agreement on Fisheries Subsidies), the OECD created a unique data set on the status of assessed fish stocks bringing together data that are otherwise only available in a number of different forms and locations, making it both difficult to access for the non-technical policy maker and difficult to compare across countries. These data illuminate detailed trends in stock health for assessed stocks at a country level and provide information on trends in stock productivity, adding further nuance to the trends observed at global and regional levels and identifying priorities for action.
Box 2.2. OECD stock status data
As part of the OECD Review of Fisheries, the OECD now regularly collects data on stock assessments of harvested stocks with nationally determined biological sustainability standards (i.e. limit reference points, typically defined in terms of biomass or mortality thresholds) and higher management standards (i.e. target reference points, typically aimed at optimising catch value or volume under sustainability constraints).
In 2021, 32 countries and economies reported data on recent assessments from a total of 1 457 stocks (“recent” being defined as within the last ten years). The data do not contain any information on fish stocks where the status has not been assessed.
The OECD countries included in the data set are: Australia, Canada, Chile, Colombia, Denmark, the European Union (stock status only), Estonia, Finland, France, Germany, Greece, Italy, Japan, Korea, Latvia, Lithuania, the Netherlands, Norway, New Zealand, Poland, Slovenia, Spain, Sweden, the Republic of Türkiye, the United Kingdom, and the United States.
The emerging economies included in the data set are: Argentina, Brazil, the People’s Republic of China (hereafter “China”), Peru, Chinese Taipei, and Thailand.
Data, which reflect the most up‑to‑date understanding of stock status in reporting countries and economies in 2021, were used to produce country-level indicators on the health and productivity of assessed fish stocks with regard to national management standards:
The total number of stocks assessed reported on and of those:
the number of stocks in good health (i.e. stocks that meet all assigned sustainability standards)
the number of stocks that fall below sustainability standards (i.e. stocks below one or more limit reference points)
the number of stocks with an undetermined status (where an assessment was attempted but uncertainty in the results prevented a determination from being made).
the number of stocks in good health that meet higher management standards
the number of stocks in good health that fall below higher management standards
the number of stocks in good health where the status with respect to higher management standards is undetermined
the number of stocks in good health that do not have defined higher management standards.
These indicators are available on the OECD statistical portal.
Figure 2.1. Structure of the stock status data set
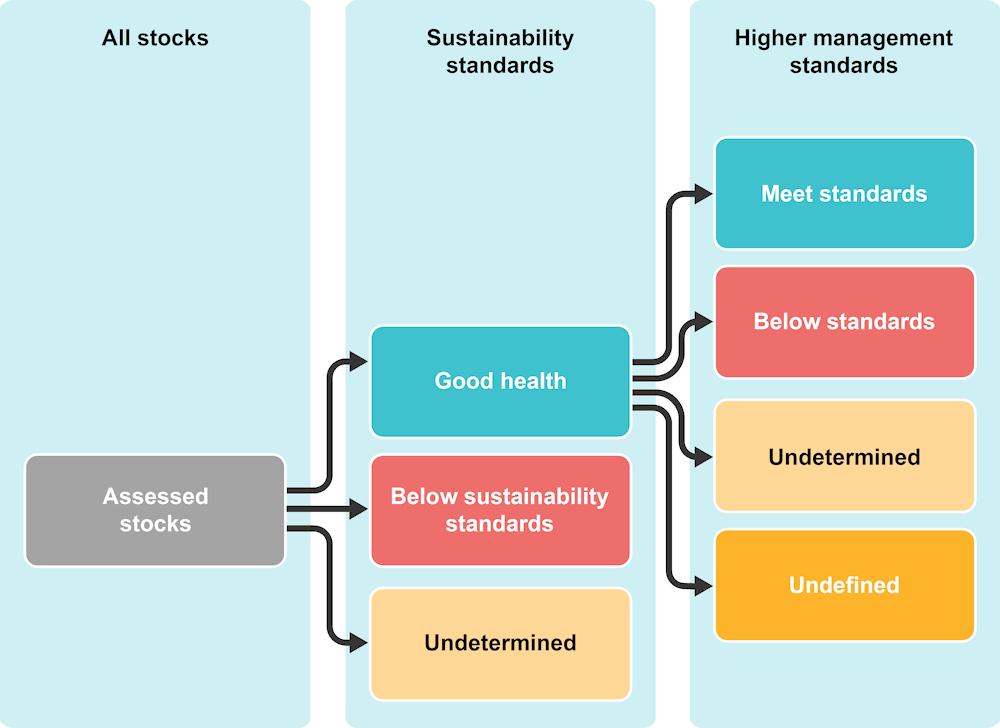
Note: The status of each stock is based on assessments conducted by the reporting countries or economies and no further calculations are conducted by the OECD.
2.2.1. The health of stocks is declining overall but varies across countries
Overall, recent assessments from 1 456 stocks were reported in 2021. Of these, 64% (939) were assessed as being in good health, 18% (258) fell below sustainability standards and 18% (259) had an undetermined status with respect to biological sustainability (Figure 2.2, left panel). Notably, stocks with an undetermined status are likely to be a mix of both stocks that are in good health and those that do not meet sustainability standards. Further, in all countries, some fish stocks have not been assessed and are not included in these data. Therefore, the actual proportions of both stocks in good health and stocks falling below sustainability standards in all harvested stocks likely vary from what is reported in the database (for assessed stocks).
Of the stocks which were in good health (939), 47% (438) also meet higher management standards (i.e. they were abundant enough to allow the volume or value of catch to be maximised under sustainability constraints). Further, 11% (103) fell below these higher management standards, 7% (67) had an undetermined status and, for 36% (331), no higher management standards were defined (Figure 2.2, right panel). As mentioned above, stocks with an underdetermined status may or may not meet higher management targets.
At the country level, there are significant variations in the number of stocks assessed and in their status with respect to biological sustainability (Figure 2.3). The mean number of stocks assessed by the countries and economies in the database is 57 and the median number of stocks assessed is 24.5. Australia reported the most stock assessments (449), followed by the United States (326) and New Zealand (183). There are many reasons why countries assess different numbers of stocks. The number of stocks that are commercially exploited, which is a factor of both the size of the sector and the marine areas of the country, is perhaps the most important one. The capacity of national authorities to conduct stock assessments also varies and can impact the number of assessments reported in the data set. Finally, in some cases where a large number of species are exploited in the same area, for example in tropical reef fisheries, it may not be practical or even possible to conduct individual stock assessments, which will also impact the number of stock assessments (Hilborn et al., 2020[2]).
The proportion of assessed stocks in good health also varies considerably across countries (Figure 2.3). In several countries – Estonia, Finland, Iceland, Korea, Latvia, Lithuania and Poland –all assessed stocks reported are in good health. However, bar Korea, all these countries reported assessments from seven or fewer stocks, which in the case of EU Member states may indicate missing data as only EU level stock assessments are reported in the data set. Colombia has the highest proportion of stocks which do not meet sustainability standards (52%), followed by Japan (51%) and Chile (45%).
The proportion of stocks with undetermined status also varies, with Thailand (100%), Spain (54%) and the United Kingdom (44%) reporting the highest proportion. While capacity constraints can prevent effective stock assessments, as is likely the case in Thailand, other factors such as the biology of the species being assessed are also important. For example, the Norway lobster (Nephrops norvegicus) is a burrowing species that is notoriously difficult to assess and consequently many European stocks are data poor and lack limit reference points (Aguzzi et al., 2022[24]). Both Spain and the United Kingdom exploit a high number of these stocks, hence the proportion of stocks with an undetermined status is inflated relative to other countries. Finally, in some cases, the COVID-19 pandemic has prevented effective assessments, further increasing the number of undetermined stocks.
Generally speaking, stock status numbers must be considered within their country-specific contexts, which will impact the number and type of assessments conducted, as well as the standards considered for biological sustainability. Natural variation in stock health, or the impacts of exogenous factors not linked to fishing, such as climate change, can have significant impacts on the health of stocks and lead to unexpected declines (or increases) irrespective of management regimes. Therefore, care must be taken not to draw strong conclusions from the evidence of this single data snapshot. Finally, it should be noted that the data do not provide insight into the efficacy of management in a particular country, or across countries.
With 18% of stocks falling below sustainability standards, there is considerable scope to increase catch and improve the economic conditions for fishers (and reduce GHG emissions) by rebuilding these stocks. As explored above, fisheries management generally works and rebuilding stocks to levels that can support MSY will likely add significant value to the fisheries sector of the countries and economies in the data set. In some cases, management regimes will have been adjusted already, to compensate for stocks falling below the thresholds for biological sustainability. The data do not account for any remedial action taken by countries to address stocks which are not meeting sustainability standards, nor does it contain data on the level of exploitation of the stocks in question. However, where management regimes have not been adjusted, fisheries managers should consider reviewing and adjusting them.
Investing in developing methodologies and capacity to understand the status of the 18% of stocks with undetermined status, and those unassessed, would also likely improve sustainability and increase economic returns in cases where overfishing is occurring but has not been detected or where stocks are underfished, particularly where the species are of significant commercial importance (e.g. Norway lobster). Moreover, the new WTO Agreement on Fisheries Subsidies prohibits subsidies to the fishing of overfished stocks and calls on members to take special care and exercise due restraint when granting subsidies to fishing stocks the status of which is unknown (Article 5.3). Clarifying the status of undetermined and unassessed stocks is thus important for compliance.
Notes: For EU Member States, stock assessments are reported at the EU level then assigned to the individual countries based on their initial allocation of quotas. Quota swaps made after the initial allocation are not considered. The allocation of stocks to individual Member States also means that the number of assessments will not sum to 1 457 due to double counting.
Source: OECD (2022[25]), Fisheries and Aquaculture Indicators, http://stats.oecd.org/wbos/default.aspx?datasetcode=FISH_INDICATORS.
2.2.2. Stock status changes 2019-21
The OECD started collecting data on stock status assessments as part of the Review of Fisheries 2020, making it possible to compare the data collected in 2021 to the data collected in 2019. In 2021, the number of stocks reported to the OECD increased from 1 119 in 2019 to 1 456. The bulk of the 333 additional stocks reported in 2021 came from two countries: Australia, which reported 168 additional stocks, and the United States, which reported 58 new stocks. The additional stocks are unlikely to be the result of new stocks being exploited but rather the result of additional assessments and a better data compilation and collection systems. Notably, Australia and the United States both have publicly available, centralised databases of stock status assessment: the Status of the Australian Fish Stocks Report3 in Australia and Stock Smart in the United States.4
The changing number of stocks between the two points in time in the data set means the relationship between the change in the share of stocks in good health in the data set and the actual trends in the health of assessed fish stocks is not straightforward. The data are not intended to be a globally representative sub-sample of stock health but rather the results of all stock assessments performed within the reporting countries and economies. As such, there is potential for biases to be introduced if the distribution of stock health in the new stocks is significantly different from the distribution of already included stocks. However, reporting stock health in 2021 only for the stocks reported in 2019 would have meant ignoring additional data, potentially biasing results, an issue that would only worsen over time as the data set expands. As such, the data are reported as “vintages”, such that 2021 and 2019 are considered snapshots of what was known about fish stock status at these dates, and comparisons are made on that basis.
The proportion of assessed stocks in good health has declined slightly, from 65.6% in 2019 to 64.5% in 2021 (Figure 2.4). Both of these proportions and the small decline seen between 2019 and 2021 closely mirror the globally representative data reported by the FAO in SOFIA 2022 (FAO, 2022[5]), which reports a slight decline in sustainably fished stocks from 65.8% in 2017 to 64.6% in 2019. The FAO data are based on a globally representative subset of stocks, so the close alignment between the two data sets suggests that the OECD data on assessed stocks may be a good approximation of the situation of fish stocks globally, despite its partial geographical coverage and the caveats discussed above.5 The proportion of stocks that do not meet sustainability standards has declined slightly, from 22.7% to 17.7%, while the proportion of stocks with an undetermined status has increased from 11.2% to 17.8%. The increase in undetermined stocks may be driven, at least in part, by the COVID-19 pandemic interfering with normal assessment processes (OECD, 2021[26]).
The proportion of stocks meeting higher management standards in the database has also declined, from 53.7% in 2019 to 46.6% in 2021, which is consistent with the trend in biological sustainability (Figure 2.5). Similarly, the number of stocks that fall below higher management standards has declined slightly, from 12.5% to 11%, while stocks with an undetermined status have increased from 3% to 7.1%. The proportion of stocks with undefined higher management standards has risen from 30.8% to 35.5%, largely due to the additional stocks reported for which higher management standards are not defined.
The decline in stocks meeting higher management standards (7%) is greater than the decline in stocks in good health (2%). Even when accounting for the large numbers of additional stocks with no defined higher management standards, which could exaggerate or mask trends, the decline in stocks achieving higher management standards between 2019 and 2021 is still 5%. The greater decline in stocks meeting higher management standards when compared to the decline in sustainability standards could be indicative of future trends in biological sustainability, if the stocks that no longer meet higher management standards continue to decline – a potentially worrying trend. However, whether the trends in status with respect to higher management standards can act as an early warning sign for biological sustainability will only become apparent as the time series develops.
2.3. Stock management of the most valuable species
2.3.1. The majority of stocks are covered by total allowable catch limits and other quotas
Fisheries management is the most important human-driven process impacting fisheries stocks (aside from fishing itself) and data on how fish stocks are managed are, therefore, important to the three pillars of fisheries sustainability. Fundamentally, fisheries management attempts to control the impact of fishing on stocks to ensure their long-term viability and the sustainability of the industry and communities that rely upon them. Management regimes are generally a collection of tools that aim to limit either the way fish are caught (input controls) or the level and type of catch (output controls).
Input controls regulate fleet and gear characteristics (e.g. vessel size and power, gear type and configuration), along with how that can be applied (with spatial or temporal restrictions). Output controls usually take the form of quotas, typically through total allowable catch (TAC) limits, which cap the total quantity of an individual stock that can be harvested. Individual or community quotas are sometimes used to augment TACs and they define the conditions under which catch shares can be sold or exchanged (or not). Output controls also include regulations on minimum fish sizes, which regulate the type of catch rather than the overall level of catch.
Increasingly, governments are looking towards ecosystem-based fisheries management (EBFM) as a way of ensuring both the sustainability of fisheries and the continued delivery of other ocean ecosystem services. EBFM is a holistic approach that considers fisheries resources in the context of the wider ecosystem, making the maintenance of that ecosystem and its services the primary function of the management approaches (Pikitch et al., 2004[27]).6 This is in contrast to traditional fisheries management, which generally considers fish stocks individually, with the level of harvest for each as the target of management actions. Effective EBFM plans require the integration of many different management actions, some of which are not traditionally used to manage fisheries (Levin et al., 2018[28]). However, EBFM can be difficult to implement in practice given the high data requirements, particularly for areas where data may be lacking (e.g. detailed marine habitat maps). This chapter, therefore, looks specifically at the tools used to manage stocks as a first step in documenting fisheries management practices.
To better understand how countries use different tools to manage their fish stocks, the OECD sent out a questionnaire to collate data on how countries and economies manage their most valuable harvested stocks. The data focus on traditional fisheries management tools as a first step in documenting fisheries management and as input in any future assessment of how EBFM is implemented at the country level.
Box 2.3. OECD stock management data
As part of the OECD Review of Fisheries, the OECD now regularly collects data on how countries and economies manage their most valuable harvested stocks. The data set covers each country’s five most valuable species (as per 2018 data), and data are reported at the stock level (i.e. if different stocks of one species are managed differently the information is reported for each stock of that species individually). In 2021, 29 countries and economies reported data.
The OECD countries included in the data set are: Australia, Canada, Chile, Colombia, Denmark, Estonia, Finland, France, Germany, Greece, Italy, Japan, Korea, Latvia, Lithuania, the Netherlands, Norway, New Zealand, Poland, Slovenia, Spain, Sweden, the Republic of Türkiye, the United Kingdom and the United States.
The emerging economies included in the data set are: Argentina, Brazil, China, Peru and Chinese Taipei.
For each stock, detailed information is provided on the different tools used in management, covering both:
Input controls, which regulate fleet and gear characteristics (e.g. vessel size and power, gear type and configuration), along with where and when fishing can take place (e.g. with spatial or temporal restrictions)
Output controls, which set harvesting limits either at the level of a fishery, with total allowable catch (TAC) limits that cap the total quantity of an individual stock that can be harvested, or at the level of individuals or communities, with specific quotas (e.g. individual transferable quotas, individual quotas or community quotas). Specific quotas usually define the conditions under which quotas can be sold and exchanged (or not). Output controls also include regulations on minimum fish sizes, which regulate the type of catch rather than the overall level of catch.
The data are then used to compile summary statistics such as the frequency of use of different management tools across the stocks in the data set; the proportion of landings – in volume and value – covered by different tools; and the co-occurrence of different tools at the stock level. These statistics are available on the OECD statistical portal.
The data set covers each country’s five most valuable species (as per 2018 data). On average, the top five species by value represented 59% of the value of all landings by the reporting countries and economies. In 81% of cases, the top five species represented more than 40% of the total landings, reaching more than 90% in Argentina, Finland and Poland. Therefore, the top five species can be considered representative of how countries generally approach fisheries management.
This is the second time these data have been collected. For the 2020 edition of the OECD Review of Fisheries, data were collected on the management tools used for the top five most valuable species in 2016. Due to natural variation in the value of landings, the top five species in 2016 may have been different from those in 2018; to maintain a consistent time series, data were collected for both the same species as for the 2020 edition and for any new species that entered the top five species by value in 2018. Consequently, some countries have submitted data on more than five species.
The data were also collected at a stock level, meaning that if a country exploits more than one stock of the species, data are reported on the tools used to manage each stock for each of the species in question. The data set, therefore, contains information on 313 stocks of 107 species from 29 countries and economies.
2.3.2. The majority of landings from the most valuable species came from stocks covered by total allowable catch limits
In 2021, the most frequently used management tools were gear restrictions, which were used in the management of 87% (273) of stocks, while TACs were the second-most commonly applied tool and were used in 76% (238) of stocks (Figure 2.6). This pattern is very similar to what was seen in 2019, which is expected given the large overlap in the two data sets. In 2020, species covered entirely by TACs accounted for USD 9.2 billion in landings, or 61% of the value of landing for all the species in the data set (Figure 2.7). A further USD 900 million (6%) was from species where some, but not all, of the stocks are covered by TACs (partial coverage), while USD 5.1 billion (33%) came from species where stocks are not covered by TACs.7 Species covered entirely by TACs accounted for 12.6 million tonnes of fish, 81% of all landings from the most commercially important species, while species partially covered by TACs provided a further 0.2 million tonnes (or 1% of landings by volume) and species not covered by TACs provided 2.8 million tonnes (or 18%). At the country level, 27% of countries reported using TACs in all of the stocks reported to the OECD, while over half the countries reported using TACs in more than 80% of the reported stocks.
Notes: TAC: total allowable catch; ITQ: individual transferable quota. The number of stocks using the tool is given in brackets.
Note: TAC: total allowable catch. Landings values were not available for Japan for 2020 therefore data from 2019 were used.
Source: OECD (2022[29]), OECD (2022), Marine landings, http://stats.oecd.org/wbos/default.aspx?datasetcode=FISH_LAND.
Aside from TACs, other forms of quotas, such as individual quotas (IQs) or individual transferable quotas (ITQs), are also used to manage fisheries and incentivise economic efficiency and improved resource stewardship. ITQs were used in 42% of stocks and IQs in 16%, while only 7% of stocks (22 in total) used both IQs and ITQs simultaneously. Therefore, just over half the reported stocks used either ITQs or IQs. Community-managed quotas (CMQs) are also used for output control. They differ from IQs and ITQs as they place the quota in the control of a group of fishers rather than allocating the rights individually. CMQs were used in 13% of stocks and TACs were present in almost all of them. In just over half of the stocks using CMQs, ITQs were also used. CMQs were more likely to be used to manage benthic and demersal species than pelagic fishes (Annex Table 2.A.1 and Annex Table 2.A.2).
2.3.3. Input controls are used in nearly all stocks in the database with gear restrictions and area restrictions the most frequently used
Of the 318 stocks included in the database, just 2% (6) do not use input controls. Of these, four stocks did not use any management tools at all. A combination of three different quotas is used in the remaining two stocks. Therefore, there are constraints in almost all stocks on how and where fish are caught. The most frequently used input controls are gear restrictions, which are used in 87% (273) of the stocks, making them the most frequently applied of all the management tools in the database (Figure 2.6). Area restrictions were the second-most frequently applied input control and were used in 66% (206) of stocks, while harvest capacity limits were found in 58% (181) of stocks.
The frequent use of gear restrictions to manage fisheries of all types is expected. For most fisheries, several different types of gear can be used. However, different types of gear vary both in their effectiveness for catching target species as well as in the extent to which they trap non-target species and impact the environment. This is true for both active and passive gear; gillnets, for example, are a passive gear that can be used to efficiently harvest certain species, but have been implicated in marine mammal and sea bird bycatch (Regular et al., 2013[30]; Read, Drinker and Northridge, 2006[31]). Trawl nets, an active gear, can efficiently target benthic species, but can degrade benthic habitats (Eastwood et al., 2007[32]). Therefore, fisheries managers require gear controls to balance the trade-offs between environmental sustainability and profitability in many fisheries, hence their popularity as a management tool.
Area-based restrictions to fishing, such as limitations on allowed fishing practices in the context of marine protected areas (MPAs), are a traditional fisheries management tool that has gained increasing prominence under EBFM (Halpern, Lester and McLeod, 2010[33]). Area-based restrictions can have significant positive effects on marine ecosystems and are often relatively straightforward to implement, which is likely a factor in their frequent use (Box 2.4). However, designing an effective system of area restrictions can be technically challenging and expensive to enforce (Gill et al., 2017[34]). Further, the data do not include information on the use of EBFM and more information is needed to understand how governments are using this more holistic approach to fisheries management.
Box 2.4. Area-based restrictions in fisheries management
There are several different types of area-based restrictions, including marine no-take zones, fish stock recovery areas (which can be designated under the European Union’s common fisheries policy), marine protected areas (MPAs) and other effective area-based measures. MPAs are perhaps the most widespread area restrictions and have expanded rapidly since 2005 (UNEP-WCMC and IUCN, 2021[35]) driven primarily by Aichi Target 11, which calls for 10% of territorial waters to be covered by protected areas. Countries are currently negotiating a new deal at the Convention on Biological Diversity and there are calls for a target of 30% in any new agreement. Given their increasing numbers, it is important to understand how area-based restrictions, and in particular MPAs, impact fisheries and what role they can play in both traditional fisheries management and ecosystem-based fisheries management (EBFM).
From a biodiversity perspective, there is significant evidence highlighting the effectiveness of MPAs at increasing fish biomass, species diversity and restoring ecosystem processes (Sala et al., 2018[36]; Aburto-Oropeza et al., 2011[37]; Sala and Giakoumi, 2017[38]). Evidence also suggests that larger and older MPAs that are more strongly protected are more effective (Edgar et al., 2014[39]). Ecosystem-level benefits, such as spillover effects and increased biomass, are the most well-studied benefits for fisheries (Mangi et al., 2022[40]). However, MPAs are part of complex socio-ecological systems that have broad consequences for the local economy and community (Rees et al., 2018[41]; Gaines et al., 2010[42]), which are relatively understudied (Rees et al., 2021[43]) (Rees et al., 2021[43]).
In general, MPAs lead to an initial decline in fisheries revenue if fishers are excluded from regions where they previously harvested. This decline is often followed by a slow increase in revenues as the ecosystem benefits such as spillover effects develop, leading to increased catch per unit effort in surrounding fisheries (Mangi et al., 2022[40]). The formation of an MPA is also often accompanied by increasing opportunities for revenue from non-fishing related activities, such as tourism (Pascual et al., 2016[44]). Empirical work suggests that a well-designed reserve network can increase fisheries profit (White et al., 2008[45]). However, the development of additional revenue streams and the recovery of fisheries revenue can take several years to manifest, potentially leaving local communities worse off in the short term (Mangi et al., 2022[40]). Further, in some cases, revenues may not recover, and additional revenue streams may not be able to make up for this shortfall, leading to economic losses over the longer term. These losses can be significant, such as in the case of the Phoenix Island Protected area in Kiribati, which is alleged to have cost the government USD 145 million in lost revenue from fisheries between 2015 and 2021 and is now potentially being opened to commercial fishing (Carreon, 2021[46]).
Importantly, the people bearing the costs of an MPA may not be those reaping the benefits from it (Rees et al., 2021[43]) (Rees et al., 2021[43]). For example, the spillover effects may be in different species, which some fishers cannot exploit due to a lack of the right gear or quotas, and fishers may not be able to take advantage of new revenue streams, such as from tourism. So even in cases where the benefits are greater than the costs, and the community as a whole is better off, it is still possible for fishers to suffer adverse consequences. When integrating MPAs into fisheries management, which is common under EBFM, it is important to understand the socio‑economic system in which they operate to prevent adverse economic impacts on particular groups.
2.3.4. Multiple input controls are often used to manage stocks, in particular for benthic species
On average, stocks have 3.6 input controls in place; however, this number obscures some important trends in how input controls are used in combination with quotas and each other. Stocks without TACs use 3.9 input controls on average, more than stocks with TACs, which use 3.5, likely because additional measures are required to control fishers in the absence of overall catch controls. Stocks with no quota or TAC at all used on average 4 input controls, supporting this conclusion. However, in contrast to what was observed in the OECD Review of Fisheries 2020, stocks that use TACs with additional quotas actually used marginally more input controls (3.7) than those that used TACs without additional quotas (3.5).
Table 2.1. Use of input controls by quota type
|
Number of input controls |
---|---|
Total allowable catch |
3.5 |
Individual tradable quota |
3.7 |
Individual non-transferable quota |
4.8 |
Community-managed quota |
4.3 |
Output controls were used in conjunction with input controls in almost all stocks; however, the use of input controls varied across the different types of quotas (Table 2.1). Stocks that use IQs and CMQs used considerably more input controls on average than other stocks, 4.8 and 4.3, respectively. Stocks using ITQs used 3.7 input controls on average by comparison, only slightly above the average for all stocks. It is not clear why stocks with IQs and CMQs use a higher than average number of input controls. More research and data are needed to understand if there is indeed some causal relationship or if it is simply an artefact of a relatively small sample size; only 49 stocks use IQ and 42 use CMQs.
Stocks of benthic species (109 stocks) use on average 4.4 input controls, which is higher than stocks of demersal species (2.8 input controls on average in 63 stocks) and pelagic species (3.0 input controls on average over 118 stocks). This likely reflects the challenges of managing benthic stocks compared to pelagic or demersal stocks, including the protection of benthic habitats, which are important for both fish stocks and a healthy ocean in general. Benthic stocks were more likely than average to have vessel power restrictions, season restrictions, investment restrictions and harvest capacity limits.
Fisheries managers use a combination of tools to manage fisheries. Understanding which combinations of tools are effective is essential for good fisheries management. The first step is to examine the co-occurrence of management tools to explore which combinations tend to occur together. The blue boxes in Figure 2.8 indicate a statistically significant association between two management tools and the number is the Cramer’s V statistic, a measure of the strength of that association. There are significant associations between TACs and other forms of quota (ITQs, IQs and CMQs), with TACs having the strongest association with ITQs. However, ITQs, IQs and CMQs are not significantly associated with each other, which indicates that they do not occur together regularly. This is likely because integrating multiple different quotas systems is challenging and can lead to overly complex management regimes, which might reduce compliance (Healey and Hennessey, 1998[47]).
Figure 2.8. Statistical associations between different management tools, 2021
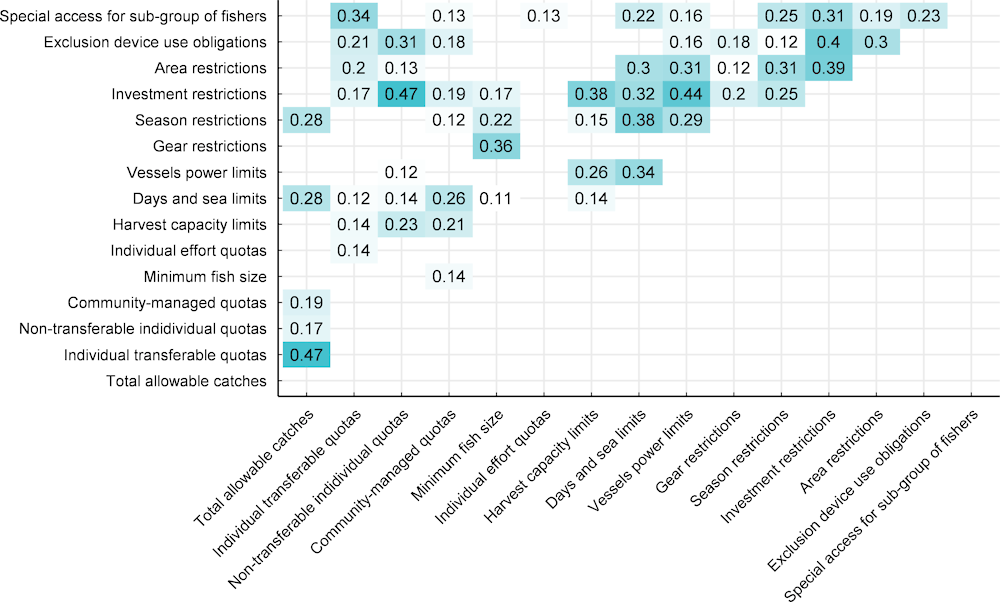
Notes: Shaded boxes indicate a statistically significant (at p< 0.05) association between two management tools based on a Chi squared test. The numbers in the boxes are the Cramer’s V statistic, which is a measure of the effect size of the association between the management tools. A medium association will have a Cramer’s V of 0.3-0.5 and a small association of 0.1-0.3.
In contrast to quotas, input controls share a high number of significant associations. This confirms the patterns described above indicating that multiple input controls tend to be used on the same stocks to control various aspects of fishers’ activity. The three strongest associations between input controls are between investment restrictions and vessel power limits, exclusion device obligation and area restrictions. These associations highlight how packages of input controls generally try to simultaneously limit where, how and how much fishing is taking place. However, the reason for the associations is not clear in some cases. For example, the strongest association is between IQs and investment restrictions, which is not easily explained. More research is needed to understand what is driving particular combinations of management tools and how this can impact the efficacy of fisheries management regimes.
2.4. Combining stock status and management data
Understanding how management tools impact fisheries outcomes is very important for ensuring the long‑term sustainability and productivity of fisheries resources. However, making links between management tools and stock status is complicated, as there is a time lag between management action and any impact on stock health and, as explained above, stock status is impacted by many factors besides management tools, such as climate change. Any analysis must avoid assigning causality erroneously. The long-term goal of this data collection is, nevertheless, to create a time series of data that will allow an analysis of how management tools impact stock status.
However, building this time series is a challenge in itself. The way stocks are labelled by management authorities and entities in charge of stock assessments varies across countries, and sometimes across national bodies or across time. For example, the International Commission for the Exploration of the Seas assesses stocks harvested by countries in the North Atlantic Ocean, including several European Union Member States. In doing so, however, it uses stock names which do not align with those used by the European Commission in the allocation of quotas. As such, it is not always easy, or even possible, to reconcile information on stock status with information on stock management. It is also sometimes difficult to find information about the volume and value of landings of a particular stock.
Using consistent names for stocks, particularly those shared between countries, would allow more stocks to be included in this data set, and generally help illuminate the effectiveness of fisheries management. Countries should consider adopting good-practice standards for reporting data at the stock level, particularly in the case of shared stocks. These standards could mandate the use of consistent names or codes for shared stocks, the use of ASFIS (Aquatic Sciences and Fisheries Information System) codes for harvested species (where available), and publicly available notices of any changes to naming conventions for species and stocks.
For just over a quarter of the stocks (80) reported to the management survey, information on the status of the stock in 2021 was also identified in the stock status data set. In 73 of these stocks, the status in 2019 was also available. What follows is a description of these data and should be interpreted with the above caveats in mind.
The majority (65%) of the stocks with both management and status data available were in good health, 15% fell below sustainability standards and 20% had an undetermined status, which is largely in line with the wider data set. There are differences in how management tools are used across these categories (Figure 2.9Figure 2.2), but strong inferences are not possible given the relatively low sample size and the short time series. For example, while 100% of stocks falling below sustainability standards have a TAC, it is not possible to know if the TAC was instigated before the health decline or after, as part of a rebuilding plan. Further, only 12 stocks in the data set fell below sustainability standards, making it difficult to know if this is a pattern or an artefact. Therefore, linking management tool use to stock status outcomes is not currently possible and care should be taken when interpreting these numbers.
Note: TACs: total allowable catches; ITQs: individual transferable quotas.
There are, however, some interesting general trends. Stocks that fell below sustainability standards have the fewest management in tools on average (4.4), followed by stocks that were in good health (5.6) and stocks with an undetermined status (7.8). Input controls are used more frequently to manage stocks with an undetermined status than stocks that were either meeting or not meeting sustainability standards. On average, stocks with an undetermined status have an average of 4.9 different input controls, while stocks either meeting or not meeting standards use 3.3 and 2.3 different input controls, respectively. Notably, controls that limit fishing effort and capacity (harvest capacity limits, days at sea limits, vessel power limits, investment restrictions and season restrictions) were all more common in stocks with an undetermined status than in stocks where the status has been determined.
The increased use of input controls for stocks with an undetermined status is likely due to features of those stocks which favour input controls and pose challenges to effective assessment. Indeed, benthic species are overrepresented among stocks with an undetermined status. Across all 80 stocks, benthic species account for 45% of stocks but represent 63% of stocks with an undetermined status and include species such as the Norway lobster, the great Atlantic scallop, the Queen crab and the American lobster. Therefore, it may be features of the species itself (e.g. difficult to assess) driving both the increased use of input controls and the undetermined status.
2.5. The challenge of ghost fishing gear
Managing fisheries is complicated by exogenous factors which can directly impact the health of stocks. Ghost fishing gear (hereafter ghost gear), which refers to fishing gear that remains in the sea after having been lost, abandoned or discarded, is one such factor.8
The unintended capture of marine biota by fishing gear after it has been abandoned, lost or discarded at sea can indeed have important consequences for fisheries.9 These include a reduced abundance of target species, which can reduce fishers’ CPUE and thus their profitability and have adverse impacts on local and global food security.10 In fisheries where the impact of ghost gear on target species mortality is significant, management can be directly affected by the phenomenon.11 Failing to account for the impact of ghost gear would indeed bias management targets and compromise the achievement of sustainability objectives.
Beyond the impacts on fisheries, ghost gear can have significant impacts on the marine environment. Ghost gear is believed to cause the vast majority of entanglement cases, which is particularly damaging for large marine fauna.12 For this reason, the damage potential of ghost gear is believed to be higher than its share of ocean plastic by volume. Further, through abrasion and smothering of the seabed, ghost gear also adversely impacts corals and other benthic organisms (MacFadyen, Huntington and Cappell, 2009[48]; GESAMP, 2021[49]).
Largely comprised of plastic material, ghost gear also generates many of the environmental challenges caused by marine plastic litter, including deterioration into microplastics and subsequent bio‑accumulation in marine organisms.13 Floating marine debris from ghost gear can also transport encrusting organisms such as bacteria or algae, leading to the introduction of alien species harmful to a new ecosystem. The debris also act as vectors of microbial communities potentially harmful to ecosystems and human health (Gilman et al., 2021[50]; OECD, 2021[51]).
Given the difficulty of observing the quantity of ghost gear, lack of evidence on the magnitude of unintended catches through ghost fishing and thus difficulty in estimating its mortality impact, fisheries management should not only try to account for it, but also strive to reduce its occurrence as much as possible. In 2021, the OECD was commissioned to produce a report on how G7 members were addressing the issue of ghost gear and how they could consider adopting best practices to do so more effectively. What follows is a condensed and updated version of the report produced in this context: “Towards G7 action to combat ghost fishing gear” (OECD, 2021[6]).
2.5.1. The causes of ghost gear are varied and measuring its magnitude and impact challenging
Fishing gear can be abandoned, lost or discarded at sea for a number of reasons, driven by the natural environment or by human actions. Adverse weather conditions such as storms, strong currents or winds can carry gear away, remove visual locators, or make it difficult and dangerous for fishers to retrieve gear. Interactions between fishing gear and wildlife, other gear, vessels, rocks, corals and man-made structures lying beneath the surface can also cause its loss (Richardson et al., 2021[52]; GESAMP, 2021[49]). Entanglement of mobile gear such as trawling nets with static gear located in the same area is a common reason for loss (MacFadyen, Huntington and Cappell, 2009[48]). Gear can also be lost due to misuse, such as deploying too many nets or when gear is left at sea for too long. In addition, gear is sometimes intentionally discarded or abandoned at sea when fishers consider it more practical and economical than disposing of gear onshore. Notably, this can happen when port-side reception facilities for end-of-life gear are lacking. Finally, gear can be discarded to hide illegal, unreported and unregulated fishing practices.
It is difficult to estimate the volume of ghost gear in the oceans since it is difficult to detect and comes from a wide variety of sources. Research quantifying ghost gear is often specific to particular types of gear and geographic areas. Evidence suggests that a significant proportion of some types of gear is lost, however estimates vary. A meta-analysis of papers looking at the magnitude of ghost gear production concluded that, on average, 5.7% of nets, 8.6% of traps and 29% of lines in use are lost each year (Richardson, Hardesty and Wilcox, 2019[53]).14 A subsequent study found an average 1.82% of fishing gear is lost annually across all gears (Richardson et al., 2022[54]). Even if gear is lost at lower rates that previously thought, it is significant. For example, a 3.3% annual loss in longlines equates to nearly 740 000 km of fishing line (Richardson et al., 2022[54]).
Studies on the prevalence of ghost gear also suggest that it can constitute a significant source of marine debris. For example, an estimated 70% of marine debris by mass in the five main ocean gyres is fishing related (UNEP, 2016[55]). Another study estimated that fishing gear makes up between 75 and 86% of the floating plastics in the North Pacific Garbage Patch (Lebreton et al., 2022[56]). At local levels, fishing gear was found to account for about half of the marine debris collected in retrieval programmes for the outer shoreline of British Columbia (Markel and Smith, 2020[57]) and was the main source of plastic litter found in beach litter surveys undertaken in the Maritime Area of the Convention for the Protection of the Marine Environment of the North-East Atlantic (OSPAR Commission, n.d.[58]).
Bridging the gap between global-level estimations of ghost gear and local-level studies is challenging. There is no agreed methodology for measuring ghost gear, which poses challenges when aggregating and comparing regional estimates. In addition, the frequency of gear loss, discard and abandonment at sea remains largely undocumented in many areas of the world (GESAMP, 2021[49]). As a result, global estimates vary significantly. Most recently, the global volume of ghost gear was estimated to be 1.5% of all oceanic macro-plastics at the low end (300 000 t) (OECD, 2022[59]) and 9-10% of all ocean plastics at the high end (Eunomia, 2016[60]).
In addition, the OECD (2022[59]) estimates that plastic leakage from marine activities (mostly from fishing) will more than double globally to reach 600 000 t in 2060 – 17% of which would originate from OECD countries. More research on the quantity of ghost fishing gear is urgently needed: understanding where and how ghost gear is produced is key to more targeted action. This research requires more and better data on fishing effort and location, the quantities and types of gear deployed, rates of loss (and replacement), and the composition of gear (especially the types of plastics used), particularly in the parts of the world where data are scant (GESAMP, 2021[49]). Research could also draw on better data on the actual presence of plastics in the sea and refined parameters on the share of total plastics originating from fishing.15
2.5.2. Good practices to address ghost gear
The international community is already taking action to address the issue of ghost gear, which is regularly discussed in the context of international and multilateral initiatives to reduce ocean plastic pollution. For example, it was raised in the context of the WTO Informal Dialogue on Plastics Pollution and Environmentally Sustainable Plastics Trade (IDP). Launched in November 2020 by a group of WTO members, the IDP seeks to address the rising environmental, health and economic cost of plastics pollution with trade-related solutions (WTO, 2021[61]).16 In the near future, the issue could also be addressed in the context of negotiations for an international legally binding instrument on plastic pollution, including in the marine environment, under the United Nations Environment Assembly.17
The Global Ghost Gear Initiative was created in 2015 as a multi-stakeholder initiative bringing together the fishing industry, academia, governments, intergovernmental organisations and non‑governmental organisations with the specific purpose of addressing the problems associated with ghost gear worldwide. It targets prevention, mitigation, retrieval and recycling actions, information dissemination, and capacity building around best practices to avoid creating ghost gear and minimise its detrimental impact.18
In 2021, the OECD prepared the report “Towards G7 action to combat ghost fishing gear” to support the United Kingdom’s presidency of the G7 (OECD, 2021[6]). The objective was to take stock of policy actions undertaken by G7 members to address ghost gear and, building on available evidence, to identify the scope for scaling-up and sharing best policies among G7 members and beyond. The report concludes that a comprehensive policy response is required to address ghost gear and should combine a range of government and industry actions, as well as collaboration among stakeholders at local, regional and international levels. The G7 Environment Ministers Communiqué mentioned the need for a comprehensive policy response.19
The following section briefly summarises the policy conclusions from the OECD report and updates the review of good practices to address the issue of ghost fishing gear conducted at that time, focusing on the OECD countries and partner economies covered in this report.
Gear marking
The marking of fishing gear can both help to prevent gear loss and discard and reduce the adverse impacts of ghost gear by facilitating the location and retrieval of lost gear. In 2019, the FAO adopted Voluntary Guidelines on the Marking of Fishing Gear (FAO, 2019[62]). The guidelines encourage FAO member states and regional fisheries bodies to collaborate developing and implementing pragmatic and harmonised marking systems that identify the owner and position of fishing gear, and link the gear to the person or entity responsible for fishing operations. Other actions of gear marking include a recent decision by the International Maritime Organization to implement mandatory gear marking through Annex V of the MARPOL Convention country-level mandatory gear marking systems (OECD, 2021[6]).
Improved gear and vessel design
Improving fishing gear design can help prevent loss and mitigate the effects of ghost gear. Three particularly promising areas for gear improvement include: 1) affordable and efficient marking and tracking technologies (FAO, 2019[62]); 2) escape cords and panels that can free trapped fish and reduce the ghost fishing capacity (particularly for traps); and 3) excluder devices such as the use of weak ropes or hooks, tie-downs and nets with a lower profile, rope-less fishing or entrance barriers (FAO, 2021[63]). Better gear design can also help address issues related to gear recyclability, by facilitating the separation of different plastic components. By increasing prospects for valorisation of used gear in a circular economy approach, increased recyclability can reduce incentives to abandon gear at sea. Vessel design can be improved too, for example by ensuring adequate space on-board to stow gear and emergency retrieval tools. Gear and vessel design requirements, incentives to adopt better gear designs and support for research for gear innovation can all help harness the potential of gear improvements to reduce ghost gear.
Reporting and retrieval
Reporting and retrieval help address unavoidable gear loss, for example due to extreme weather conditions. When gear is lost, immediate retrieval (when safe to do so) is the best solution to avoid detrimental impacts but it requires training and adequate equipment. If immediate recovery is not possible, loss reporting can help subsequent relocation and recovery and avoid entanglement with other vessels. Annex V of the MARPOL Convention makes reporting discarded, abandoned or lost fishing gear mandatory. The importance of reporting and retrieval is also stressed in the FAO Voluntary Guidelines on the Marking of Fishing Gear (IMO, 2017[64]; FAO, 2019[62]).
Retrieval should be incentivised and regulations are needed to ensure that fishers are not punished for carrying retrieved gear lost by others that is unauthorised. Pilot and end-of-season retrieval programmes, preferably conducted in collaboration with fishing communities and associations, are also effective at reducing the impact of ghost gear and increasing awareness of the issue.
Disposal facilities
Providing adequate disposal facilities at ports can help reduce purposeful discarding of damaged fishing gear at sea by offering fishers an avenue for disposing of end-of-life gear. Disposal facilities can also improve the collection of end-of-life gear and facilitate the preparation of gear for reuse or recycling. Under MARPOL, parties should ensure that adequate reception facilities are available at ports for disposing of garbage without causing undue delay (IMO, 2017[64]).20 However, evidence suggests that adequate port reception facilities are often lacking (MacFadyen, Huntington and Cappell, 2009[48]; Richardson et al., 2021[52]). Extended producer responsibility for fishing gear manufacturers could also facilitate disposal by shifting some of the costs of waste management from small ports and fishing operators to gear manufacturers (OECD, 2021[6]).
Spatial and temporal management
Marine spatial and temporal management measures can help prevent gear conflict, especially between mobile and static gear. These measures assign delimited geographical areas or periods of time to different marine activities or to produce and share information on the locations and timings of different activities among users of marine space and resources. In many cases, marine spatial planning started as voluntary agreements between fishers, such as in crab fishery in British Columbia (Canada) or Washington State (United States). It is now a priority for national action in many countries (OECD, 2021[6]).
Trade facilitation
Trade can enable the efficient allocation of end-of-life material such as fishing gear by facilitating the movement of material to markets with a comparative advantage in waste processing. For example, fishing gear collected in Iceland and Norway is typically shipped to continental Europe for recycling and other environmentally sound waste management (van Nijen, 2021[65]). However, challenges arise when separating the different components of fishing gear (Yamaguchi, 2018[66]).
Trade policy can facilitate the trade of less harmful plastics and substitutes and disincentivise (or even forbid) trade in the most harmful plastics, potentially creating incentives for producers and consumers to choose less harmful plastics and substitutes. New trade requirements aim to reduce trade in plastics that are difficult to recycle and to improve the quality of traded plastic waste. Such requirements have been implemented by traditional plastics importers (notably China), the Basel Convention on the Control of Transboundary Movements of Hazardous Wastes and their Disposal, and the OECD Decision of the Council on the Control of Transboundary Movements of Wastes Destined for Recovery Options.
Navigating different and evolving trade policy and regulation systems can also create challenges. Reducing friction in trade regimes while maintaining the stringency of environmental standards would encourage environmentally beneficial trade in plastics (OECD, 2022[67]). Harmonisation or joint development of trade policy, regulation and product standards should be encouraged where possible. Finally, information and awareness raising of end-of-life challenges and how they relate to trade policy can help address end-of-life issues.
Education and awareness raising
Awareness raising of the adverse impacts of ghost gear can encourage behavioural change in fishers and consumers and create pressure for fisheries stakeholders to invest in avoiding gear loss. Training can also help gear recovery.
Table 2.2. Good practices to address ghost gear implemented across OECD countries and partner economies
Type of policy measure |
Selected policy examples in countries and economies covered in the OECD Review of Fisheries 2022 |
---|---|
Marking of fishing gear |
|
Vessel design |
|
Disposal facilities |
|
Spatial or temporal planning |
|
Gear design |
|
Education and awareness |
|
Reporting and retrieval of ghost gear |
|
Extended producer responsibility |
|
Note: These examples are not exhaustive.
Source: Authors’ assessment based on OECD (2021[6]); Global Ghost Gear Initiative (2022[68]); and Ocean Outcomes (2020[69]).
2.6. Conclusion
Around two-thirds of assessed fish stocks are in good health, but their health is declining, while nearly one in five stocks are not meeting their sustainability standards. Further, in some countries, the majority of assessed stocks do not meet sustainability standards. Rebuilding overfished stocks will bring several benefits, namely increased profitability for the sector and improved environmental sustainability. It should be a priority for governments looking to improve outcomes for the communities which rely on the fishing sector.
Furthermore, just under half the stocks in good health were meeting higher management standards such as MEY or MSY. Similarly, improving stock health to meet these higher management targets will benefit both the profitability of the sector and the environment, but likely also reduce emissions from the sector by increasing fishing efficiency. Managing stocks at optimal levels represents a low‑technology method of reducing emissions from the sector, which will become increasingly important as governments look towards achieving net-zero targets in the coming decades.
The task facing fisheries managers is difficult. Ensuring the sector’s economic, environmental and social sustainability requires management systems that can effectively constrain fishing activity while allowing fishers to operate profitably. There is no perfect management system, and the way fisheries respond to management action can be complex and unpredictable. Therefore, fisheries management needs to be able to adapt to changing circumstances to ensure the sustainability of resources over time. Accurate and timely data on the status of resources and the management actions in place is fundamental to adaptive fisheries management. The data linking management tools and stock health presented in this chapter are a first step to understanding how one impacts the other.
However, continuing efforts are needed to ensure the data can provide valuable insights to fisheries managers going forward. For example, the status of around one in five stocks was undetermined, although this number is much higher in some countries, and in many cases these stocks are commercially important. In addition, in some countries, very few stocks are assessed, so little is known about the health of the resources upon which coastal communities rely. Without a good understanding of their status, fishers are likely missing out on potential profits either through underfishing or overfishing. Developing methods and capacity to assess these stocks would, therefore, create a range of benefits for fishers and governments. Moreover, the new WTO Agreement on Fisheries Subsidies prohibits governments from subsidising the fishing of overfished stocks and calls for caution when subsidising the fishing of stocks with unknown status. Thus, clarifying the status of undetermined and unassessed stocks is also important for compliance with this agreement.
Finally, fisheries management is further complicated by external factors such as climate change, ghost gear and other human activities in the marine environment, which impact fish stocks in multiple ways. Understanding how such factors impact fish stocks is crucial for effective management. However, there are significant information gaps, particularly around the local-level impacts of these factors on fisheries. Accurate and timely data on these impacts would allow the better targeting of resources to enhancing fisheries management in areas which suffer the greatest impacts.
Annex 2.A. Additional management data tables
Annex Table 2.A.1. Number of stocks using management tools split by species type
|
Pelagic |
Benthic |
Demersal |
Other |
Total |
---|---|---|---|---|---|
Total allowable catch |
95 |
80 |
50 |
13 |
238 |
Individual transferable quotas |
56 |
45 |
26 |
5 |
132 |
Non-transferable individual quotas |
18 |
20 |
7 |
4 |
49 |
Community-managed quotas |
8 |
23 |
11 |
0 |
42 |
Minimum fish size |
64 |
63 |
48 |
5 |
180 |
Individual effort quotas |
5 |
8 |
1 |
4 |
18 |
Harvest capacity limits |
63 |
76 |
31 |
11 |
181 |
Days and sea limits |
13 |
37 |
9 |
3 |
62 |
Vessels power limits |
31 |
55 |
18 |
5 |
109 |
Gear restrictions |
91 |
104 |
61 |
17 |
273 |
Season restrictions |
50 |
53 |
15 |
9 |
127 |
Investment restrictions |
15 |
49 |
7 |
3 |
74 |
Area restrictions |
71 |
74 |
49 |
12 |
206 |
Exclusion device use obligation |
7 |
34 |
16 |
4 |
61 |
Special access for sub-group of fishers |
16 |
44 |
14 |
4 |
78 |
Annex Table 2.A.2. Number of stocks in the stock management database by species type
Pelagic |
119 |
Benthic |
109 |
Demersal |
63 |
Other |
22 |
References
[37] Aburto-Oropeza, O. et al. (2011), “Large recovery of fish biomass in a no-take marine reserve”, PLoS ONE, Vol. 6/8, p. e23601, https://doi.org/10.1371/journal.pone.0023601.
[24] Aguzzi, J. et al. (2022), “Advancing fishery-independent stock assessments for the Norway lobster (Nephrops norvegicus) with new monitoring technologies”, Frontiers in Marine Science, Vol. 9, https://doi.org/10.3389/fmars.2022.969071.
[77] Antonelis, K. et al. (2011), “Dungeness crab mortality due to lost traps and a cost-benefit analysis of trap removal in Washington State waters of the Salish Sea”, North American Journal of Fisheries Management, Vol. 31/5, pp. 880-893, https://doi.org/10.1080/02755947.2011.590113.
[4] Bahri, T. et al. (eds.) (2021), “Adaptive management of fisheries in response to climate change”, FAO Fisheries and Aquaculture Technical Paper, No. 667, Food and Agriculture Organization, Rome, https://doi.org/10.4060/cb3095en.
[8] Barange, M. et al. (2018), “Impacts of climate change on fisheries and aquaculture”, FAO Fisheries and Aqauculture Technical Paper, No. 627, Food and Agriculture Organization, Rome, https://www.fao.org/publications/card/en/c/CA0356EN.
[1] Barbier, E. (2017), “Marine ecosystem services”, Current Biology, Vol. 27/11, pp. R507-R510, https://doi.org/10.1016/j.cub.2017.03.020.
[15] Bianchi, M. et al. (2022), “Assessing seafood nutritional diversity together with climate impacts informs more comprehensive dietary advice”, Communications Earth & Environment, Vol. 3/1, https://doi.org/10.1038/s43247-022-00516-4.
[46] Carreon, B. (2021), “Kiribati to open one of world’s largest marine protected areas to commercial fishing”, The Guardian, https://www.theguardian.com/world/2021/nov/16/kiribati-to-open-one-of-worlds-largest-marine-protected-areas-to-commercial-fishing.
[10] Cheung, W. et al. (2010), “Large-scale redistribution of maximum fisheries catch potential in the global ocean under climate change”, Global Change Biology, Vol. 16/1, pp. 24-35, https://doi.org/10.1111/j.1365-2486.2009.01995.x.
[7] Cheung, W., R. Watson and D. Pauly (2013), “Signature of ocean warming in global fisheries catch”, Nature, Vol. 497/7449, pp. 365-368, https://doi.org/10.1038/nature12156.
[72] Cho, D. (2005), “Challenges to marine debris management in Korea”, Coastal Management, Vol. 33/4, pp. 389-409, https://doi.org/10.1080/08920750500217559.
[3] Costello, C. et al. (2016), “Global fishery prospects under contrasting management regimes”, Proceedings of the National Academy of Sciences, Vol. 113/18, pp. 5125-5129, https://doi.org/10.1073/pnas.1520420113.
[20] Driscoll, J. and P. Tyedmers (2010), “Fuel use and greenhouse gas emission implications of fisheries management: The case of the New England Atlantic herring fishery”, Marine Policy, Vol. 34/3, pp. 353-359, https://doi.org/10.1016/j.marpol.2009.08.005.
[32] Eastwood, P. et al. (2007), “Human activities in UK offshore waters: An assessment of direct, physical pressure on the seabed”, ICES Journal of Marine Science, Vol. 64/3, pp. 453-463, https://doi.org/10.1093/icesjms/fsm001.
[39] Edgar, G. et al. (2014), “Global conservation outcomes depend on marine protected areas with five key features”, Nature, Vol. 506/7487, pp. 216-220, https://doi.org/10.1038/nature13022.
[60] Eunomia (2016), Plastics in the Marine Environment, Eunomia, Bristol, https://www.eunomia.co.uk/reports-tools/plastics-in-the-marine-environment (accessed on 22 February 2021).
[5] FAO (2022), The State of World Fisheries and Aquaculture 2022: Towards Blue Transformation, Food and Agriculture Organization, Rome, https://doi.org/10.4060/cc0461en.
[63] FAO (2021), Fishing Operations: Guidelines to Prevent and Reduce Bycatch of Marine Mammals in Capture Fisheries, Food and Agriculture Organization, Rome, https://doi.org/10.4060/cb2887en.
[62] FAO (2019), Voluntary Guidelines on the Marking of Fishing Gear, Food and Agriculture Organization, Rome, https://www.fao.org/3/ca3546t/ca3546t.pdf.
[75] FAO (1995), Code of Conduct for Responsible Fisheries, Food and Agriculture Organization, Rome, https://www.fao.org/global-record/resources/detail/fr/c/879859.
[23] Gabrielli, C. and S. Jafarzadeh (2020), Alternative Fuels and Propulsion Systems for Fishing Vessels, SINTEF Energy Research, https://hdl.handle.net/11250/2684524.
[42] Gaines, S. et al. (2010), “Evolving science of marine reserves: New developments and emerging research frontiers”, Proceedings of the National Academy of Sciences, Vol. 107/43, pp. 18251-18255, https://doi.org/10.1073/pnas.1002098107.
[70] Gall, S. and R. Thompson (2015), “The impact of debris on marine life”, Marine Pollution Bulletin, Vol. 92/1-2, pp. 170-179, https://doi.org/10.1016/j.marpolbul.2014.12.041.
[14] Gephart, J. et al. (2021), “Environmental performance of blue foods”, Nature, Vol. 597/7876, pp. 360-365, https://doi.org/10.1038/s41586-021-03889-2.
[49] GESAMP (2021), “Sea-based sources of marine litter”, Joint Group of Experts on the Scientific Aspects of Marine Environmental Protection.
[73] GESAMP (2016), Sources, Fate and Effects of Microplastics in the Marine Environment: Part 2 of a Global Assessment, International Maritime Organization, London, http://www.gesamp.org/site/assets/files/1275/sources-fate-and-effects-of-microplastics-in-the-marine-environment-part-2-of-a-global-assessment-en.pdf.
[34] Gill, D. et al. (2017), “Capacity shortfalls hinder the performance of marine protected areas globally”, Nature, Vol. 543/7647, pp. 665-669, https://doi.org/10.1038/nature21708.
[50] Gilman, E. et al. (2021), “Highest risk abandoned, lost and discarded fishing gear”, Scientific Reports, Vol. 11/1, https://doi.org/10.1038/s41598-021-86123-3.
[68] Global Ghost Gear Initiative (2022), Best Practice Framework for the Management of Fishing Gear: June 2021 Update, Global Ghost Gear Initiative, prepared by Huntington, T. of Poseidon Aquatic Resources Management Ltd, https://www.ghostgear.org/s/GGGI-Best-Practice-Framework-for-the-Management-of-Fishing-Gear-C-BPF-2021-Update-FINAL.pdf.
[76] Global Ghost Gear Initiative (2018), “GWR Polymers/Newlyn harbour net recycling”, web page, https://www.ghostgear.org/projects/2018/10/10/gwr-polymers-newlyn-harbour-net-recycling (accessed on 20 May 2022).
[13] Greer, K. et al. (2019), “Global trends in carbon dioxide (CO2) emissions from fuel combustion in marine fisheries from 1950 to 2016”, Marine Policy, Vol. 107, p. 103382, https://doi.org/10.1016/j.marpol.2018.12.001.
[33] Halpern, B., S. Lester and K. McLeod (2010), “Placing marine protected areas onto the ecosystem-based management seascape”, Proceedings of the National Academy of Sciences, Vol. 107/43, pp. 18312-18317, https://doi.org/10.1073/pnas.0908503107.
[47] Healey, M. and T. Hennessey (1998), “The paradox of fairness: The impact of escalating complexity on fishery management”, Marine Policy, Vol. 22/2, pp. 109-118, https://doi.org/10.1016/s0308-597x(97)00037-7.
[2] Hilborn, R. et al. (2020), “Effective fisheries management instrumental in improving fish stock status”, Proceedings of the National Academy of Sciences, Vol. 117/4, pp. 2218-2224, https://doi.org/10.1073/pnas.1909726116.
[64] IMO (2017), 2017 Guidelines for the Implemenetation of MARPOL Annex V (MEPC 295 71), International Maritime Organization.
[74] Kuczenski, B. et al. (2021), “Plastic gear loss estimates from remote observation of industrial fishing activity”, Fish and Fisheries, Vol. 23/1, pp. 22-33, https://doi.org/10.1111/faf.12596.
[56] Lebreton, L. et al. (2022), “Industrialised fishing nations largely contribute to floating plastic pollution in the North Pacific subtropical gyre”, Scientific Reports, Vol. 12/1, https://doi.org/10.1038/s41598-022-16529-0.
[28] Levin, P. et al. (2018), “Building effective fishery ecosystem plans”, Marine Policy, Vol. 92, pp. 48-57, https://doi.org/10.1016/j.marpol.2018.01.019.
[38] Linwood, P. (ed.) (2017), “No-take marine reserves are the most effective protected areas in the ocean”, ICES Journal of Marine Science, Vol. 75/3, pp. 1166-1168, https://doi.org/10.1093/icesjms/fsx059.
[48] MacFadyen, G., T. Huntington and R. Cappell (2009), Abandoned, Lost or Otherwise Discarded Fishing Gear, Food and Agriculture Organization, Rome, https://www.fao.org/publications/card/en/c/b1c2166f-78d5-5c21-b678-fe30cd51b154 (accessed on 10 March 2021).
[40] Mangi, S. et al. (2022), Research for PECH Committee – Costs and Benefits of Spatial Protection Measures as Tools for Fisheries Management, European Parliament, Policy Department for Structural and Cohesion Policies, Brussels, https://www.europarl.europa.eu/RegData/etudes/STUD/2022/733087/IPOL_STU(2022)733087_EN.pdf.
[57] Markel, R. and K. Smith (2020), SSTOA and WTA Marine Debris Removal Initiative 2020: Coastal Environmental Protection, Employment, and Economic Recovery During the COVID-19 Pandemic, https://wilderness-tourism.bc.ca/wp-content/uploads/2021/01/SSTOA-MDRI-2020-FINAL-Report-PUBLIC.pdf.
[17] Mitchell, C. and C. Cleveland (1993), “Resource scarcity, energy use and environmental impact: A case study of the New Bedford, Massachusetts, USA, fisheries”, Environmental Management, Vol. 17/3, pp. 305-317, https://doi.org/10.1007/bf02394673.
[69] Ocean Outcomes (2020), Ghost Gear Legislation Analysis, WWF – World Wide Fund For Nature, Gland, Switzerland, https://www.oceanoutcomes.org/news/new-report-policy-solutions-regulations-ghost-gear.
[25] OECD (2022), Fisheries and Aquaculture Indicators, https://stats.oecd.org/Index.aspx?DataSetCode=FISH_INDICATORS.
[67] OECD (2022), Global Plastics Outlook: Economic Drivers, Environmental Impacts and Policy Options, OECD Publishing, Paris, https://doi.org/10.1787/de747aef-en.
[59] OECD (2022), Global Plastics Outlook: Policy Scenarios to 2060, OECD Publishing, Paris, https://doi.org/10.1787/aa1edf33-en.
[29] OECD (2022), “Marine landings”, Fisheries and Aquaculture database, https://stats.oecd.org/Index.aspx?DataSetCode=FISH_LAND.
[26] OECD (2021), “COVID-19 and multilateral fisheries management”, OECD Policy Responses to Coronavirus (COVID-19), OECD Publishing, Paris, https://doi.org/10.1787/cc1214fe-en.
[51] OECD (2021), Policies to Reduce Microplastics Pollution in Water: Focus on Textiles and Tyres, OECD Publishing, Paris, https://doi.org/10.1787/7ec7e5ef-en.
[6] OECD (2021), “Towards G7 action to combat ghost fishing gear: A background report prepared for the 2021 G7 presidency of the United Kingdom”, OECD Environment Policy Papers, No. 25, OECD Publishing, Paris, https://doi.org/10.1787/a4c86e42-en.
[16] OECD/FAO (2022), OECD-FAO Agricultural Outlook 2022-2031, OECD Publishing, Paris, https://doi.org/10.1787/f1b0b29c-en.
[58] OSPAR Commission (n.d.), “Beach litter monitoring: Characteristics of litter in the marine and coastal environment”, https://oap.ospar.org/en/ospar-assessments/committee-assessments/eiha-thematic-assessments/marine-litter/beach-litter-monitoring.
[11] Palacios‐Abrantes, J. et al. (2022), “Timing and magnitude of climate‐driven range shifts in transboundary fish stocks challenge their management”, Global Change Biology, Vol. 28/7, pp. 2312-2326, https://doi.org/10.1111/gcb.16058.
[12] Parker, R. et al. (2018), “Fuel use and greenhouse gas emissions of world fisheries”, Nature Climate Change, Vol. 8/4, pp. 333-337, https://doi.org/10.1038/s41558-018-0117-x.
[18] Parker, R. et al. (2015), “Environmental and economic dimensions of fuel use in Australian fisheries”, Journal of Cleaner Production, Vol. 87, pp. 78-86, https://doi.org/10.1016/j.jclepro.2014.09.081.
[44] Pascual, M. et al. (2016), “Socioeconomic impacts of marine protected areas in the Mediterranean and Black Seas”, Ocean & Coastal Management, Vol. 133, pp. 1-10, https://doi.org/10.1016/j.ocecoaman.2016.09.001.
[27] Pikitch, E. et al. (2004), “Ecosystem-based fishery management”, Science, Vol. 305/5682, pp. 346-347, https://doi.org/10.1126/science.1098222.
[22] Prellezo, R. (ed.) (2021), “Factors affecting greenhouse gas emissions in fisheries: Evidence from Iceland’s demersal fisheries”, ICES Journal of Marine Science, Vol. 78/7, pp. 2385-2394, https://doi.org/10.1093/icesjms/fsab109.
[31] Read, A., P. Drinker and S. Northridge (2006), “Bycatch of marine mammals in US and global fisheries”, Conservation Biology, Vol. 20/1, pp. 163-169, https://doi.org/10.1111/j.1523-1739.2006.00338.x.
[43] Rees, S. et al. (2021), “An evaluation of the social and economic impact of a marine protected area on commercial fisheries”, Fisheries Research, Vol. 235, p. 105819, https://doi.org/10.1016/j.fishres.2020.105819.
[41] Rees, S. et al. (2018), “Bridging the divide: Social-ecological coherence in marine protected area network design”, Aquatic Conservation: Marine and Freshwater Ecosystems, Vol. 28/3, pp. 754-763, https://doi.org/10.1002/aqc.2885.
[30] Regular, P. et al. (2013), “Canadian fishery closures provide a large-scale test of the impact of gillnet bycatch on seabird populations”, Biology Letters, Vol. 9/4, p. 20130088, https://doi.org/10.1098/rsbl.2013.0088.
[54] Richardson, K. et al. (2022), “Global estimates of fishing gear lost to the ocean each year”, Science Advances, Vol. 8/41, https://doi.org/10.1126/sciadv.abq0135.
[52] Richardson, K. et al. (2021), “Global causes, drivers, and prevention measures for lost fishing gear”, Frontiers in Marine Science, Vol. 8, https://doi.org/10.3389/fmars.2021.690447.
[53] Richardson, K., B. Hardesty and C. Wilcox (2019), “Estimates of fishing gear loss rates at a global scale: A literature review and meta‐analysis”, Fish and Fisheries, Vol. 20/6, pp. 1218-1231, https://doi.org/10.1111/faf.12407.
[36] Sala, E. et al. (2018), “Assessing real progress towards effective ocean protection”, Marine Policy, Vol. 91, pp. 11-13, https://doi.org/10.1016/j.marpol.2018.02.004.
[9] Tittensor, D. et al. (2021), “Next-generation ensemble projections reveal higher climate risks for marine ecosystems”, Nature Climate Change, Vol. 11/11, pp. 973-981, https://doi.org/10.1038/s41558-021-01173-9.
[55] UNEP (2016), Marine Plastic Debris and Microplastics: Global Lessons and Research to Inspire Action and Guide Policy Change, United Nations Environment Programme, Nairobi, https://wedocs.unep.org/20.500.11822/7720.
[35] UNEP-WCMC and IUCN (2021), Protected Planet: The World Database on Protected Areas (WDPA) and World Database on Other Effective Area-based Conservation Measures (WD-OECM), http://www.protectedplanet.net.
[65] van Nijen, J. (2021), “How to come to a more circular (management) system of fishing gear”, Dutch Ministry of Infrastructure and Water Management, WVL/BN REM, https://www.noordzeeloket.nl/publish/pages/189036/report-how-to-come-to-a-more-circular-management-system-of-fishing-gear-in-the-ospar-region_.pdf.
[19] Waldo, S. and A. Paulrud (2016), “Reducing greenhouse gas emissions in fisheries: The case of multiple regulatory instruments in Sweden”, Environmental and Resource Economics, Vol. 68/2, pp. 275-295, https://doi.org/10.1007/s10640-016-0018-2.
[45] White, C. et al. (2008), “Marine reserve effects on fishery profit”, Ecology Letters, Vol. 11/4, pp. 370-379, https://doi.org/10.1111/j.1461-0248.2007.01151.x.
[71] Wilcox, C. et al. (2016), “Using expert elicitation to estimate the impacts of plastic pollution on marine wildlife”, Marine Policy, Vol. 65, pp. 107-114, https://doi.org/10.1016/j.marpol.2015.10.014.
[61] WTO (2021), Informal Dialogue on Plastic Pollution and Environmentally Sustainable Plastics Trade (IDP) Ministerial Statement on Plastic Pollution and Environmentally Sustainable Plastics Trade, World Trade Organization, Geneva, https://docs.wto.org/dol2fe/Pages/SS/directdoc.aspx?filename=q:/WT/MIN21/8R2.pdf&Open=True (accessed on 8 June 2022).
[66] Yamaguchi, S. (2018), “International trade and the transition to a more resource efficient and circular economy: A concept paper”, OECD Trade and Environment Working Papers, No. 2018/03, OECD Publishing, Paris, https://doi.org/10.1787/847feb24-en.
[78] Ye, Y. et al. (2012), “Rebuilding global fisheries: The World Summit goal, costs and benefits”, Fish and Fisheries, Vol. 14/2, pp. 174-185, https://doi.org/10.1111/j.1467-2979.2012.00460.x.
[21] Ziegler, F. et al. (2016), “Same stock, different management: Quantifying the sustainability of three shrimp fisheries in the Skagerrak from a product perspective”, ICES Journal of Marine Science, Vol. 73/7, pp. 1806-1814, https://doi.org/10.1093/icesjms/fsw035.
Notes
← 1. SDG 14.4 states: “By 2020, effectively regulate harvesting and end overfishing, illegal, unreported and unregulated fishing and destructive fishing practices and implement science-based management plans, in order to restore fish stocks in the shortest time feasible, at least to levels that can produce maximum sustainable yield as determined by their biological characteristics.” Aichi Target 6 states: “By 2020, all fish and invertebrate stocks and aquatic plants are managed and harvested sustainably, legally and applying ecosystem based approaches, so that overfishing is avoided, recovery plans and measures are in place for all depleted species, fisheries have no significant adverse impacts on threatened species and vulnerable ecosystems and the impacts of fisheries on stocks, species and ecosystems are within safe ecological limits.”
← 2. In advocating a precautionary approach to fisheries management, the FAO Code of Conduct for Responsible Fisheries (FAO, 1995[75]) recommends: “[…] on the basis of the best scientific evidence available, inter alia, determin[ing]: a) stock-specific target reference points, and, at the same time, the action to be taken if they are exceeded; and b) stock-specific limit reference points, and, at the same time, the action to be taken if they are exceeded; when a limit reference point is approached, measures should be taken to ensure that it will not be exceeded.”
← 5. The FAO data set differs from the OECD data in that it does not have an undetermined category. This is because the FAO data are based on number of reference stocks, where information is collected by the FAO itself and decisions regarding the status are taken by the FAO on the basis of available data, even if the data are suboptimal (for the detailed methodology, see Ye et al. (2012[78])). Stocks in good health in the OECD data set are the equivalent of underfished and maximally sustainably fished stocks in the FAO data. However, overfished stocks (35.4%) in the FAO data do not perfectly correspond to undetermined stocks and stocks falling below sustainability standards in the OECD data (36%), as this would imply all undetermined stocks are overfished, which is unlikely. However, undetermined stocks are probably a mix of both healthy stocks and stocks not meeting sustainability standards, suggesting the proportions of both are underestimated in the current data.
← 6. Pikitich et al. (2004[27]) define the goals of EBFM as: “(i) avoid degradation of ecosystems, as measured by indicators of environmental quality and system status; (ii) minimise the risk of irreversible change to natural assemblages of species and ecosystem processes; (iii) obtain and maintain long-term socioeconomic benefits without compromising the ecosystem; and (iv) generate knowledge of ecosystem processes sufficient to understand the likely consequences of human actions.”
← 7. Data on landings value were not available for Japan for 2020. Data for 2019 were used instead.
← 8. Ghost gear is sometimes also referred to as abandoned, lost or otherwise discarded fishing gear. It also occurs in lakes and rivers, but this report only considers marine-based ghost gear.
← 9. Fishing gear loss also comes at significant direct costs for the industry – with the direct cost of replacing the lost gear and the opportunity costs of losing the fish that had been caught and of losing fishing opportunities until the gear is replaced.
← 10. Gear loss also comes at significant direct costs for the industry, such as the cost of replacing the lost gear, the loss of the fish that had been caught and the opportunity cost of not being able to fish until the gear is replaced. Ghost gear also implies costs for other sectors of the blue economy – notwithstanding the cost to society of deteriorated ocean ecosystems. For example, the shipping sector is affected by the risk of entanglement with vessel propellers while coastal tourism is affected by the impacts of litter on the aesthetic value of natural areas. Ghost fishing gear has even been reported to have contributed to the loss of human lives when it contributed to vessel sinking (Cho, 2005[72]).
← 11. For instance, a study conducted in the Salish Sea estimates that 4.5% of the value of the Dungeness crab harvest is lost annually to ghost fishing (Antonelis et al., 2011[77]).
← 12. A review of evidence on encounters between marine animals and marine plastic debris conducted by Gall and Thompson (2015[70]) suggests that 79% of entanglement cases documented resulted in the injury or death of the animals, while only 4% of plastic ingestion cases had such a detrimental impact. The same study estimated that over two-thirds of entanglement incidents were caused by fishing gear, such as plastic ropes and netting. Another impact study of the most commonly found forms of marine plastics debris found that fishing gear would have four times more impact on marine mammals, turtles and seabirds through entanglement than all other forms of marine debris combined (Wilcox et al., 2016[71]).
← 13. Field and laboratory studies have detected ingestion of microplastics by commercially fished species (OECD, 2021[51]). Microplastics can have both physical and chemical toxicity on marine organisms and humans. Chemical impacts for humans may include endocrine disruptions, carcinogenicity and effects on reproductive health (OECD, 2021[6]). Other potential effects observed on marine biota include altered feeding behaviour, liver toxicity, tumour promotion and reduced survival (GESAMP, 2016[73]). The physical toxicity of ingested microplastics on humans remains largely unknown and more research is needed, but some research already suggests that systemic exposure to microplastics ingestion may lead to localised effects on the immune system, inflammation of the gut and intestine irritation (OECD, 2021[51]).
← 14. The reviewed literature included 68 papers spanning 32 countries and territories across the Atlantic, Indian, Pacific and Southern Oceans and the Baltic, Caribbean and Mediterranean Seas. Publications were generally more biased to the United States and Europe, and toward pot and net fisheries, with limited literature for line fisheries.
← 15. For example, Kuczenski (2021[74]) recently made use of automated identification system data from Global Fishing Watch, previous estimates of gear losses by type (Richardson, Hardesty and Wilcox, 2019[53]) and predictive modelling to estimate the amount of ghost gear entering the ocean to about 48 000 t annually. However, this figure derives from an estimation focusing on industrial trawl, long line and purse seine fisheries only. The study, in particular, does not consider ghost gear from gillnets, fish aggregating devices, or traps and pots, all of which make up the top three most likely gear classes to be lost according to the Global Ghost Gear Initiative’s Best Practice Framework for the Management of Fishing Gear. The study also ignores smaller commercial and artisanal fisheries.
← 16. The IDP currently has 16 co-sponsors and is open to all WTO members. The aim of the group is to complement discussions in the WTO Committee on Trade and Environment and other fora. Key topics being discussed improving transparency; monitoring trade trends; promoting best practices; strengthening policy coherence; identifying the scope for collective approaches; assessing capacity and technical assistance needs; and co-operating with other international processes and efforts (WTO, 2021[61]).
← 17. See Resolution 14 adopted by the United Nations Environment Assembly at its fifth session on 2 March 2022: “End plastic pollution: Towards an international legally binding instrument”.
← 18. The Global Ghost Gear Initiative has developed a best practice framework for the management of fishing gear (Global Ghost Gear Initiative, 2022[68]). Examples of retrieval, collecting and recycling projects are described in Global Ghost Gear Initiative (2018[76]).
← 19. See the Climate and Environment Ministers’ Communiqué.
← 20. The International Convention for the Prevention of Pollution from Ships (MARPOL) is the main international convention covering the prevention of pollution of the marine environment by ships from operational or accidental causes. MARPOL includes regulations aimed at preventing and minimising pollution from ships – both accidental pollution and that from routine operations – and currently includes six technical annexes. Adoption: 1973 (Convention), 1978 (1978 Protocol), 1997 (Protocol – Annex VI); entry into force: 2 October 1983 (Annexes I and II).