This chapter presents policy options to tackle endocrine disruptors in water bodies, drinking water and wastewater. It documents policy approaches that intervene throughout the life cycle of endocrine disrupting chemicals (EDCs) at the source, during use, and at the end-of-pipe. In addition, it proposes interventions that are centred around the adverse effects of EDCs. Effect-centred approaches are well-suited to respond to emerging monitoring methods, such as bioassays, that do not instantly identify the culprit chemical. Lastly, this chapter makes the case for international actions as the adverse impacts of EDCs are a global concern in need of global solutions.
Endocrine Disrupting Chemicals in Freshwater
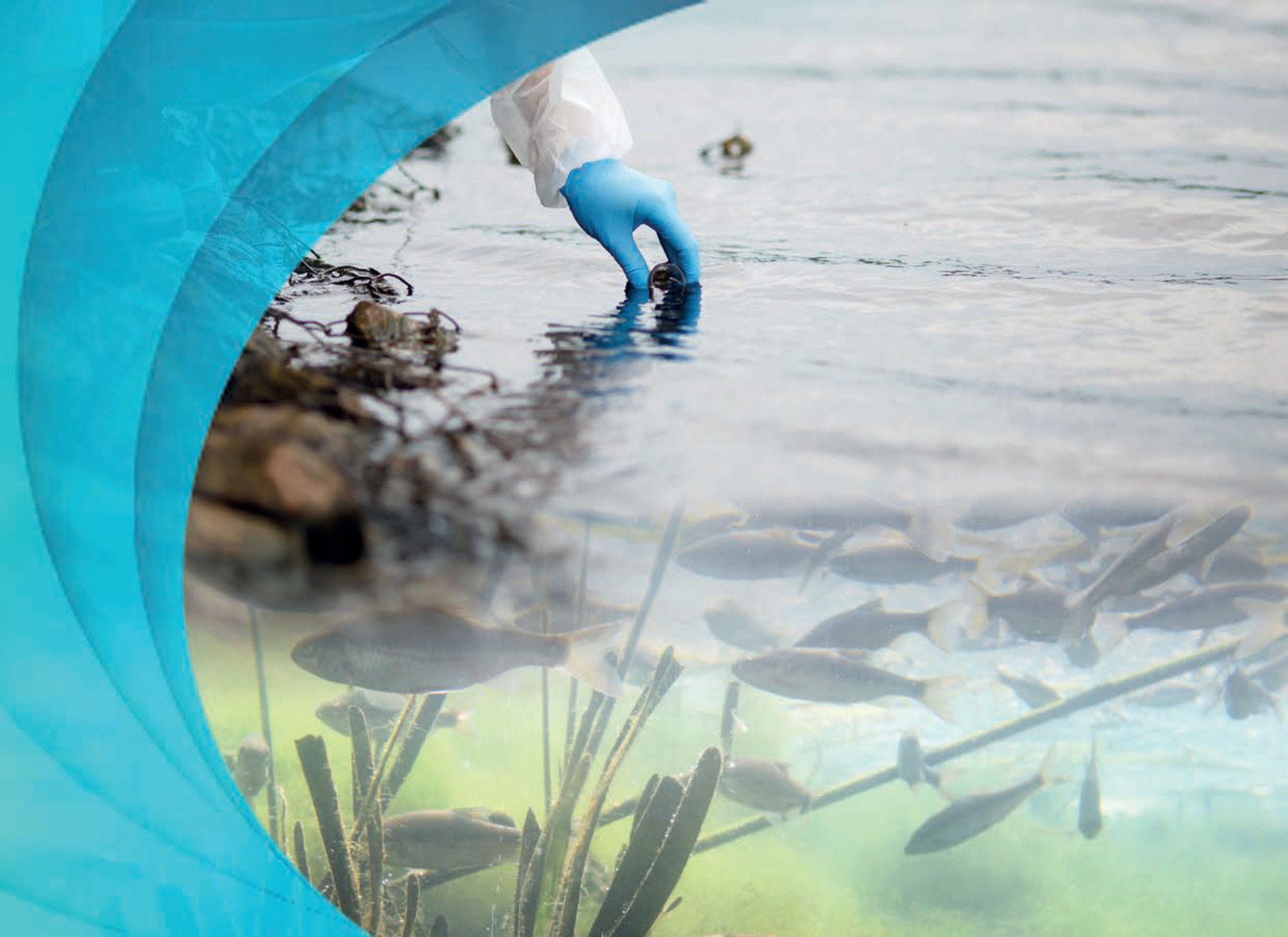
3. Policy options to reduce and manage endocrine disruption in freshwater
Abstract
3.1. Introduction
As presented in Chapter 1, endocrine disrupting chemicals (EDCs) are compounds that can disrupt the endocrine system and cause adverse effects in intact organisms or their offspring. EDCs are produced by various industries (e.g., pharmaceuticals, pesticides, personal care products, electronics) and will eventually make their way into the environment where they can impact human health and ecosystem integrity. Since EDCs are emitted from different sources, monitoring EDCs in freshwater is important to prioritise mitigation actions. Monitoring methods have been discussed in Chapter 2, which recommends supplementing chemical analysis with bioassays and other methods where appropriate and applicable.
This chapter presents the different policy options that can be used to address EDCs, supported by country case studies. It takes into consideration that some EDCs are unknown, whilst endocrine disrupting effects have already been observed in freshwater. This chapter pays particular attention to measures that can support a further diffusion of the test methods reviewed in Chapter 2.
Section 3.2 discusses principles that underlie policy decisions, such as principles on the accepted level of risk or hazard. Section 3.3 documents existing policy approaches that intervene throughout the life cycle of EDCs at the source, during use, and at the end-of-pipe. Section 3.4 proposes interventions that are centred around the adverse effects of EDCs. Effect-centred approaches are well-suited to respond to emerging monitoring methods that do not instantly identify the culprit chemical, such as bioassays. Lastly, Section 3.5 makes the case for international actions as EDCs are a global concern in need of global solutions.
3.2. Principles underpinning policy decisions
The type of policy action is preceded by an agreed approach to the accepted level of risk. This is ultimately a political decision, informed by societal debate. Such a debate is particularly important for EDCs, as some substances are suspected of having endocrine active properties, but this may not be acknowledged or established with certainty. The same holds true for mixture effects. Moreover, endocrine disruptive substances fall under different legislative spheres, which may limit the toolbox of water regulators in addressing (suspected) endocrine disrupting effects in water (Figure 3.1).
Figure 3.1. Regulations relevant to EDCs and their impacts on water quality
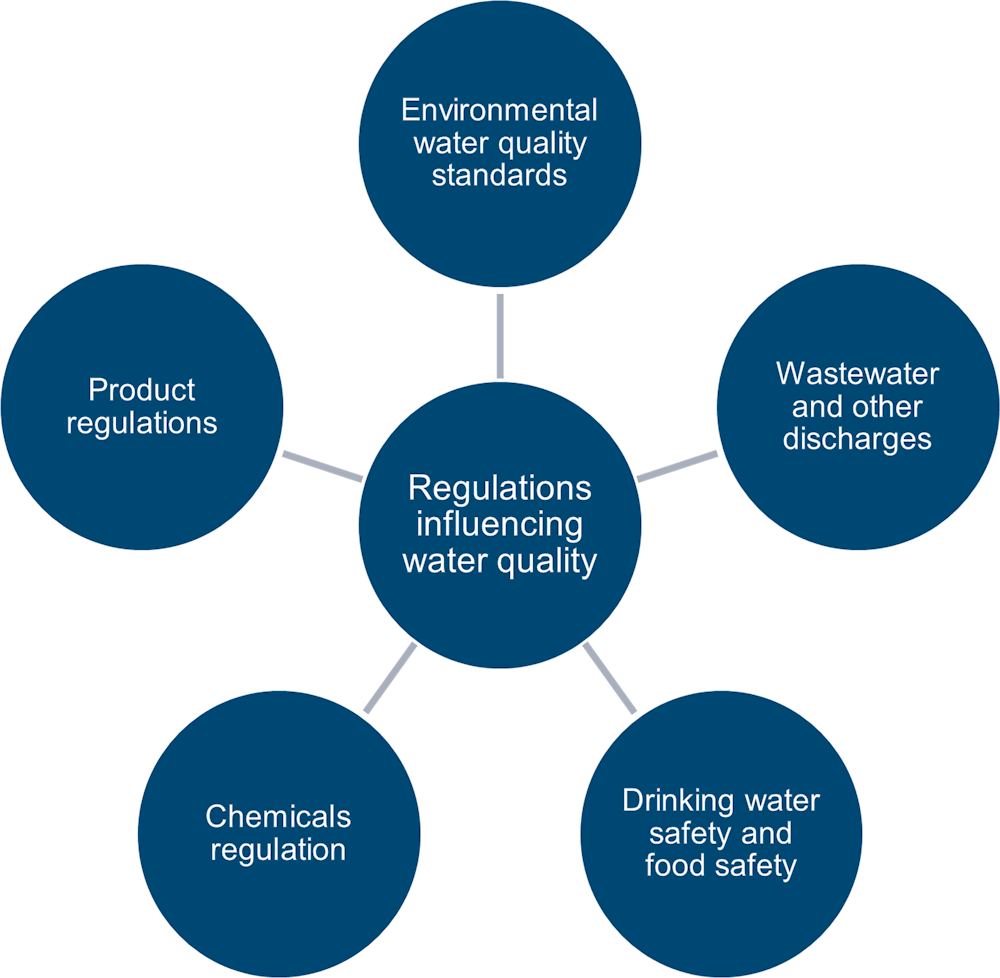
Note: Product regulations can cover a vast array of products, e.g., agricultural inputs, cosmetics and personal care products, pharmaceuticals.
Source: Authors
3.2.1. Principles that guide decision-making under uncertainty
Uncertainty is inherent to risk management of chemicals. Policy principles can provide guidance on decision-making under uncertainty. The types of uncertainty vary, depending on the compound and policy approach. It can include uncertainty in hazard or exposure assessments, uncertainty in the economic costs and benefits of a decision, uncertainty in the enforcement and effectiveness of the risk management approach, uncertainty in the safety of a substitution, and others (OECD, 2022[1]).
A more proactive policy approach, based on the precautionary principle, could be considered when the environmental and human health risks are uncertain and the potential consequences of inaction are high. For example, it is worth considering a precautionary policy approach when there is a long-term risk to the environment, health, or the economy. The damage caused at the population and ecosystem levels can take years to repair and can be experienced across generations. This is particularly relevant to EDCs.
Risk versus hazard approaches
Chemicals can be managed based on risk or hazard. Decisions of chemicals management can be guided by the adverse impact of the chemical regardless of human or wildlife exposure to the chemical. This so-called hazard-based decision-making “focuses on addressing the inherent hazards of chemicals through substitution or other approaches, rather than calculating an acceptable level of risk” (UNEP, 2019[2]). The alternative is a risk-based approach, where decisions are prioritised based on exposure to the chemical. “This includes identifying use patterns that may create widespread exposure across a population, or intense exposure for a subset of the population” (UNEP, 2019[2]).
Hazard-based approaches to meet water quality objectives are best achieved through chemical related regulation. Realistically, the water community has limited control over the governance of substances and products, and water policies often enter the ‘regulatory stage’ at the end-of-life of substances. Chemical related regulations are the gatekeeper of chemicals on the market, and product regulations determine their use. Implementing a hazard approach is even more complex in transboundary contexts, as water is a recipient of wastes and chemicals from other jurisdictions with different regulations or enforcement. Moreover, the impacts can occur decades after the phasing out of chemicals, as some EDCs are legacy chemicals that can persist in the aquatic environment, such as in sediments (Kurek et al., 2019[3]). Nevertheless, hazard-based approaches could be appropriate in drinking water production, wastewater reuse, or for the protection of critical ecosystems. Additional treatment can be justified based on the precautionary principle.
Water regulation traditionally uses risk-based approaches. The water community can set the acceptable level of risk and the tolerated concentrations in water, for example by setting water quality standards or effluent standards. Water quality standards are based on different parameters, such as predicted no effect concentrations (PNEC): concentrations at which there are no predicted effects to humans, aquatic organisms, or secondary poisoning of predators. When a risk is uncertain, the accepted level of risk can be lowered out of precaution, such as by specifying an additional assessment factor to existing environmental quality standards that reflect the endocrine properties, the risk of mixtures and any potential uncertainty of the chemical (James, Kroll and Minier, 2023[4]). Box 2.12 in Chapter 2 presents a methodology to take the risk of endocrine disruption into consideration in environmental quality standards.
However, risks from EDCs in water to human health and ecosystems remain difficult to quantify. Given the large number of compounds present in the aquatic environment, prioritisation frameworks can support the selection of substances to monitor and regulate. Table 3.1 presents a prioritisation framework developed by the NORMAN Network, and similar prioritisation frameworks have been developed (Götz et al., 2009[5]; Johnson et al., 2017[6]; Gaston et al., 2019[7]). Follow-up actions for monitoring and assessment are based on the state of knowledge of a specific substance (Dulio and von der Ohe, 2013[8]; von der Ohe et al., 2011[9]). Bioassays, combined with effect-directed analysis, can support the prioritisation of contaminants (Smital et al., 2012[10]). These methods have been described in detail in Chapter 2.
Table 3.1. Prioritisation of problematic EDCs in freshwater: determining actions based on the state of knowledge of hazards
Category |
Description of the state of knowledge on the hazard of a substance |
Appropriate action for priority substances within category |
---|---|---|
A |
Substances for which there is sufficient evidence of exposure and adverse effects at environmental concentration |
Integration in routine monitoring and derivation of legally binding environmental quality standards |
B |
Substances for which hazard assessment is based on experimental data BUT few monitoring data |
Screening studies for information about current exposure |
C |
Substances for which there is evidence of exposure BUT hazard assessment is based on predicted toxicity (PNEC) |
Rigorous hazard assessment |
D |
Substances for which hazard assessment is based on experimental data BUT analytical capabilities are not yet satisfactory |
Improvement of analytical methods required |
E |
Substances for which no or few monitoring data AND hazard assessment is based on predicted toxicity (PNEC) |
Screening studies AND rigorous hazard assessment |
F |
Substances for which toxicity data are sufficient for the derivation of an EQS and there is evidence that the exposure does not pose a hazard to ecosystems |
Monitoring efforts for these compounds could be reduced1 |
Note: Based on the NORMAN Prioritisation framework for emerging substances. Note that actions should only be applied to a shortlist of prioritised chemicals within each category.
Note1: Reducing monitoring might have a negative consequence if the investigated or suspected EDC leads to severe effects in mixtures with other chemicals.
Source: Adapted from (Dulio and von der Ohe, 2013[8])
A hierarchy of policy principles
The following hierarchy of OECD principles can usefully guide the development of policy for the management of diffuse pollution sources. They are captured by the OECD Council Recommendation on Water (OECD, 2016[11]):
Principle of pollution prevention – prevention of pollution is often more cost-effective than treatment and restoration.
Principle of treatment at source – treatment at the earliest stage possible is generally more effective and less costly than waiting until pollution is widely dispersed.
Polluter pays principle – makes it costly for those activities that generate pollution and provides an economic incentive for reducing the pollution.
Beneficiary pays principle – allows sharing of the financial burden with those who benefit from water quality improvements. Minimum pollution regulations must be met to first ensure additionality and avoid rewarding polluters.
In addition, environmental justice is a relevant guiding principle in the case of EDCs, as some subpopulations may be more affected than others. Section 3.4.4, on minimising the impacts of EDCs on vulnerable populations, discusses this in further detail. Environmental justice can comprise (OECD, 2017[12]):
Distributive justice - fair treatment in terms of access to natural resources, environmental services fand benefits, and environmental risk exposure.
Corrective justice - accountability and remediation for environmental harm.
Procedural justice - access to environmental information, judicial and administrative proceedings and participation in environmental decision making.
3.3. Policies that address the life cycle of endocrine disrupting chemicals
Policy recommendations
EDCs can be found in multiple classes of chemicals, e.g., natural and artificial hormones, pesticides, plasticisers, and flame retardants. They are released into freshwater throughout the life cycle of the chemical from its production, distribution, usage to its disposal. A policy mix that addresses all steps of the life cycle of EDCs could have the following design:
At the source, Environmental Protection Agencies, water authorities, river basin organisations and water service providers can support initiatives that decrease the identification time of EDCs, by turning to new approach methods (NAMs). NAMs include methods such as high-throughput in vitro screening, omics and in silico methods such as Quantitative structure-activity relationship (QSAR). Group-wise assessments can also help increase the efficiency of EDC assessment and simultaneously reduce animal use for the chemical assessment. The forementioned water entities have a role to play in sharing monitoring results and raising issues relevant to (emerging) water risks.
Water authorities would benefit from stimulating and getting involved in use-orientated initiatives even if these are not directly linked to the water sector, such as waste disposal campaigns, consumer awareness campaigns, labelling schemes, and private sector initiatives. User decisions, even if motivated by personal health reasons, co-benefit the environment.
End-of-pipe measures should only be used in conjunction with source-directed and use-orientated measures. An over-emphasis on upgrading wastewater treatment infrastructure is not a sustainable, optimal use of limited financial, technical and natural resources. Regulators could prioritise more stringent treatment standards to those discharges that pose a particular pressure to health or ecosystems. Regulators and service providers could consider advanced treatment and monitoring with bioassays for wastewater reuse and sewage sludge recovery infrastructure.
Regulators could consider making use of existing public databases when issuing discharge permits, such as EDLists.org, the Endocrine Active Substances Information System, Database of Endocrine Disrupting Chemicals and their Toxicity Profiles. The databases inform about the suspected or confirmed endocrine disrupting properties of released substances. Assessments based on the grouping of chemicals can also inform water discharge permits. Water permits could include a condition for additional monitoring requirements when there is a suspected risk of EDCs being released in the environment.
There are several mitigation options in the EDC life cycle that contribute to water quality improvement at the source, during use and at the end-of-pipe (Figure 3.2). Source-directed approaches impose, incentivise or encourage measures that prevent the release of EDCs into water bodies. A focus on preventive options early in an EDC life cycle, may deliver the most long-term and large-scale benefits. This, however, requires an acceleration of chemicals assessment or increased adoption of the precautionary principle. Use-orientated policy approaches include policy instruments which impose, incentivise, or encourage a reduction in the use of EDCs and their release to the environment. Users of EDC’s, such as consumers or the agricultural sector, have a role to play in making choices on the use and disposal of EDCs. Some users avoid using EDCs for personal health reasons. Such decisions co-benefit the environment as less waste is generated and accumulated. End-of-pipe measures focus on removing or eliminating EDCs after their use or release into water. End-of-pipe policies involve different types of instruments that impose, incentivise, or encourage improved wastewater treatment and solid waste disposal. Relying solely on end-of-pipe measures, such as WWTP upgrades, can be costly, energy intensive and toxic transformation products may be formed. However, in combination with source-directed and use-orientated approaches, extra treatment at the level of WWTPs play a role in reducing EDCs in the aquatic environment. Buffer zones or wetlands are promising nature-based solutions in capturing some EDCs before their release into the aquatic environment.
Regulatory, economic and voluntary policy instruments are all part of the policy toolkit that is needed to manage multiple sources of pollution throughout the life cycle of EDCs (OECD, 2016[11]; OECD, 2019[13]). Figure 3.1 presents a mix of these policy instruments, in no particular order.
Figure 3.2. Selected life cycle instruments that contribute to water quality improvements
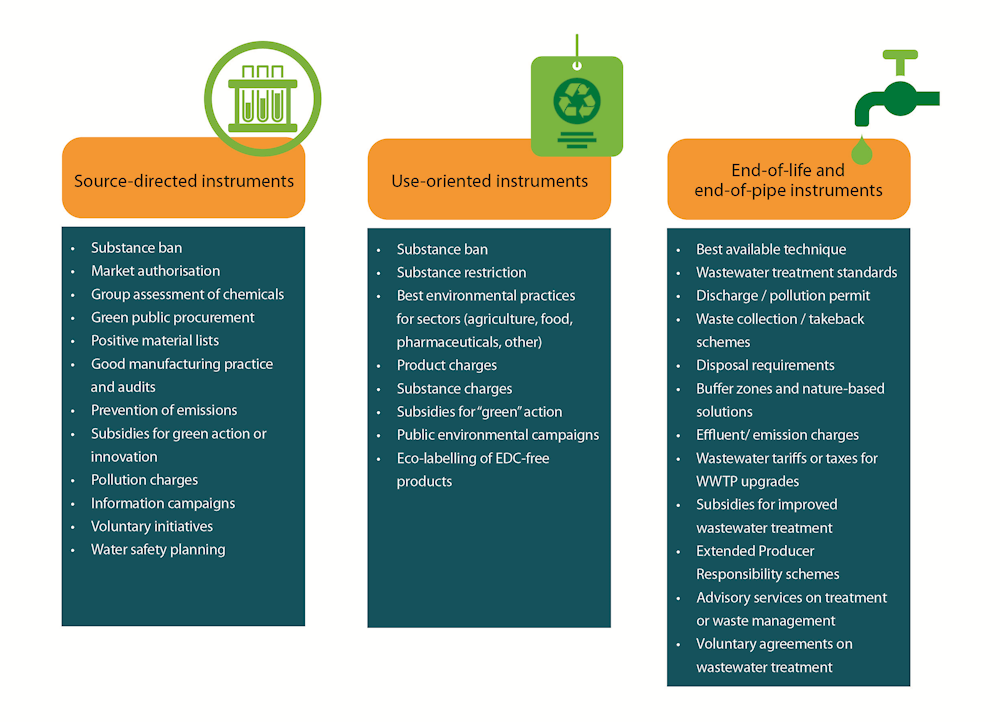
Note: The presented instruments are non-exhaustive
Source: Adapted from (OECD, 2017[14]; OECD, 2019[13]; OECD, 2021[15]; OECD, 2022[1]; OECD, 2023[16])
The difficulty with managing the life cycle of EDCs is that, first, many chemicals are not identified as endocrine disrupting or endocrine active even though effects have been detected in water, and second, EDCs comprise numerous product groups (Table 3.2). Addressing the life cycle of each of these sources and uses is beyond the scope of this publication. This report therefore refers to the policy recommendations made in other recent OECD reports on contaminants of emerging concern, which are equally relevant and applicable in the case of EDCs. Table 3.2 provides an overview of the OECD reports that cover in more detail the measures to manage the life cycle of specific substances or product groups. The following paragraphs highlight three policy approaches particularly relevant to EDCs: A source-directed approach: Risk assessment of substances and products, A use-oriented approach: Labelling consumer products, End-of-pipe measures: Wastewater reuse and sewage sludge recovery.
Table 3.2. Relevant OECD resources on managing the life cycle of substances and product groups
Substance or product group |
Relevant OECD policy studies |
---|---|
Consumer products (e.g., children’s products, electronics, textiles) |
Policies to reduce microplastics pollution in water (OECD, 2021[15]) |
Cosmetics, personal care products |
Pharmaceutical residues in freshwater (OECD, 2019[13]) |
Food contact materials (e.g., plastic food containers, food wrappers, baby bottles) |
Workshop report on flexible food-grade plastic packaging (OECD, 2023[16]) |
Industrial chemicals |
Government risk management approaches used for chemicals management (OECD, 2022[1]) |
Metals |
Government risk management approaches used for chemicals management (OECD, 2022[1]) |
Pesticides |
Diffuse pollution, degraded waters (OECD, 2017[14]) |
Pharmaceuticals (for humans and livestock) |
Pharmaceutical residues in freshwater (OECD, 2019[13]) |
Synthetic and naturally occurring hormones |
Pharmaceutical residues in freshwater (OECD, 2019[13]) |
Note: Even though the above-mentioned reports are not specific to EDCs, they present relevant source-directed, use-oriented or end-of-pipe measures of product groups that can end up as endocrine active or endocrine disrupting pollutant in freshwater
3.3.1. A source-directed approach: Risk assessment of substances and products
This section explores how EDCs are assessed and how the water community could contribute to the prioritisation of substances. Chemical related legislation is the gatekeeper to chemicals entering the market, by assessing whether a substance poses a risk to function(s) of the endocrine system. Source-directed approaches are therefore dependent on risk assessments of substances, and specific regulations for consumer products, agriculture and pharmaceuticals.
Risk assessments and chemicals management are promising source-based policy measures. They are best combined with other source-directed policy instruments that reduce the production of EDCs (listed in Figure 3.2).
A first approach to reduce EDCs in the environment is to evaluate their risks to health and biodiversity. To ensure science-based regulation of EDCs, various countries and regions have developed frameworks for the evaluation of the endocrine active or disrupting properties of chemicals (IPCP, 2017[17]). Those include programs such as the Endocrine Disruptor Screening Program (EDSP) by the US-EPA (EDSTAC, 1998[18]), the Extended Tasks on Endocrine Disruption (EXTEND) by Japan’s Ministry of the Environment (MoE) (Box 3.1) and the EU guidance for the identification of EDCs under the REACH (Andersson et al., 2018[19]). To provide guidance on evaluation of chemicals for endocrine disruption, the OECD continuously develops and standardises test guidelines for the identification of EDCs (OECD, 2018[20]).
While those programs are well described and OECD guidance documents are available (OECD, 2018[20]), it is still important to mention that those processes are data- and time-intensive. The US-EPA’s EDSP illustrates the time-intensity of the EDC screening process (Maffini and Vandenberg, 2022[21]; U.S. EPA, 2021[22]). It started the evaluation of 52 chemicals in 2005. In 2015, all 52 chemicals were analysed through the EDSP first Tier of testing (U.S. EPA, n.d.[23]). From those 52, 18 were recommended for further testing in Tier 2, of which the results are still pending. In 2013, a second list of 109 chemicals was prepared for testing, containing 41 pesticides ingredients and 68 chemicals targeted by the Safe Drinking Water Act (U.S. EPA, 2013[24]).
Many countries aim to decrease the identification time by turning to new approach methods (NAMs) which include methods like high-throughput in vitro screening, omics and in silico methods such as Quantitative structure-activity relationship (QSAR). Examples are the ToxCast and Tox21 programmes in the US (Dix et al., 2007[25]; Krewski et al., 2010[26]), and the EU-ToxRisk and ONTOX (Daneshian et al., 2016[27]; Vinken et al., 2021[28]). Other countries, such as Japan (Box 3.1) are evaluating the appropriate use of NAMs, as challenges remain in deviating from traditional toxicological risk assessment approaches. The use of Integrated Approaches for Testing and Assessment (IATAs) can help to combine the information gathered from different methods. The OECD provides guidance and case studies on the use of IATAs (OECD, 2017[29]).
While IATAs and NAMs can provide quick information, challenges remain in advancing evaluation of EDCs. For one, the common definition of EDCs states that an EDC “consequently causes adverse health effects in an intact organism, or its progeny, or (sub)populations” (WHO-UNEP, 2013[30]). This requires proof of adverse effects with high weight-of-evidence. The data acquired need to be able to show that the chemical or mixture (1) generates adverse effects in an intact organism or its progeniture, (2) acts via an endocrine mode of action and (3), that the adverse effects is caused by the endocrine mode of action (Kassotis et al., 2020[31]; James, Kroll and Minier, 2023[4]). It is worth pointing out that for routine water quality monitoring, as presented in Chapter 2, whole animal testing overshoots the intended purpose of establishing a risk profile of the chemical present in the water sample.
Group-wise assessment of chemicals can help increase the efficiency of EDC assessment while reducing animal use. Some chemicals are analogues: similar structures may ignite similar biological activities (Swedish Government Inquiries, 2019[32]; OECD, 2017[33]). The grouping of those similar chemicals can be justified with tools such as QSAR. Group-wise assessments can be done to avoid assessing every endpoint for every chemical. Furthermore, this could help prevent harmful substitutions for which acquiring a sufficient knowledge base to enable regulation can take years. As an example, the European Chemicals Agency grouped 148 bisphenols for risk assessment and recommended the restriction of 30 of them in relation to their potential of disrupting the endocrine system and causing reprotoxic effects (ECHA, 2021[34]). To learn more about the grouping of chemicals, please refer to the OECD guidance on the subject (OECD, 2017[33]). Assessments based on grouping chemicals can also inform water discharge permits, for example by including a condition for additional monitoring when there is a suspected risk of EDCs being released in the environment.
Water managers have access to public databases that inform about the suspected or confirmed endocrine disrupting properties of a substance. Such databases can support on the prioritisation of problematic EDCs in activities such as monitoring, permitting and designing policy interventions. The website EDLists.org, initiated by Belgium, Denmark, France, the Netherlands, Spain and Sweden, informs stakeholders about the current status of substances identified as endocrine disruptors or suspected of having endocrine disrupting properties (edlists.org, n.d.[35]) Other databases compile available toxicity data on EDCs and EASs, such as the Endocrine Active Substances Information System (European Commission, 2022[36]) and the Database of Endocrine Disrupting Chemicals and their Toxicity Profiles (Institute of Mathematical Sciences, n.d.[37]) (Karthikeyan et al., 2019[38]; Karthikeyan et al., 2021[39]).
Box 3.1. Japan’s Extended Tasks on Endocrine Disruption 2022
Already in 1998, Japan’s Ministry of Environment (MoE) put itself at the forefront of EDC research and method development with the launch of the Strategic Programs on Environmental Endocrine Disruptors ’98 (SPEED’98) (MoE Japan, 1998[40]). The programme was followed by Extended Tasks on Endocrine Disruption (EXTEND) 2005, 2010, 2016 and 2022 (MoE Japan, 2005[41]; MoE Japan, 2010[42]; MoE Japan, 2016[43]; MoE Japan, 2022[44]).
Over the years, the programme led to the development of knowledge of the presence of EDCs in Japan, as well as the development of methods for the identification of EDCs. Those methods include the OECD Test Guideline 240 for the Medaka Extended One Generation Reproduction Test (MEOGRT) (OECD, 2018[45]) and OECD Test Guideline 241 on the Larval Amphibian Growth and Development Assay (LAGDA) (OECD, 2018[46]) developed in collaboration with the US.
Moreover, in EXTEND 2016, Japan implemented its 2-Tier framework to assess EDCs with in vitro bioassays as pre-screening, followed by short-term in vivo bioassays (Tier 1). Tier 2 applies long-term in vivo bioassays to confirm positive results obtained in Tier 1. All the bioassays used in the framework have been developed by Japan to assess effects in non-mammalian species (fish, frog, daphnia). Through this framework, over 200 substances detected in Japanese aquatic environment have been tested with at least in vitro bioassays in Tier 1. Furthermore, six substances have been fully evaluated with the MEOGRT. Through its programme on EDCs, Japan is also involved in international initiatives such as developing OECD Test Guidelines and international research programmes.
The EXTEND 2022 objectives include:
Assessment of effect and method development
Monitoring of environmental concentrations of EDCs and exposure assessment
Risk assessment and management
Collection of data and improving knowledge
International collaboration and information sharing
Assessing pesticides and pharmaceuticals for EDC potential
Investigating New Approach Methods to reduce animal use in the assessment of EDCs
Introducing perspectives on mixtures assessment
Proposing methodologies and procedures for assessments under regulatory risk management of EDCs
3.3.2. A use-oriented approach: Labelling consumer products
Labelling schemes can be implemented to share information related to health or the environmental impact of products and packaging. Labelling can support consumers in making informed decisions on the products they use.
In 2022, the European Commission released a proposal for the creation of dedicated hazard classes for EDCs under the ‘EU Regulation on classification, labelling and packaging of substances and mixture’ (European Commission, 2022[47]). If adopted, this proposal requires manufacturers, importers or downstream users of substances or mixtures to classify, label and package their hazardous chemicals appropriately before placing them on the market. Besides “hazard labels” that warn consumers against potential hazards, positive labels can inform consumers on the low risk of using a product. For example, a study in Korea found that consumers are willing to pay around $2/year for an “EDC-free” labelling policy (Kim, Lee and Yoo, 2018[48]) (see also Box 3.5 on the OECD study on the willingness to pay to avoid negative health effects due to chemical exposure).
The Nordic Swan Ecolabel is an example of voluntary product labelling. It has adopted a set of principles in awarding their Ecolabel. It restricts products that 1) have hazardous properties (hazard-based approach in principle), though under specific circumstances a small quantity of hazardous substance can be allowed (risk-based approach when necessary), 2) are identified as endocrine disruptor or potential endocrine disruptor, and 3) are to be avoided based on precautionary principle, particularly applied to groups of similar substances (Nordic Swan, n.d.[49]). The supermarket COOP Denmark completely phased out the use of EDCs from their products (Box 3.2).
There are some challenges with EDC-labelling. Only a few compounds have been identified as EDCs, while the list of suspected compounds is much longer, and unknown endocrine disrupting compounds may exist. Absence of a hazard label or presence of an “EDC-free” label could therefore lead to the incorrect assumption that a product is truly free of EDCs (Government of Belgium, 2022[50]).
Box 3.2. Voluntary action by a Danish supermarket: co-benefits for the environment through safe consumer products
COOP Denmark is one of Denmark’s largest retailers. In 2015 COOP Denmark voluntary started to remove several EDCs1 from products and packaging. As there was no regulatory requirement to do so, the supermarket collaborated with suppliers, authorities and scientists to voluntarily phase out the EDCs from products. The process took several years. One of the main challenges was to find a cost-neutral alternative to the harmful substances.
The decision was driven by a wish to supply safe products and protect its consumers. Commercial interests also played a role, as the company wished to protect its brand and maintain high customer loyalty. While this decision was driven by the motivation to protect consumers from exposure to EDCs through food consumption and product usage, COOP Denmark may also have created co-benefits for the environment. As a percentage of consumer products still end up in freshwater through incineration facilities, landfills or litter, a safe product design may reduce endocrine disrupting effects in water.
To inform consumers about product safety COOP Denmark also worked with green labels awarded by third parties, such as Nordic Swan.
Note1: Per- and Polyfluorinated Compounds (PFC/PFAS0) and Bisphenols (BPA, BPS, BPFT, and others)
Source: Presentation by COOP Denmark at the Conference "Chemicals: better protecting health and the environment", organised within the framework of the French Presidency of the Council of the European Union, May 2022
3.3.3. End-of-pipe measures: Wastewater reuse and sewage sludge recovery
Urban wastewater treatment
Urban wastewater is one of the sources of EDCs in the environment as conventional wastewater treatment plants (WWTPs) are not designed to fully remove contaminants of emerging concern. A study of the removal of micropollutants in wastewater treatment processes in the Baltic Sea region showed that PFAS and pharmaceuticals are not efficiently removed by conventional wastewater treatment practices (HELCOM, 2022[51]). The study found similar concentrations both in influents and in effluents. What is more, some compounds (such as PFOA and PFNA) occurred in higher concentrations in effluents than in influents. Metals were only moderately removed.
Advanced wastewater treatment processes, such as reverse osmosis, ozonation, activated carbon, membranes and advanced oxidation technologies, can achieve higher removal rates in comparison to conventional secondary treatment. However, a “one size fits all” treatment for EDCs does not exist and no single technology can remove all EDCs (Azizi et al., 2022[52]; HELCOM, 2022[51]). What is more, upgrading treatment processes is not always necessary, nor cost-effective nor sustainable. Some treatment methods have high carbon emissions. The production of raw materials used for treatment can also have an environmental footprint. For instance, activated carbon is an effective method in removing PFAS, but its production and regeneration comes from the burning of fossil fuels (NORMAN Network and Water Europe, 2019[53]).
Countries commonly prioritise stringent treatment standards for those discharges that pose a particular pressure to health or ecosystems. This is the practice in Switzerland (OECD, 2019[13]). The NORMAN Network suggests the following criteria to prioritise WWTPs that could benefit from additional treatment (NORMAN Network and Water Europe, 2019[53]):
Large WWTPs service areas, and/or
WWTPs with a high proportion of wastewater compared to the receiving water body (consider seasonal and climate change scenarios to anticipate flows with lower dilution potential), and/or
WWTP that influence drinking water resources, and/or
WWTPs that influence valuable ecosystems.
The removal effectiveness and cost efficiency of wastewater treatment options, and options to finance wastewater treatment plant upgrades, are discussed in detail in Section 3.5 of the OECD report on Pharmaceutical Residues in Freshwater (OECD, 2019[13]). This discussion is highly relevant in the context of EDCs.
Wastewater reuse and sewage sludge recovery
Driven by the impacts of climate change, previously water abundant countries are increasingly facing droughts. Consequently, countries are turning towards reusing wastewater for agriculture, horticulture, cooling, or aquifer recharge (Fairbrother et al., 2019[54]). What is more, in transition to the circular economy, sewage sludge is more and more recovered as a nutrient for agricultural practices. However, wastewater reuse and sewage sludge recovery may put additional pressure on water quality as both products contain contaminants of emerging concern and their transformation products, including EDCs (Domini et al., 2022[55]; Sichler et al., 2022[56]; Kumar et al., 2022[57]). Advanced treatment of sludge and wastewater, monitoring and setting appropriate water quality standards can reduce the risk of endocrine disruption.
Advanced treatment and monitoring can securely provide recycled water and can sufficiently remove EDCs. The Environmental Protection Authority (EPA) of Victoria, Australia, studied contaminants of emerging concern (CECs), including EDCs, in recycled water (OECD, 2022[58]) (see also Box 2.1, Chapter 2). The study was performed at thirty WWTPs. It detected 181 contaminants, including 15 EDCs. In general, wastewater treatment was able to reduce the EDC concentration. The best treatment was a combination of activated sludge processes with extended aeration, ultraviolet light disinfection, microfiltration, reverse osmosis, and chlorine. However, this treatment is expensive.
In some countries, sewage sludge generated during wastewater treatment is applied as fertiliser for agriculture. However, EDCs and other contaminants of emerging concern have been detected in sewage sludge. Sludge disposal can thus unintentionally lead to the spread of EDCs on land and in water. The Danish Environmental Protection Agency (EPA) identified thousands of substances in treated sewage sludge through non-targeted screening analysis (Danish EPA, 2022[59]). Perfluorooctane sulfonic acid (PFOS), a type of PFAS, were detected on all sites. Other compounds detected were mercury, cadmium, 1H-benzotriazole, 2,6-dichlorophenol, bisphenol S, methylparaben, terbutryn and prosulfocarb. The concentrations of substances differed per site. In a follow-up study, the Danish EPA analysed three new alternatives for sludge disposal that can recycle phosphorous and minimise the emission of green-house gases (mono-incineration, pyrolysis integrated with pre-drying, and hydrothermal liquefaction) (Danish EPA, 2023[60]). The study stresses high uncertainty regarding the transformation of PFAS compounds during treatment, which can lead to the further spread of PFAS compounds during use.
Monitoring the water quality impacts of recycled wastewater can inform on the risk of negative impacts on health and ecosystems. As mentioned above, the Danish EPA applied non-targeted and suspect screening to determine micropollutants, including EDCs, in sewage sludge. California (United States) emphasised routine monitoring to ensure the safety of recycled water. California’s State Water Board introduced a state-of-the-art monitoring programme including two bioassays as a water quality indicator. One bioassay monitors estrogenic effects of effluents (California State Water Board, 2018[61])(see also Box 2.5, Chapter 2).
Whilst it may be obvious to adopt recycled water quality standards based on human health protection goals, given public concerns about the safety of recycled water, more stringent criteria based on the protection of ecosystems may be the better choice. Water quality standards are more stringent for ecosystem protection than for human health, as aquatic organisms are more susceptible to chemicals than humans due to their size and permanent exposure in water. Chapter 2 provides guidance on developing water quality standards for different purposes.
3.4. Policies centred around the effects of EDCs
Policy recommendations
Endocrine disrupting effects may be observed while the culprit is not immediately evident. Intervention strategies and response plans that put effects rather than individual culprit chemicals at the centre, combine the following actions:
Intervention strategies or response plans can reduce the lag time between observed or suspected abnormal effects, such as in wild fish, and the mitigation of the causes. In many instances, the suspected effects and culprit chemicals need to be confirmed through additional analysis. Valuable response plans cover accepted methods for collecting evidence, temporary no-regret or low-cost mitigation options, interpretation of exceeded threshold values or trigger values, roles and responsibilities of authorities and (suspected) sources of emission, and a communication plan.
Endocrine disrupting chemicals touch many sectors. National strategies and action plans on EDCs can build bridges across sectors and send a policy signal of national priorities related to the issue. National strategies and action plans can act as a first step towards developing policy instruments and monitoring programmes. Most national action plans also contain a research agenda to reduce uncertainties and guide on the development of measures.
Some authorities are considering introducing water quality regulation based on bioassays. Supplementing substance-by-substance water quality criteria with effect-based environmental quality norms could better capture the effect of mixtures, non-regulated chemicals, and impacts of low concentrations of chemicals. With the current state of development of bioassays and other methods, as well as the identification of endocrine disrupting chemicals, many regulators are not yet comfortable introducing monitoring programmes and regulations. A transition phase helps to establish a knowledge base, derive threshold values for monitoring and regulation, and mature the bioassay market.
Policies and actions could specifically consider the impact of EDCs on vulnerable populations. The risk of exposure to endocrine disrupting chemicals can be higher to certain groups within a population, such as children, adolescents, pregnant women, and lactating women. Moreover, exposure levels can differ within societies and across countries. Culturally important species or cultural keystone species could also be threatened. Interventions may therefore consider a wide scope of factors affecting vulnerability, including biological susceptibility, socio-economic vulnerability, and cultural vulnerability to EDCs. Actions could include risk assessments for vulnerable groups and populations, information campaigns targeting specific groups, and monitoring of endangered species and cultural keystone species.
Life cycle interventions, as discussed in the previous section, may not be fully fitted to EDCs and may result into a regulatory mismatch for several reasons. First, the sources of EDCs in freshwater vary widely, ranging from consumer products, industry, pharmaceuticals, agriculture, hormones, etc. Moreover, chemicals can act in mixtures, possibly when the life cycles of compounds come together. Lastly, not all EDCs are identified, suspected or even known as EDCs, while effects may already be detected in effect-based monitoring programmes and non-target screening. With the increased use of bioassays as water quality monitoring method, that do not identify the culprit chemical at once, effect-centred approaches can complement life cycle-based interventions.
Effect-centred approaches impose, incentivise or encourage measures that reduce the cumulative impacts of endocrine disruptors on humans, aquatic species or ecosystems, regardless of a compound’s regulatory identification as endocrine disruptive or endocrine active. Effect-centred approaches typically respond to the results detected in water monitoring, such as bioassays, emphasize precautionary measures directed at vulnerable populations, and adopt an intersectoral approach to reduce environmental pressures from EDCs. As with effect-based monitoring, effect-centred policy approaches take the effects of endocrine disruption on human and ecosystem health as a starting point for action.
3.4.1. Effect-based environmental quality norms
It remains to be seen if the current system of environmental quality norms (EQNs; also known as environmental quality standards) is a viable option to address contaminants of emerging concern. Existing EQNs aim to determine acceptable water quality based on an assessment of individual compounds. Some of the challenges are the vast number of chemicals in the environment, the effect of mixtures, and the time it takes to develop quality norms for every single chemical (OECD, 2019[13]). Combining compound and effect-based norms may thus provide a more holistic picture of water quality (Brack et al., 2018[62]).
There are few cases of effect-based methods (bioassays) as an environmental quality norm. The California State Water Board is the first regulator to adopt reporting limits for the estrogen receptor (ERa) and the Aryl hydrocarbon receptor (AhR) for recycled wastewater, the former being associated with endocrine activity or disruption (California State Water Board, 2018[61]). The European Commission has submitted a proposal that recommends the monitoring of estrogenic activity in water bodies, and that gives way for the adoption of effect-based environmental quality norms in the future (European Commission, 2022[63]).
Environmental quality norms based on bioassays are ideally generic and based on non-animal methods (see Table 2.3, Chapter 2). Generic environmental quality norms (i.e., not pegged to a specific brand of bioassay) are more appropriate in the regulatory context because they do not discriminate between methods and they allow any bioassay-provider to enter the market. As animal methods are still used in effluent testing, regulators should avoid prescribing a regulatory standard that unintentionally stimulates animal testing, particularly if non-animal methods are available. Section 2.6, Chapter 2, explains in more detail how to derive environmental quality norms for bioassays. A transition phase can support the implementation of effect-based environmental quality norms. Other requisites that need to be in place before adopting an effect-based environmental quality norm are a monitoring budget, laboratory capacity, sampling protocols and a sufficient supply of bioassays.
Adopting a transition phase to avoid decision paralysis
With the current state of development of bioassays, many regulators are not yet comfortable in adopting effect-based methods as an environmental quality standard. A transition phase to establish a knowledge base, derive effect-based trigger values or environmental quality norms, and develop a mature market for bioassays can be instrumental in overcoming some barriers. It gives room for trial and error before an official regulatory standard is adopted.
Concentrating efforts on estrogenic bioassays can be an appropriate first step as estrogenic assays have been validated and are widely accepted, and knowledge of these effects is relatively well established (OECD, 2022[58]). Adverse outcome pathways and bioassays for other endocrine axes, EATS and non-EATS, are less well established. For example, there are no standardised in vitro bioassays for the thyroid axis even though thyroid disruption is known for disrupting metamorphosis in amphibians (OECD, 2022[58]).
The European Commission's proposal for amending the Water Framework Directive suggests an intermediary phase of applying chemical analysis alongside effect-based monitoring of estrogens for a period of at least two years (European Commission, 2022[63]). This two-year period allows time to collect and compare data which can inform any future decision on the use of routine effect-based monitoring and deriving effect-based trigger values. Chemical analysis will monitor the individual compounds of E2, E1 and EE2 (comparing concentrations against threshold levels), while effect-based methods will monitor estrogenic effects.
The California State Water Board also adopted an intermediate phase for optimising the selection of effect-based methods (Box 2.5, Chapter 2). The Scientific Advisory Panel of the California State Water Board recommended a three-phased approach towards the adoption of bioassays (Drewes et al., 2018[64]):
Phase 1: Data collection to determine the range of responses for in vitro bioassays and to confirm that the in vitro bioassays represent endpoints relevant to human health.
Phase 2: Pilot evaluation of the effect-based trigger values, i.e., of the interpretation of the monitoring results by in vitro bioassays. This includes interlaboratory comparisons.
Phase 3: Full implementation of bioassays as an integral component of routine screening/monitoring of recycled water quality.
The California State Water Board implemented bioassays (ERa and the Aryl hydrocarbon receptor, AhR) as a pre-screening tool of water quality hotspots affected by wastewater recycling (California State Water Board, 2018[61]). The selected bioassays will be tested for a period 3 years starting in 2020, after which an evaluation will take place on the relevance of the methods, and whether it is appropriate to continue, remove or substitute the current bioassays. During this period no regulatory action will be undertaken if the threshold values are exceeded (OECD, 2022[58]).
Canada’s Environmental Effects Monitoring (EEM) Programme (Chapter 2, Box 2.8) also allowed ample time for setting the right trigger values for in situ wildlife monitoring (Environment Canada, 2010[65]). The trigger values were not set until the results of four monitoring cycles were collected for two fish species at 125 pulp & paper mill sites, which took 12 years. Moreover, the EEM Programme has built in regulatory feedback loops. It cyclically evaluates if effluent standards are adequate in protecting fish, fish habitat and fish usability. Guidance documents are updated if needed. This demonstrates the need for patience to acquire data to set threshold levels and the importance of flexibility to respond to new findings and arising needs.
3.4.2. Response plans
Intensified monitoring of endocrine disruptors and endocrine disrupting effects will likely increase the need to intervene and implement mitigation actions. Case studies often show a lag time between observed abnormalities and mitigating action. See for example the case of France where, three years after fishermen observed changes in fish, actions were taken to eliminate the pollutant (Box 2.9, Chapter 2) (Creusot et al., 2014[66]; Sanchez et al., 2011[67]). Similarly, the Canadian EEM programme works in three-year cycles, and it can take up to six years between observation of abnormalities and mitigation actions (Box 2.7, Chapter 2) (Environment Canada, 2010[65]).
This lag time is often caused by a need to validate effects through further research, notably by confirming effects through effect-directed analysis or in vivo methods. Collecting proof of the causality between the culprit chemical and observed effect is also time-consuming. All the same, delaying mitigation action comes with risks to human health and ecosystem integrity, as well as economic costs. The cost of inaction, or acting after the damage is done, is likely more expensive than preventive measures. On the other hand, unnecessary action is also costly, such as disrupting business processes, sediment remediation or upgrading wastewater treatment plants.
There is value in developing a response plan, protocol or good practice guide or other approach to quickly mobilise a response to observed or suspected abnormal effects in freshwater. A response plan could cover, for example:
Accepted methods for collecting evidence. This includes describing how a weight of evidence shall be established. It ensures that regulators follow a consistent, clear, and transparent delivery of evidence for the evaluation of water contamination. Moreover, it determines the circumstances under which in vitro methods, QSAR, grouping and/or international databases are accepted as evidence. This is particularly important as the international definition of endocrine disruption still leads to the expectation that “consequently causes adverse health effects in an intact organism, or its progeny, or (sub)populations” (WHO-UNEP, 2013[30]). A protocol that describes accepted methods can help to avoid unnecessary animal testing.
Temporary no-regret or low-cost mitigation options, such as putting in place buffer zones, temporarily taking suspected effluents to tertiary treatment facilities, providing warnings to consumers to hold off consumption of suspected fish or crops.
Guidance on the interpretation of exceeded threshold values or trigger values, and the appropriate response actions. For example, the California State Water Board works with different tiers of action depending on the level exceedance of the bioanalytical equivalent concentration (BEQ) (Table 3.3) (California State Water Board, 2018[61]).
Roles and responsibilities of involved authorities (Ministry of Health, EPA, utilities, basin authority), industry, and the actor responsible for the emission. The EEM programme in Canada is an example of a monitoring programme where the role of each stakeholder is well defined (Box 2.7, Chapter 2).
A communication plan that details out how the monitoring results can be interpreted and explained, particularly in relation to health concerns for human and wildlife, and that explains any follow-up actions. The communication plan may also make general recommendations on behavioural changes and making environmentally friendly choices.
Table 3.3. California’s State Water Board’s response actions to detected activity in bioassays
BEQ/MTL Threshold |
Response action by the recycled water producer |
---|---|
If BEQ/MTL ratio is consistently less than or equal to 0.15 for ER-α or 1.0 for AhR |
After completion of the pilot monitoring phase, consider decreasing monitoring frequency or requesting removal of the endpoint from the monitoring programme. |
If BEQ/MTL ratio is greater than 0.15 and less than or equal to 10 for ER-α or greater than 1.0 and less than or equal to 10 for AhR |
Continue to monitor. |
If BEQ/MTL ratio is greater than 10 and less than or equal to 1000 |
Check the data, resample within 72 hours of notification of the result and analyse to confirm bioassay result. Continue to monitor. Contact the regional water board and the State Water Board to discuss additional actions, which may include, but are not limited to, targeted analytical chemistry monitoring, increased frequency of bioassay monitoring, and implementation of a source identification program. |
If BEQ/MTL ratio is greater than 1000 |
Check the data, resample within 72 hours of notification of the result and analyse to confirm bioassay result. Continue to monitor. Contact the regional water board and the State Water Board to discuss additional actions, which may include, but are not limited to, targeted and/or nontargeted analytical chemistry monitoring, increased frequency of bioassay monitoring, toxicological studies, engineering removal studies, modification of facility operation, implementation of a source identification program, and monitoring at additional locations. |
Note: BEQ = Bioanalytical Equivalent Concentration; MTL = Monitoring Trigger Level (nanograms/litre)
Several OECD documents could serve as a model for guidance documents targeting EDCs, such as the Principles on Good Laboratory Practice (OECD, 1998[68]), Best Available Techniques to Prevent and Control Mercury Releases to Land and Water (OECD, 2022[69]), and Guiding Principles and Key Elements for Establishing a Weight of Evidence for Chemical Assessment (OECD, 2019[70]).
Regulatory entry-points can justify and accelerate precautionary action, such as legislative clauses permitting action based on the precautionary principle or acknowledging EDCs as a potential risk. The European Drinking Water Directive, for example, refers to endocrine-disrupting compounds at several places of the preamble – providing an entry point for action (European Union, 2020[71]).
3.4.3. National action plans on EDCs
As EDCs touch many sectors, there is a need for a cross-sectoral approach. National action plans or strategies can act as a first step towards developing policy instruments and monitoring programmes. National action plans can coordinate efforts between sectors, strengthen knowledge, and, more generally, send a policy signal on the priorities of government. National action plans are common practice in the One Health context, such as the National Action Plans on Antimicrobial Resistance (AMR) (OECD, 2019[13]; Özçelik et al., 2022[72]; Anderson et al., 2019[73]; Brack et al., 2022[74]).
National action plans are increasingly adopted for endocrine disruption. France is one of the frontrunners with its Second National Strategy on Endocrine Disruptors (Box 3.3). Other examples are Japan’s EXTEND programme (Box 3.1) and Belgium’s National Action Plan on EDCs 2022-2026 (Section 3.4.2) (Government of Belgium, 2022[50]). In Canada, researchers from multiple disciplines have grouped themselves in the Intersectoral Centre for Endocrine Disruptors Analysis (ICEDA) (Box 3.4).
Based on existing national strategies on endocrine disruption, and the national action plans on antimicrobial resistance (WHO, n.d.[75]; Özçelik et al., 2022[76]), the following checklist for water-relevant national strategies on endocrine disruption emerges:
A description of the coordination, governance structure and implementation across sectors, at least involving the human health sector, chemicals’ sector, agricultural sector, environmental sector (including water and biodiversity), food safety (including drinking water, packaging, and agricultural and aquaculture products), and industry.
An analysis of regulatory strengths and weaknesses, identifying regulatory gaps and science-policy gaps. Such an assessment is part of Belgium’s National Action Plan (Government of Belgium, 2022[50]). The European Commission published a regulatory Fitness Check on EDCs in 2019-2020, assessing whether the different pieces of EU legislation are fit to address the human health and ecosystem impacts of EDCs (European Commission, 2020[77]). The fitness check recognised that the EU regulatory system of EDCs is overall fragmented and limited, and it urges a comprehensive simplification and consolidation.
Research priorities and exploratory work to fill gaps, particularly on the assessment of chemicals and their impact on humans and ecosystems. Research and chemicals’ assessments are an integral part of the national strategies of Belgium and France (Government of Belgium, 2022[50]; Ministère de la transition écologique et solidaire, 2019[78]) (Box 3.8).
A set of actions targeted at the reduction of EDCs in the (aquatic) environment, ranging from no-regret measures, precautionary or hazard-based approaches for critical hotspots. Actions or investments that require additional cost-benefit analyses, such as more stringent wastewater discharge standards can also be prepared or implemented.
Water quality monitoring, including scaling up existing programmes and developing new initiatives. This includes pilot projects or roll-out of new methods such as bioassays and non-targeted screening. Pilot projects can create “snapshots”, “archives” or “digital freezes” of water samples, which can be useful for future analysis (Badry et al., 2022[79]).
Communication activities that improve awareness of the risks of EDCs and that guide on risk-reducing actions. Communicating the results of new monitoring methods (such as bioassays) to decision-makers, industry and the public demands specific attention, as this is highly technical and prone to misinterpretation. Communication activities could also address public water quality concerns, such as concerns related to chemicals found in the environment and drinking water. Belgium’s national strategy prioritises communication and outreach activities specifically targeting vulnerable populations (Government of Belgium, 2022[50]). People’s willingness to pay across OECD countries to address chemicals-related health risks, including fertility loss and low birthweight, underlines the relevance of action plans and public communication (Box 3.5).
A costed implementation plan with an indication of funding gaps, a resource mobilisation strategy, and a monitoring and evaluation plan.
The global scope of the issue lays bare how tackling EDCs from only a national level is not nearly enough. As such, a coherent, coordinated and far-reaching global strategy needs to be developed, in parallel with national policy frameworks. Developing a global approach to the issue of EDCs could be inspired by the Global Action Plan on Microbial Resistance (WHO, 2015[80]).
Box 3.3. France’s Second National Strategy on Endocrine Disruptors
In 2019, France launched its Second National Strategy on Endocrine Disruptors (SNPE 2) to tackle EDCs in all spheres of society, including freshwater (Ministère de la transition écologique et solidaire, 2019[78]). It is part of the fourth national plan health environment called “My environment, My health” of the Ministry of Ecological Transition and the Ministry of Solidarity and Health. Both programmes aim to protect biodiversity, health and ecosystem integrity through prevention and pollution reduction. A French survey published in 2018 and 2022 by the Institute of radioprotection and nuclear safety (IRSN) indicated that half of the population was concerned about (IRSN, 2018[81]; IRSN, 2022[82]). The SNPE 2 encompasses a total of 50 actions to tackle EDCs, which are classified in three main goals:
1. Training and informing. This goal aims to prevent exposure by training healthcare professionals, workers manipulating EDCs (e.g., farmers), and workers in contact with vulnerable populations (e.g., teachers). One of the objectives is to inform parents about creating a better environment for their new-borns (1000-premiers-jours.fr, n.d.[83]). The programme also published a list of confirmed and suspected EDCs (edlists.org, n.d.[35]). Chemical assessments are also part of this goal.
2. Protecting the environment and the population. This goal is based on the “One Health” concept, which presupposes that human health is linked to the health of wildlife and the environment. This goal aims to continue and improve the monitoring of EDCs found in water, air and soil. It makes the data publicly available. Furthermore, this goal explores the best available techniques to address contaminated sites. Moreover, this goal funds research projects and ignites voluntary action to reduce the number of products containing EDCs and substitute EDCs with safer chemicals. Lastly, this goal reiterates France’s ambition to tackle the issue at EU-level.
3. Improving knowledge. This goal aims to reinforce the evaluation of substances. It supports the development of tools and methods to assess EDCs and it finances public-private platforms to conduct method validation (see, for example, Box 3.8). Furthermore, research on EDCs, their mode of action and their impact on human (studies of cohorts) and ecosystem health will be continued to improve knowledge, model EDC exposure (i.e. their fate in the body and environment), and develop good laboratory practices.
SNPE 2 aims to be as inclusive as possible. The strategy considers multiple stakeholders, including politicians, experts, industry, associations for the protection of the environment and consumers, the public and more. It specifically considers vulnerable populations (babies, teenagers and pregnant women) and populations with social vulnerability (exposed workers, specific social context, geographical location).
Box 3.4. Scientists join forces across disciplines and sectors to better manage EDCs in Canada
To remove barriers and decompartmentalise knowledge between and across sectors of industry and academic disciplines, researchers in the province of Quebec, Canada, founded the Intersectoral Centre for Endocrine Disruptors Analysis (ICEDA) in 2020. ICEDA’s mission is to inform, assist and provide resources to the government, non-governmental organisations, industry and the general population in the identification, recognition, quantification, and management of EDCs. To achieve this mission, ICEDA’s work is divided into three axes: intersectoral collaboration, knowledge sharing and the active involvement of policymakers.
To stimulate intersectoral collaboration and update the current knowledge on EDCs, ICEDA published an open-access special issue on EDCs in the Environmental Research journal (Langlois et al., 2022[84]). The edition comprises fourteen peer-reviewed articles with topics ranging from EDC detection methods, endocrine endpoints and regulation and remediation of EDCs. The lead authors presented the highlights to federal ministerial departments on health and environment.
To raise awareness of EDCs, ICEDA also invests resources in public outreach, especially to children. ICEDA reaches out to families through children's books, teen magazines, video clips and interactive activities. Moreover, young researchers are involved in every project.
ICEDA also stimulates dialogue between academia and all levels of government (federal, provincial, and municipal) to improve policies that address EDCs. Policymakers take part in ICEDA committees to guide on the development of the scientific program. Moreover, ICEDA launched a series of workshops to discuss ways to move from science to action for EDCs in Canada. Some recommendations from the workshops were: governing bodies (including regulators), academia and NGOs, should continue collaborating on chemical related regulation and management; stronger direct links between lab-based research, NGOs and policymakers should be made; and science should be translated in understandable language for public consumption.
ICEDA has 170 members, including 71 students, from 48 institutions and 8 different countries. It received funding from the Institut national de la recherche scientifique and the Fonds de recherche du Québec Nature et technologies (FRQNT).
Source: Case study provided by Myriam Castonguay, Coordinator of ICEDA. The Special issue on EDCs: (Langlois et al., 2022[84])
Box 3.5. Willingness-to-pay to avoid negative health effects due to exposure to chemicals
The OECD project on “Surveys of willingness-to-pay to avoid chemicals-related health effects” (SWACHE) indicated that people in OECD countries are willing to pay a significant amount to reduce chemicals-related health risk. Several health outcomes included in the SWACHE project can be linked to exposure to EDCs such as fertility loss, very low birth weight, and IQ loss. On average, people are willing to pay USD 3 0501 to avoid the loss of 1 IQ point in their children; future parents are willing to pay USD 91 0002 to avoid infertility; and parents are willing to pay USD 1 194 0003 to avoid very low birth weight of their new-born (Dussaux et al., 2023[85]; Mourato et al., 2023[86]; Ščasný, Zvěřinová and Dussaux, 2023[87]).
The results of this study could support decisions on whether chemicals management options and environmental policies are worth implementing based on a cost-benefit analysis. Assessment of chemicals management options and environmental policies can be considerably improved by better estimating their costs and benefits. The SWACHE project provides data to support such analyses. Understanding people’s willingness to pay to avoid negative health outcomes supports the quantification of the benefits of chemicals regulation and the cost of policy inaction.
The values estimated in these studies could feed into cost-benefit analyses of water management decisions, such as investments in additional wastewater treatment infrastructure or the remediation of sediments when such investments benefit human health4.
It should be noted that the results are not limited to exposure to EDCs through water. The studies take into account any class of chemical and any type of medium. Moreover, this study only evaluated health endpoints. Environmental endpoints, such as biodiversity loss, are not covered generating willingness to pay value that can be used for the evaluation of a variety of environmental and health policies and not only chemicals management options.
Note1: USD2022 Purchasing Power Parity (PPP) 3 050
Note2: Value of a statistical case; USD2022 PPP 91 000
Note3: Value of a statistical case; USD2022 PPP 1 194 000
Note4: While the survey questionnaires employ safer chemicals products as a payment vehicle to elicit willingness to pay, the surveys successfully deliver people trade-off between a reduced risk and a higher private cost.
3.4.4. Minimising negative impacts on vulnerable populations
Some populations are particularly vulnerable to EDCs, which can take various forms: biological susceptibility, socio-economic vulnerability, and cultural vulnerability to EDCs. The risk of exposure to EDCs can be higher to certain groups within a population, such as children, adolescents, pregnant women, and lactating women. Moreover, exposure levels can differ within societies and across countries, such as communities living close to contaminated sites, or groups that eat relatively more contaminated fish - for example, due to their socioeconomic status (U.S. EPA, 2019[88]). Physical effects aside, humans can also be culturally affected by EDCs when the existence of culturally important species or cultural keystone species is threatened. This is especially relevant to indigenous peoples.
Table 3.4. Factors affecting susceptibility to chemicals or pollutants
Intrinsic factors (biological) |
Extrinsic factors (exposure-related) |
Extrinsic factors (biodiversity-related) |
---|---|---|
Age and life stage Gender Race/ethnicity Genetic polymorphisms |
Disease status Socio-economic status Nutrition status Geographic proximity Lifestyle |
Cultural practices and needs |
Source: Adapted from USEPA “Factors affecting susceptibility” (U.S. EPA, n.d.[89])
To improve human exposure risk assessments, the U.S. EPA has integrated a specific guidance and toolbox of techniques to assess risks for vulnerable groups and populations (U.S. EPA, 2019[88]). The multitude of sources and exposure routes, some of them uncertain, complicates the design of policies targeting vulnerable populations. Humans can be exposed to chemicals through different routes and water is only one source of exposure (Govarts et al., 2023[90]). Food products, consumer products, air, or occupational activity also contribute. The relative contribution of each of these sources to the health of a human being is hard to establish and varies from compound to compound. Risk assessments for vulnerable groups and populations can guide the development of policies.
Some policy options to targeting biologically susceptible groups have been put to practice. Through dietary advice campaigns, Sweden discourages children, adolescents and women of childbearing age from consuming contaminated fish and fish from specific water bodies, such as the Baltic Sea and several lakes, due to the concentrations of dioxins and PCB in fish (Swedish National Food Administration, 2008[91]). The Belgian National Action Plan on Endocrine Disruptors endorses several actions to protect vulnerable groups from exposure to pesticides. Examples are information campaigns on limiting the use of plant protection products around schools, playgrounds, childcare facilities and health care facilities, and subsidies for initiatives that inform, guide or raise awareness of vulnerable groups (Government of Belgium, 2022[50]; Government of Belgium, 2018[92]).
Looking at social vulnerabilities, EDCs may constitute an environmental justice issue, although the patterns are complex. The US EPA’s definition of environmental justice is: “Environmental justice is the fair treatment and meaningful involvement of all people regardless of race, colour, national origin, or income with respect to the development, implementation and enforcement of environmental laws, regulations and policies” (U.S. EPA, n.d.[93]). Socio-economic status can influence exposure to EDCs. There is evidence that marginalised communities - with lower socio-economic status - have reported higher exposures to EDCs (Ruiz et al., 2017[94]). Inequalities in EDCs exposure have been observed in the United States (Attina et al., 2019[95]; Pumarega et al., 2016[96]). Ethnic minorities are disproportionately exposed to these chemicals, hence contributing to inequalities in diseases and disability. However, the role of water pollution hotspots in creating such environmental injustices is less studied. Some compounds have been found in higher concentrations in populations with higher socio-economic status (Govarts et al., 2023[90]). Studies in Belgium and the United States found an association between fish and shellfish consumption, high socio-economic status and relatively high concentrations of chlorinated compounds (in Belgium) and PFOA, mercury and arsenic (in the United States) in the sampled populations (Morrens et al., 2012[97]; Schoeters et al., 2022[98]; Tyrrell et al., 2013[99]).
Policies should also consider the needs and practices of indigenous peoples, in particular in protecting culturally significant species and cultural keystone species on which indigenous communities depend for their social, economic, physical and spiritual wellbeing (Garibaldi and Turner, 2004[100]). Some aquatic species are of cultural significance to indigenous communities (Noble et al., 2016[101]). EDCs could threaten the abundance, size, or distribution of species, potentially including those species that are of cultural significance. In New Zealand, Māori communities and scientists conducted a 4-year research project on aquatic cultural keystone species. The project developed cultural values-based environmental assessment and reporting frameworks, and co-management and restoration strategies (NIWA, n.d.[102]). Whilst this project was not specifically targeting the issue of EDCs, its design could be relevant in identifying pressures from EDCs on cultural keystone species. Canada is integrating environmental DNA (eDNA) tools and indigenous ecological knowledge to model regional biodiversity changes (Box 2.8, Chapter 2).
3.5. A global challenge: international actions at the forefront
Policy recommendations
A global issue of concern, endocrine disruption is appropriately addressed at an international level. International actions can also boost innovation in water monitoring and assessment. Many regulators and utilities, in OECD and non-OECD countries alike, face challenges in the roll-out of water quality monitoring based on bioassays. Access to internationally standardised bioassays relevant for water quality testing, limited bioanalytical laboratory capacity and costs are common barriers. The following repertoire can inspire global responses to endocrine disruption:
There is need to upscale, at international level, the standardisation and verification of test methods that are appropriate for water quality testing, based on international environmental technology verification processes or the principle of mutual acceptance of data. Currently, there are only few international guidelines and standardised methods for sampling and analysis for water quality testing using bioassays. The International Organization for Standardization (ISO) provides relevant methods for water quality testing and testing the estrogenic potential of water and wastewater. OECD Test Guidelines for EDCs are also relevant, though these have not been specifically developed for the purpose of water quality testing.
The international market of bioassays for water quality testing needs to be expanded in terms of number of suppliers, endpoint variety and geographical service areas. Stimulating the demand for and development of new bioassays, based on non-animal methods, can support in achieving a diverse supply and reasonable cost of methods. Performance standards for bioassays, ideally at the international level to create a level playing field, can ease the market entry of new methods and method providers.
Governments, at national level, could stimulate the uptake of new methods, by developing user toolkits for water authorities or water utilities and by training commercial, governmental and/or medical laboratories to perform bioanalytical methods for water quality testing. Laboratories specialised in water quality analysis often lack in-house expertise to apply bioanalytical methods, and training programmes for laboratory experts have proven to be effective.
Mainstream the issue of endocrine disruption on global science-policy agendas, such as agendas on chemicals management, waste management and the One Health agenda.
International research partnerships can encourage knowledge- and data-sharing on EDCs. Research partnerships have also been instrumental in the transition to new monitoring methods and the development of regulation.
There is a strong rationale for international and regional coordination to address endocrine disruption, as EDCs 1) are transported across international basins and ecosystems, 2) may be imported into a jurisdiction (national or sub-national) through trade, and 3) create impacts that are experienced globally (Godfray et al., 2019[103]; Kassotis et al., 2020[31]). The Endocrine Society recognises EDCs as a global health issue and affirms that “health issues related to EDCs cannot be geographically compartmentalised and should be addressed by intergovernmental actions” (Endocrine Society, 2018[104]). Yet, many research and policy initiatives, such as biomonitoring and water quality monitoring programmes, are limited to high-income countries. Low- and middle-income countries cannot afford such programmes and yet often are disproportionately exposed to products and waste (Kassotis et al., 2020[31]). This section presents four recommendations for actions at the international level.
3.5.1. International market for bioassays
The test method available on the market - bioassays in particular - do not meet the needs of regulators and water service providers across the world. The market of bioassays for the purpose of water quality testing is limited in terms of number of suppliers, variety of endpoints, standardisation of tests (more on this in the paragraph below), suppliers’ and laboratories’ awareness of the specificities of water quality testing, and awareness of regulators and water service providers on the advantages and disadvantages of bioassays. International collaboration can accelerate the development of robust knowledge and databases, stimulate markets for new (monitoring) technologies, and support standardisation at the appropriate geographical scale. Moreover, demand-driven initiatives can facilitate market access to small and medium-sized enterprises and reduce costs at a longer term. Box 3.6 describes the international market of bioassays and makes recommendations for improvement. Methods such as mass spectrometry can also be scaled up at international level, for example by training national experts and by sharing robots across regions.
Box 3.6. The demand and supply of bioassays: a blind spot for water quality testing?
One of the main barriers to adopting bioassays for water quality testing is their limited availability, even though the assays may exist for other purposes such as pharmaceutical development, chemicals assessment and food analysis. This box characterises the international market of bioassays for water quality testing.
Supply-side
The global market of bioassays for water quality testing is dominated by a small number of international companies. Bioanalytical companies provide cell lines, test kits, and licences to laboratories to use their methods. Currently, only one company provides services across the full bioanalytical chain - from sample treatment to analysis - removing the need to run bioanalytical tests by the regulator or specialised laboratories.
In addition, academia is involved in developing bioassays, but these are often not offered on the market or made available for public use. Similarly, companies outside of the environmental sector, such as pharmaceutics, are involved in the development of bioassays that are not adapted to water quality monitoring.
To facilitate the uptake of bioassays for water quality monitoring, the supply market needs to mature. The international market of bioassays specific to water quality testing needs to be expanded in terms of suppliers, bioassay variety and geographical service areas. Market diversification can, first of all, make substitutes available which makes the market less sensitive to disruptions in supply, and secondly, reduce the costs of cell lines and kits. Governments could play a role in stimulating supply by accelerating the validation of bioassays (see for example Box 3.8), standardising methods (Box 3.7), developing performance standards for new methods, transferring technologies from other sectors to the water sector, and overall by stimulating demand.
Demand-side
With increasing interest to monitor endocrine disruption and other chemical risks in water, there is an expectation that the market for bioassays for water quality monitoring will expand. Potential demand comes from wastewater treatment facilities for municipal water and recycled water, the drinking water surveillance chain (from source water to treatment), and authorities tasked with environmental monitoring. Middle-income countries form a potential market once the supply side matures and the price decreases. For these countries, bioassays could possibly become a partial substitute for expensive chemical analysis, such as for the monitoring of pharmaceuticals.
In many countries, laboratories do not have the capacity to perform biological analysis at scale. Water quality and accredited commercial laboratories often lack bioanalytical capacity, whereas medical laboratories lack knowledge of water samples. As a consequence, bioanalytical analysis is outsourced to university laboratories which cannot deliver at scale and may not have the personnel specialised in water safety analysis.
Governments could stimulate demand by training commercial, governmental and/or medical laboratories to perform bioanalytical methods for water quality testing. User toolkits for water authorities or water utilities could also stimulate demand, such as the “Deltafact” toolkit in the Netherlands. The Deltafact toolkit includes simple explainers of bioassays, a cost assessment, suggested batteries of bioassays for drinking water, surface water and wastewater, case studies and knowledge gaps (De Baat, Van Den Berg and Pronk, 2022[105]).
Source: Authors, based on correspondence and interviews with stakeholders from the water sector.
3.5.2. International standardisation and validation of test methods for water quality testing
One of the main barriers to adopting new monitoring tools, such as bioassays and non-targeted analysis, is the lack of standardisation and validation of methods. Standardisation and harmonisation at international level, based international environmental technology verification processes or on the mutual acceptance of data principle, can avoid duplication, and therefore reduce costs. For instance, the net benefits of the OECD work on Environmental Health and Safety, including the OECD Mutual Acceptance of Data (MAD) system for chemicals testing and assessment, are estimated to be more than EUR 309 million per year (OECD, 2019[106]).
Currently, only few international guidelines on how to use and analyse bioassays and prepare samples for water quality testing have been developed. ISO has standardised methods for water quality testing and testing the estrogenic potential of (waste)water (e.g., the ISO 5667 series on water sampling and the ISO 19040 series on the estrogenic potential of water and wastewater). Moreover, the OECD Test Guidelines for bioassays are a useful tool for analyses, but these need to be further tailored for purposes of water quality testing. This means that authorities interested in the implementation of such methodologies need to rely on highly trained experts to develop monitoring strategies and methods. International collaboration to develop those guidelines and standardised protocols would make new approaches clearer and more accessible. Those guidelines would need to cover topics such as sampling, sample preparation, bioassays, analysis of results and risk assessment. The Global Water Research Coalition is an international initiative that has made steps towards standardisation of effect-based methods and sampling for water. Box 3.7 presents recommendations on the standardisation of methods. Box 3.8 presents an initiative that supports bioassay developers in the validation of their test methods in an OECD Test Guideline.
Box 3.7. Standardisation of methods for water quality testing
One of the ways to enhance water quality monitoring this is to standardise and verify methods for the specific purpose of water quality testing (e.g., through setting performance standards, environmental technology verification processes, good practices, or guidance documents on adapting test guidelines for water sampling). The four methods below can particularly benefit from standardisation:
Bioassays
Accelerating the standardisation of bioassays for water quality testing is crucial for the maturation of water quality monitoring. Aspects of the standardisation process are the verification of methods, the development of performance standards for new methods, and the development of a broad range of methods that address different endpoints relevant to water quality. Initiatives that support in the validation of test guidelines for ISO or OECD, such as the Plateforme public-privé pour la pré-validation des méthodes de caractérisation des perturbateurs endocriniens (Pepper) (Box 3.8), can facilitate this process. Standardisation of bioanalytical methods include a description of the method, procedures, observation, data and reporting, validity criteria of the test, apparatus and materials, test documentation and report templates, and a standard operating protocol (SOP) for the test performance.
Sampling
Sampling strategies and sample preparations are key to the successful performance of bioanalytical tests, and other monitoring strategies. The development of guidelines and standard operating procedures would facilitate the use of effect-based methods, effect-directed analysis and non-target screening (Neale et al., 2022[107]). To date, sampling is standardised in ISO standards (e.g. ISO 5667 on design of sampling programmes and sampling techniques for all aspects of sampling of water, and ISO 19458 on sampling for microbiological investigations) (WFD, 2009[108]), but it has not been mainstreamed across methods. California and Switzerland have developed a Standard Operating Procedure for sampling for water quality testing by bioassays (Kienle et al., 2015[109]; NWRI, 2020[110]). Generic guidelines on testing water samples can support the standardisation of tests. It could be worthwhile exploring if existing and future OECD Test Guidelines can be complemented with a sampling protocol for those methods that are relevant to water quality testing. Lastly, passive sampling is an upcoming method that is not standardised.
Databases
To widely adopt non-targeted analysis (NTA) and effect-directed analysis (EDA), there is a need for standardisation and harmonisation of methods to ensure that data can be exchanged and compared, for example regarding potential culprit chemicals detected with EDA methods. Keeping global records of NTA data also ease effect-directed analysis, as databases of suspected effect-drivers are readily available. Databases of collected data for chemistry (e.g. Information platform or chemical monitoring (IPCHEM)) can help information sharing, which in turn could help regulation (Hollender et al., 2019[111]).
eDNA/eRNA methods
To adopt eDNA methods for ecological surveys, invasive species management, and regulatory purposes, methods of standardisation are needed. An example of eDNA standardisation is Canada’s iTrackDNA programme (for more on this programme, see Box 2.8, Chapter 2).
Source: Authors
Box 3.8. Pepper: a public-private partnership to accelerate the validation of bioassays
The Pepper Platform (Plateforme public-privé pour la pré-validation des méthodes de caractérisation des perturbateurs endocriniens) is a public-private platform, based in France, that supports bioassay developers in the process of pre-validation of test methods for the identification of endocrine disruptors.
The validation of test methods, such as bioassays, ensures the quality of reproducible methods that can be used around the world and in which regulators can have confidence. Validation can result, for example, in an ISO standard or an OECD Test Guideline. While a careful validation process is necessary, the validation process is also long and costly. The validation process of an in vitro bioassay takes at least two years and can cost as much as EUR 1 million or more, excluding the costs of method development. To give an example of the costs involved for the validation of in vitro bioassays in an OECD Test Guideline, requires that three laboratories without prior experience with the method acquire the know-how to apply the method, demonstrate the repeatability, predictability and reproducibility of the results for 30 chemicals, replicating the experiments at least three times. For in vivo bioassays, this process is even longer and more expensive.
Pepper selects methods that are mature and that meet the FRAND (Fair, Reasonable, and Non-Discriminatory) guiding principles of the OECD Test Guidelines. This means that the method needs to be accessible for users around the world, at a reasonable price. The Scientific Committee safeguards the quality.
Pepper supports the method developer in writing a standard operating protocol (SOP). Pepper also selects two other laboratories to test the SOP. The SOP can then be adjusted, if necessary, before starting the long work of establishing the reproducibility and predictability of the method. Pepper is also involved throughout the standardisation process with the OECD, from the first proposal to the official validation.
Validation of methods can also be organised by national or international entities, such as the European Centre for the Validation of Alternative Methods (ECVAM) of the EU Joint Research Centre (JRC), the Interagency Coordinating Committee on the Validation of Alternative Methods (ICCVAM) in the US, or the Japanese Centre for the Validation of Alternative Methods (JaCVAM). The public-private model of Pepper has advantages, such as the pooling public and private resources, establishing a centre of excellence, and providing access to funding for the validation process with the aim of improving chemical evaluation.
It should be noted that, besides a handful of ISO Standards, most test guidelines are not applicable to water quality testing. There is a need to adapt existing and develop new test guidelines for water quality testing. The authors are not aware of any intermediary organisation, such as Pepper, focusing water quality test guidelines.
Source: Authors and Philippe Hubert, Director of Pepper
3.5.3. International research partnerships
International research partnerships are essential and necessary in managing EDCs. They can be instrumental in sharing knowledge and data on EDCs, reducing uncertainties, supporting the transition to implementing new technologies and in supporting regulatory processes. Endocrine disruption in freshwater is still characterised by uncertainty which affects policy making. Ideally, international research partnerships also include researchers from low- and middle-income countries. Examples of research partnerships are the NORMAN Network (Box 3.9), the European Partnership for the Assessment of Risk from Chemicals (PARC) (Box 3.9), the Intersectoral Centre for Endocrine Disruptors Analysis (ICEDA) in Canada (Box 3.4) and the Global Water Research Coalition (GWRC). The GWRC has done extensive work on mainstreaming bioassays and establishing trigger values for water quality monitoring (Neale, Leusch and Escher, 2020[112]; Neale, Leusch and Escher, 2020[113]). The GWRC is a not-for-profit organisation with research organisations from Australia, Canada, France, Germany, Netherlands, Singapore, South Africa, United Kingdom, and the United States as members.
Box 3.9. Research partnerships in Europe: the NORMAN Network and PARC
Two European examples of research partnerships are the NORMAN Network and the European Partnership for the Assessment of Risk from Chemicals (PARC).
The NORMAN Network supports work on monitoring CECs. Its members comprise experts from academia, agencies and the private sector. The NORMAN Network resulted into an up-to-date database where members submit and share information on substances, suspect lists, ecotoxicology and monitoring data, including on bioassays and chemical occurrence. The network’s mission is to exchange information on CECs, improve data quality and promote synergies among research teams for a more efficient transfer of research findings to policymakers. Various activities of the network are linked to EDCs, such as the working group on bioassays and biomarkers in water quality monitoring. The working group aims to demonstrate the applicability of EBM as well as the use of effect-based trigger values. It also provides guidance documents, interlaboratory studies and communication with regulators.
PARC (European Partnership for the Assessment of Risk from Chemicals) is an institutional partnership based on regulatory drivers. The partners are from EU agencies (EEA, EFSA, ECHA) and academia. It is co-funded by the European Commission and EU Member States. PARC has several working parties such as the ones working on a common science-policy agenda, monitoring and exposure, hazard assessment and innovation in regulatory risk assessment. PARC is currently performing a pilot study for the environmental monitoring of PFAS and EDCs. The aims are to assess the background levels, characterise relevant exposure routes from diffuse and point sources and assess the effectiveness of management actions. The study will involve targeted and non-targeted analysis as well as EBM.
Source: Presentation by Dr Valeria Dulio of the French National Institute for Industrial Environment and Risks (Ineris) at the OECD Workshop on Developing Science-Informed Policy Responses to Curb Endocrine Disruption in Freshwater, 18-19 October 2022 (OECD, 2022[58])
3.5.4. Mainstreaming endocrine disruption on international agendas
A global issue of concern, endocrine disruption is appropriately addressed at an international level. It could therefore be appropriate to mainstream endocrine disruption on international science-policy agendas, such as agendas on One Health and chemicals. This includes the agreements made at the 2022 United Nations Environment Assembly to negotiate an internationally legal binding instrument by 2024 to end plastic pollution and to establish a Science-Policy Panel on Chemicals and Waste and to Prevent Pollution (Brack et al., 2022[74]). Similarly, EDCs and water quality more broadly, could take a more prominent position on the international One Health agenda.
3.6. Chapter conclusion
This chapter presented policies to tackle EDCs in freshwater. It documents existing policy approaches that intervene throughout the life cycle of EDCs. It also proposes interventions that are centred around the negative effects of EDCs on human and wildlife health, as the culprit chemical causing such negative effects is often unknown until further analysis is done. This chapter also makes the case for multilateral actions to improve monitoring, research and global action to tackle endocrine disruption.
The large number of potential endocrine active substances and their infinite number of mixtures, the diverse sources and entry-pathways into the aquatic environment and the need to make decisions under uncertainty, make policy design all the more complex. There is no single-best policy instrument to mitigate the negative effects of EDCs in water. Only a carefully designed package of policies has the potential to comprehensively reduce risks to human health and wildlife health. In addition, water quality assessments and monitoring are instrumental in safeguarding, to the best available knowledge, the integrity of ecosystems and human health. The next chapter therefore presents an action plan that supports the transition towards new monitoring methods that better capture the impacts of endocrine disruption.
References
[83] 1000-premiers-jours.fr (n.d.), 1000 Premiers Jours, https://www.1000-premiers-jours.fr/fr (accessed on 17 February 2023).
[73] Anderson, M. et al. (2019), “A governance framework for development and assessment of national action plans on antimicrobial resistance”, The Lancet Infectious Diseases, Vol. 19/11, pp. e371-e384, https://doi.org/10.1016/s1473-3099(19)30415-3.
[19] Andersson, N. et al. (2018), “Guidance for the identification of endocrine disruptors in the context of Regulations (EU) No 528/2012 and (EC) No 1107/2009”, EFSA Journal, Vol. 16/6, https://doi.org/10.2903/j.efsa.2018.5311.
[119] Attina, T. et al. (2016), “Exposure to endocrine-disrupting chemicals in the USA: a population-based disease burden and cost analysis”, The Lancet Diabetes & Endocrinology, Vol. 4/12, pp. 996-1003, https://doi.org/10.1016/S2213-8587(16)30275-3.
[95] Attina, T. et al. (2019), “Racial/ethnic disparities in disease burden and costs related to exposure to endocrine-disrupting chemicals in the United States: an exploratory analysis”, Journal of Clinical Epidemiology, Vol. 108, pp. 34-43, https://doi.org/10.1016/j.jclinepi.2018.11.024.
[52] Azizi, D. et al. (2022), “A comprehensive review on current technologies for removal of endocrine disrupting chemicals from wastewaters”, Environmental Research, Vol. 207, p. 112196, https://doi.org/10.1016/j.envres.2021.112196.
[79] Badry, A. et al. (2022), “Using environmental monitoring data from apex predators for chemicals management: towards harmonised sampling and processing of archived wildlife samples to increase the regulatory uptake of monitoring data in chemicals management”, Environmental Sciences Europe, Vol. 34/1, https://doi.org/10.1186/s12302-022-00664-6.
[74] Brack, W. et al. (2022), “One planet: one health. A call to support the initiative on a global science–policy body on chemicals and waste”, Environmental Sciences Europe, Vol. 34/1, https://doi.org/10.1186/s12302-022-00602-6.
[62] Brack, W. et al. (2018), “Towards a holistic and solution-oriented monitoring of chemical status of European water bodies: how to support the EU strategy for a non-toxic environment?”, Environmental Sciences Europe, Vol. 30/1, https://doi.org/10.1186/s12302-018-0161-1.
[61] California State Water Board (2018), Water quality control policy for recycled water, State Water Resources Control Board, California Environmental Protection Agency.
[121] Cordner, A. et al. (2021), The True Cost of PFAS and the Benefits of Acting Now, https://doi.org/10.1021/acs.est.1c03565.
[66] Creusot, N. et al. (2014), “Identification of Synthetic Steroids in River Water Downstream from Pharmaceutical Manufacture Discharges Based on a Bioanalytical Approach and Passive Sampling”, Environmental Science & Technology, Vol. 48/7, pp. 3649-3657, https://doi.org/10.1021/es405313r.
[27] Daneshian, M. et al. (2016), “Highlight report: Launch of a large integrated European in vitro toxicology project: EU-ToxRisk”, Archives of Toxicology, Vol. 90/5, pp. 1021-1024, https://doi.org/10.1007/s00204-016-1698-7.
[60] Danish EPA (2023), Analyse af fremtidig slamhåndtering. Til gavn for miljø og klima., The Danish Environmental Protection Agency.
[59] Danish EPA (2022), Non-targeted and suspect screening of sewage sludge. HITLIST4, The Danish Environmental Protection Agency.
[105] De Baat, M., S. Van Den Berg and T. Pronk (2022), Deltafact: Het toepassen van bioassays binnen Nederland, Foundation for Applied Water Research STOWA, Amersfoort, https://www.datocms-assets.com/38996/1644586511-deltafact-het-nieuwe-bioassay-spoor_v5.pdf (accessed on 7 February 2023).
[25] Dix, D. et al. (2007), “The ToxCast Program for Prioritizing Toxicity Testing of Environmental Chemicals”, Toxicological Sciences, Vol. 95/1, pp. 5-12, https://doi.org/10.1093/toxsci/kfl103.
[55] Domini, M. et al. (2022), “Sewage Sludge Quality and Management for Circular Economy Opportunities in Lombardy”, Applied Sciences, Vol. 12/20, p. 10391, https://doi.org/10.3390/app122010391.
[64] Drewes, J. et al. (2018), Monitoring Strategies for Constituents of Emerging Concern (CECs) in Recycled Water: Recommendations of a Science Advisory Panel Convened by the State Water Resources Control Board, State Water Resources Control Board, https://ftp.sccwrp.org/pub/download/DOCUMENTS/TechnicalReports/1032_CECMonitoringInRecycledWater.pdf (accessed on 7 February 2023).
[8] Dulio, V. and P. von der Ohe (2013), NORMAN Prioritisation framework for emerging substances, NORMAN Association N° W604002510, Verneuil en Halatte.
[85] Dussaux, D. et al. (2023), “Valuing a reduction in the risk of infertility: A large scale multi-country stated preference approach”, OECD Environment Working Papers, No. 215, OECD Publishing, Paris, https://doi.org/10.1787/7242509f-en.
[34] ECHA (2021), Assessment of regulatory needs - Group name: bisphenols, European Chemicals Agency.
[35] edlists.org (n.d.), Endocrine Disruptor Lists, https://edlists.org/ (accessed on 15 March 2023).
[18] EDSTAC (1998), Endocrine Disruptor Screening and Testing Advisory Committee (EDSTAC) Final Report, US EPA.
[104] Endocrine Society (2018), Endocrine-disrupting Chemicals: Position Statement, https://www.endocrine.org/advocacy/position-statements/endocrine-disrupting-chemicals (accessed on 18 December 2020).
[65] Environment Canada (2010), 2010 Pulp and Paper Environmental Effects Monitoring (EEM) Technical Guidance Document, Environment Canada, Ottawa, ON, Canada.
[63] European Commission (2022), Proposal for a Directive of the European Parliament and of the Council amending Directive 2000/60/EC establishing a framework for Community action in the field of water policy, Directive 2006/118/EC on the protection of groundwater against pollution and deterioration and Directive 2008/105/EC on environmental quality standards in the field of water policy, https://eur-lex.europa.eu/legal-content/EN/TXT/?uri=CELEX%3A52022PC0540 (accessed on 20 December 2022).
[47] European Commission (2022), Proposal for a Regulation of the European Parliament and of the Council amending Regulation (EC) No 1272/2008 of the European Parliament and of the Council on classification, labelling and packaging of substances and mixtures, https://environment.ec.europa.eu/system/files/2022-12/Proposal%20for%20a%20Regulation%20amending%20Regulation%20%28EC%29%20No%2012722008.pdf (accessed on 29 March 2023).
[36] European Commission (2022), The Endocrine Active Substances Information System, European Commission, Brussels, https://easis.jrc.ec.europa.eu/EASIS_Factsheet.pdf (accessed on 18 April 2023).
[77] European Commission (2020), “Fitness Check on endocrine disruptors”, Commission Staff Working Document.
[71] European Union (2020), “Directive (EU) 2020/2184 of the European Parliament and of the Council of 16 December 2020 on the quality of water intended for human consumption”, Offical Journal of the European Union, Vol. L 435.
[54] Fairbrother, A. et al. (2019), “Toward Sustainable Environmental Quality: Priority Research Questions for North America”, Environmental Toxicology and Chemistry, Vol. 38/8, pp. 1606-1624, https://doi.org/10.1002/etc.4502.
[100] Garibaldi, A. and N. Turner (2004), “Cultural keystone species: Implications for ecological conservation and restoration”, Ecology and Society, Vol. 9/3, https://doi.org/10.5751/ES-00669-090301.
[7] Gaston, L. et al. (2019), “Prioritization Approaches for Substances of Emerging Concern in Groundwater: A Critical Review”, Environmental Science & Technology, Vol. 53/11, pp. 6107-6122, https://doi.org/10.1021/acs.est.8b04490.
[103] Godfray, H. et al. (2019), “A restatement of the natural science evidence base on the effects of endocrine disrupting chemicals on wildlife”, Proceedings of the Royal Society B: Biological Sciences, Vol. 286/1897, https://doi.org/10.1098/rspb.2018.2416.
[5] Götz, C. et al. (2009), “Targeting aquatic microcontaminants for monitoring: exposure categorization and application to the Swiss situation”, Environmental Science and Pollution Research, Vol. 17/2, pp. 341-354, https://doi.org/10.1007/s11356-009-0167-8.
[90] Govarts, E. et al. (2023), “Harmonized human biomonitoring in European children, teenagers and adults: EU-wide exposure data of 11 chemical substance groups from the HBM4EU Aligned Studies (2014–2021)”, International Journal of Hygiene and Environmental Health, Vol. 249, p. 114119, https://doi.org/10.1016/j.ijheh.2023.114119.
[50] Government of Belgium (2022), Projet de Plan d’Action National sur les Perturbateurs Endocriniens 2022-2026, Federal Public Service (FPS) Health, Food Chain Safety and Environment, https://www.health.belgium.be/sites/default/files/uploads/fields/fpshealth_theme_file/naped_fr_cp.pdf (accessed on 28 March 2023).
[92] Government of Belgium (2018), National Action Plan Pesticides 2018-2022, Federal Public Service (FPS) Health, Food Chain Safety and Environment, https://fytoweb.be/sites/default/files/content/reduction/program-napan-belgium-20200511.pdf (accessed on 28 March 2023).
[51] HELCOM (2022), Micropollutants in wastewater and sewage sludge. Baltic Sea Environment Proceedings Proceedings No. 185., Baltic Marine Environment Protection Commission – Helsinki Commission, Helsinki.
[111] Hollender, J. et al. (2019), “High resolution mass spectrometry-based non-target screening can support regulatory environmental monitoring and chemicals management”, Environmental Sciences Europe, Vol. 31/1, https://doi.org/10.1186/s12302-019-0225-x.
[117] Hotchkiss, A. et al. (2008), Fifteen years after “wingspread” - Environmental endocrine disrupters and human and wildlife health: Where we are today and where we need to go, https://doi.org/10.1093/toxsci/kfn030.
[37] Institute of Mathematical Sciences (n.d.), Database of Endocrine Disrupting Chemicals and their Toxicity Profiles, The Institute of Mathematical Sciences, Tamil Nadu, https://cb.imsc.res.in/deduct/ (accessed on 18 April 2023).
[17] IPCP (2017), Overview Report III: Existing national, regional, and global regulatory frameworks addressing Endocrine Disrupting Chemicals (EDCs), https://wedocs.unep.org/bitstream/handle/20.500.11822/25636/edc_report3.pdf?sequence=1&isAllowed=y (accessed on 28 November 2022).
[82] IRSN (2022), Baromètre 2022 la perception des risques et de la sécurité par les Français - l’analyse, Institut de radioprotection et de sûreté nucléaire.
[81] IRSN (2018), Baromètre IRSN La perception des risques et de la sécurité par les Français 2018 - les essentiels, Institut de radioprotection et de sûreté nucléaire.
[4] James, A., A. Kroll and C. Minier (2023), “Towards a better consideration of endocrine disruption within the technical guidance for deriving environmental quality standards”, Regulatory Toxicology and Pharmacology, Vol. 143, p. 105457, https://doi.org/10.1016/j.yrtph.2023.105457.
[6] Johnson, A. et al. (2017), “An alternative approach to risk rank chemicals on the threat they pose to the aquatic environment”, Science of The Total Environment, Vol. 599-600, pp. 1372-1381, https://doi.org/10.1016/j.scitotenv.2017.05.039.
[39] Karthikeyan, B. et al. (2021), “DEDuCT 2.0: An updated knowledgebase and an exploration of the current regulations and guidelines from the perspective of endocrine disrupting chemicals”, Chemosphere, Vol. 267, p. 128898, https://doi.org/10.1016/j.chemosphere.2020.128898.
[38] Karthikeyan, B. et al. (2019), “A curated knowledgebase on endocrine disrupting chemicals and their biological systems-level perturbations”, Science of The Total Environment, Vol. 692, pp. 281-296, https://doi.org/10.1016/J.SCITOTENV.2019.07.225.
[31] Kassotis, C. et al. (2020), “Endocrine-disrupting chemicals: economic, regulatory, and policy implications”, The Lancet Diabetes & Endocrinology, Vol. 8/8, pp. 719-730, https://doi.org/10.1016/S2213-8587(20)30128-5.
[109] Kienle, C. et al. (2015), Methoden zur Beurteilung der Wasserqualität anhand von ökotoxikologischen Biotests: Ergebnisse einer Literaturrecherche und einer Expertenbefragung, Ecotox Centre, Dübendorf, https://www.centreecotox.ch/media/90556/2015_kienle_biotests_methodenempfehlung.pdf (accessed on 7 February 2023).
[48] Kim, H., H. Lee and S. Yoo (2018), “Public willingness to pay for endocrine disrupting chemicals-free labelling policy in Korea”, Applied Economics, Vol. 51/2, pp. 131-140, https://doi.org/10.1080/00036846.2018.1494803.
[116] Kortenkamp, A. and M. Faust (2018), “Regulate to reduce chemical mixture risk”, Science, Vol. 361/6399, pp. 224-226, https://doi.org/10.1126/science.aat9219.
[26] Krewski, D. et al. (2010), “Toxicity Testing in the 21st Century: A Vision and a Strategy”, Journal of Toxicology and Environmental Health, Part B, Vol. 13/2-4, pp. 51-138, https://doi.org/10.1080/10937404.2010.483176.
[57] Kumar, R. et al. (2022), “Emerging contaminants in biosolids: Presence, fate and analytical techniques”, Emerging Contaminants, Vol. 8, pp. 162-194, https://doi.org/10.1016/j.emcon.2022.03.004.
[3] Kurek, J. et al. (2019), “Ecological Legacy of DDT Archived in Lake Sediments from Eastern Canada”, Environmental Science & Technology, Vol. 53/13, pp. 7316-7325, https://doi.org/10.1021/acs.est.9b01396.
[84] Langlois, V. et al. (2022), “Twenty-five years beyond “Our Stolen Future”: How did we progress as an international society on screening and regulating Endocrine Disrupting Chemicals (EDCs)?”, Environmental Research, Vol. 210, p. 112849, https://doi.org/10.1016/j.envres.2022.112849.
[21] Maffini, M. and L. Vandenberg (2022), “Failure to Launch: The Endocrine Disruptor Screening Program at the U.S. Environmental Protection Agency”, Frontiers in Toxicology, Vol. 4, https://doi.org/10.3389/ftox.2022.908439.
[118] Malits, J., M. Naidu and L. Trasande (2022), “Exposure to Endocrine Disrupting Chemicals in Canada: Population-Based Estimates of Disease Burden and Economic Costs”, Toxics, Vol. 10/3, p. 146, https://doi.org/10.3390/toxics10030146.
[78] Ministère de la transition écologique et solidaire (2019), Second National strategy on endocrine disruptors – 2019-2022: Action Plan, Ministère de la transition écologique et solidaire and the Ministère des Solidarités et de la Santé, Paris, https://www.ecologie.gouv.fr/ (accessed on 31 May 2022).
[44] MoE Japan (2022), Further actions to endocrine disrupting effects of chemical substances - EXTEND 2022 (in japanese), Ministry of the Environment of Japan.
[43] MoE Japan (2016), Further Actions to Endocrine Disrupting Effects of Chemical Substances - EXTEND 2016 - (Tentative translation), Ministry of the Environment of Japan.
[42] MoE Japan (2010), Further Actions to Endocrine Disrupting Effects of Chemical Substances - EXTEND2010 - (Tentative Translation), Ministry of the Environment of Japan.
[41] MoE Japan (2005), Ministry of the Environment’s Perspectives on Endocrine Disrupting Effects of Substances, Ministry of the Environment of Japan.
[40] MoE Japan (1998), Strategic Programs on Environmental Endocrine Disruptors ’98 (SPEED ’98), Ministry of the Environment of Japan.
[97] Morrens, B. et al. (2012), “Social distribution of internal exposure to environmental pollution in Flemish adolescents”, International Journal of Hygiene and Environmental Health, Vol. 215/4, pp. 474-481, https://doi.org/10.1016/j.ijheh.2011.10.008.
[86] Mourato, S. et al. (2023), “Valuing the avoidance of IQ losses in children: A large scale multi-country stated preference approach”, OECD Environment Working Papers, No. 219, OECD Publishing, Paris, https://doi.org/10.1787/71574eb4-en.
[107] Neale, P. et al. (2022), “Effect-based monitoring to integrate the mixture hazards of chemicals into water safety plans”, Journal of Water and Health, Vol. 20/12, pp. 1721-1732, https://doi.org/10.2166/wh.2022.165.
[112] Neale, P., F. Leusch and B. Escher (2020), Effect Based Monitoring in Water Safety Planning, Global Water Research Coalition, London.
[113] Neale, P., F. Leusch and B. Escher (2020), “Use of effect-based monitoring for the assessment of risks of low-level mixtures of chemicals in water on man and the environment”, Global Water Research Coalition, http://www.globalwaterresearchcoalition.net/_r4284/media/system/attrib/file/825/GWRC_EBM%20in%20WST_Factsheet%203_1%206%20April%202020.pdf (accessed on 23 December 2022).
[102] NIWA (n.d.), Cultural Keystone Species: Co-management and restoration of our freshwater taonga species, National Institute of Water and Atmospheric Research, https://niwa.co.nz/te-kuwaha/research-projects/cultural-keystone-species-co-management-and-restoration-of-our-freshwater-taonga-species (accessed on 28 March 2023).
[101] Noble, M. et al. (2016), “Culturally significant fisheries: keystones for management of freshwater social-ecological systems”, Ecology and Society, Vol. 21/2, https://doi.org/10.5751/es-08353-210222.
[49] Nordic Swan (n.d.), Chemicals harmful to health, Nordic Swan Ecolabel, https://www.nordic-ecolabel.org/nordic-swan-ecolabel/environmental-aspects/chemicals-nano-and-microplastic/chemicals-harmful-to-health/ (accessed on 30 March 2023).
[53] NORMAN Network and Water Europe (2019), “Contaminants of Emerging Concern in Urban Wastewater: Joint NORMAN and Water Europe Position Paper”.
[110] NWRI (2020), Bioanalytical tools for detection and quantification of estrogenic and dioxin-like chemicals in water recycling and reuse, National Water Research Institute.
[16] OECD (2023), Workshop Report on Flexible Food-Grade Plastic Packaging, OECD Series on Risk Management, No. 76, Environment, Health and Safety, Environment Directorate, OECD.
[1] OECD (2022), Government risk management approaches used for chemicals management, OECD Series on Risk Management No. 74, Environment, Health and Safety, Environment Directorate, OECD.
[69] OECD (2022), OECD Environment, Health and Safety Publications Series on Risk Management No. 70 BAT Project Activity – Best Available Techniques (BAT) to Prevent and Control Mercury Releases to Land and Water, OECD, Paris, https://mercuryconvention.org/sites/default/files/inline-files/OECD_Releases_ENV-CBC-MONO%282022%2917.en_.pdf.
[58] OECD (2022), Summary Note of the OECD Workshop on Developing Science-Informed Policy Responses to Curb Endocrine Disruption in Freshwater, 18-19 October 2022, OECD, Paris, https://www.oecd.org/water/Summary-note-endocrine-disruption-in-freshwater.pdf (accessed on 6 February 2023).
[15] OECD (2021), Policies to Reduce Microplastics Pollution in Water: Focus on Textiles and Tyres, OECD Publishing, Paris, https://doi.org/10.1787/7ec7e5ef-en.
[70] OECD (2019), Guiding Principles and Key Elements for Establishing a Weight of Evidence for Chemical Assessment, Series on Testing and Assessment No. 311, Environment, Health and Safety Division, OECD Environment Directorate, https://www.oecd.org/chemicalsafety/risk-assessment/guiding-principles-and-key-elements-for-establishing-a-weight-of-evidence-for-chemical-assessment.pdf.
[13] OECD (2019), Pharmaceutical Residues in Freshwater: Hazards and Policy Responses, OECD Studies on Water, OECD Publishing, Paris, https://doi.org/10.1787/c936f42d-en.
[106] OECD (2019), Saving Costs in Chemicals Management: How the OECD Ensures Benefits to Society, OECD Publishing, Paris, https://doi.org/10.1787/9789264311718-en.
[46] OECD (2018), “Larval Amphibian Growth and Development Assay (LAGDA) (OECD TG 241)”, in Revised Guidance Document 150 on Standardised Test Guidelines for Evaluating Chemicals for Endocrine Disruption, OECD Publishing, Paris, https://doi.org/10.1787/9789264304741-15-en.
[45] OECD (2018), “Medaka Extended One-Generation Reproduction Test (MEOGRT) (OECD TG 240)”, in Revised Guidance Document 150 on Standardised Test Guidelines for Evaluating Chemicals for Endocrine Disruption, OECD Publishing, Paris, https://doi.org/10.1787/9789264304741-18-en.
[20] OECD (2018), Revised Guidance Document 150 on Standardised Test Guidelines for Evaluating Chemicals for Endocrine Disruption, OECD Series on Testing and Assessment, No. 150, OECD Publishing, Paris, https://doi.org/10.1787/9789264304741-en.
[14] OECD (2017), Diffuse Pollution, Degraded Waters: Emerging Policy Solutions, OECD Studies on Water, OECD Publishing, Paris, https://doi.org/10.1787/9789264269064-en.
[29] OECD (2017), Guidance Document for the Use of Adverse Outcome Pathways in Developing Integrated Approaches to Testing and Assessment (IATA), OECD Series on Testing and Assessment, No. 260, OECD Publishing, Paris, https://doi.org/10.1787/44bb06c1-en.
[33] OECD (2017), Guidance on Grouping of Chemicals, Second Edition, OECD Series on Testing and Assessment, No. 194, OECD Publishing, Paris, https://doi.org/10.1787/9789264274679-en.
[12] OECD (2017), OECD Environmental Performance Reviews: Korea 2017, OECD Environmental Performance Reviews, OECD Publishing, Paris, https://doi.org/10.1787/9789264268265-en.
[11] OECD (2016), OECD Council Recommendation on Water, OECD, Paris.
[68] OECD (1998), OECD series on principles of good laboratory practice and compliance monitoring Number 1. OECD principles on good laboratory practice (as revised in 1997).
[72] Özçelik, E. et al. (2022), “A comparative assessment of action plans on antimicrobial resistance from OECD and G20 countries using natural language processing”, Health Policy, Vol. 126/6, pp. 522-533, https://doi.org/10.1016/j.healthpol.2022.03.011.
[76] Özçelik, E. et al. (2022), A comparative assessment of action plans on antimicrobial resistance from OECD and G20 countries using natural language processing, https://doi.org/10.1016/j.healthpol.2022.03.011.
[96] Pumarega, J. et al. (2016), “Number of Persistent Organic Pollutants Detected at High Concentrations in Blood Samples of the United States Population”, PLOS ONE, Vol. 11/8, p. e0160432, https://doi.org/10.1371/journal.pone.0160432.
[94] Ruiz, D. et al. (2017), “Disparities in Environmental Exposures to Endocrine-Disrupting Chemicals and Diabetes Risk in Vulnerable Populations”, Diabetes Care, Vol. 41/1, pp. 193-205, https://doi.org/10.2337/dc16-2765.
[114] Saaristo, M. et al. (2018), “Direct and indirect effects of chemical contaminants on the behaviour, ecology and evolution of wildlife”, Proceedings of the Royal Society B, Vol. 285/1885, https://doi.org/10.1098/RSPB.2018.1297.
[67] Sanchez, W. et al. (2011), “Adverse effects in wild fish living downstream from pharmaceutical manufacture discharges”, Environment International, Vol. 37/8, https://doi.org/10.1016/j.envint.2011.06.002.
[87] Ščasný, M., I. Zvěřinová and D. Dussaux (2023), “Valuing a reduction in the risk of very low birth weight: A large scale multi-country stated preference approach”, OECD Environment Working Papers, No. 217, OECD Publishing, Paris, https://doi.org/10.1787/dfd159a1-en.
[98] Schoeters, G. et al. (2022), “Internal exposure of Flemish teenagers to environmental pollutants: Results of the Flemish Environment and Health Study 2016–2020 (FLEHS IV)”, International Journal of Hygiene and Environmental Health, Vol. 242, p. 113972, https://doi.org/10.1016/j.ijheh.2022.113972.
[56] Sichler, T. et al. (2022), “Future nutrient recovery from sewage sludge regarding three different scenarios - German case study”, Journal of Cleaner Production, Vol. 333, p. 130130, https://doi.org/10.1016/j.jclepro.2021.130130.
[10] Smital, T. et al. (2012), “Prioritisation of organic contaminants in a river basin using chemical analyses and bioassays”, Environmental Science and Pollution Research, Vol. 20/3, pp. 1384-1395, https://doi.org/10.1007/s11356-012-1059-x.
[32] Swedish Government Inquiries (2019), Future chemical risk management. Accounting for combination effects and assessing chemicals in groups. The report of the Committee of Combination of effects and assessing chemicals in groups., Swedish Government Inquiries, https://www.government.se/4adb1a/contentassets/ee36b3e49c354bb8967f3a33a2d5ca50/future-chemical-risk-management---accounting-for-combination-effects-and-assessing-chemicals-in-groups-sou-201945 (accessed on 22 July 2022).
[91] Swedish National Food Administration (2008), Advice about food for you who are pregnant, Swedish National Food Administration, Uppsala, https://www.livsmedelsverket.se/globalassets/publikationsdatabas/andra-sprak/rad-om-mat-till-dig-som-ar-gravid/advice-about-food-for-you-who-are-pregnant.pdf (accessed on 28 March 2023).
[120] Trasande, L. et al. (2016), “Burden of disease and costs of exposure to endocrine disrupting chemicals in the European Union: an updated analysis”, Andrology, Vol. 4/4, pp. 565-572, https://doi.org/10.1111/andr.12178.
[99] Tyrrell, J. et al. (2013), “Associations between socioeconomic status and environmental toxicant concentrations in adults in the USA: NHANES 2001–2010”, Environment International, Vol. 59, pp. 328-335, https://doi.org/10.1016/j.envint.2013.06.017.
[22] U.S. EPA (2021), Report No. 21-E-0186 EPA’s Endocrine Disruptor Screening Program Has Made Limited Progress in Assessing Pesticides, US EPA Office of the Inspector General, Washington, D.C, https://www.epa.gov/office-inspector-general/report-epas-endocrine-disruptor-screening-program-has-made-limited (accessed on 29 November 2022).
[88] U.S. EPA (2019), Guidelines for Human Exposure Assessment, Risk Assessment Forum, United States Environmental Protection Agency.
[24] U.S. EPA (2013), Endocrine Disruptor Screening Program: Final Second List of Chemicals and Substances for Tier 1 Screening, United States Environmental Protection Agency, Washington, DC, https://www.regulations.gov/document/EPA-HQ-OPPT-2009-0477-0074 (accessed on 18 April 2023).
[23] U.S. EPA (n.d.), Endocrine Disruptor Screening Program Tier 1 Screening Determinations and Associated Data Evaluation Records.
[93] U.S. EPA (n.d.), Environmental Justice, https://www.epa.gov/environmentaljustice (accessed on 30 March 2023).
[89] U.S. EPA (n.d.), Exposure Assessment Tools by Lifestages and Populations - Highly Exposed or Other Susceptible Population Groups, https://www.epa.gov/expobox/exposure-assessment-tools-lifestages-and-populations-highly-exposed-or-other-susceptible (accessed on 30 March 2023).
[2] UNEP (2019), UNEP Guidance on Risk Reduction Tools for Chemicals Control, United Nations Environment Programme, Nairobi.
[28] Vinken, M. et al. (2021), “Safer chemicals using less animals: kick-off of the European ONTOX project”, Toxicology, Vol. 458, p. 152846, https://doi.org/10.1016/j.tox.2021.152846.
[9] von der Ohe, P. et al. (2011), “A new risk assessment approach for the prioritization of 500 classical and emerging organic microcontaminants as potential river basin specific pollutants under the European Water Framework Directive”, Science of The Total Environment, Vol. 409/11, pp. 2064-2077, https://doi.org/10.1016/j.scitotenv.2011.01.054.
[108] WFD (2009), Guidance Document No. 19 Guidance on Surface Water Chemical Monitoring Under the Water Framework Directive - Common Implementation Strategy for the Water Framework Directive (2000/60/EC), WFD.
[80] WHO (2015), Global Action Plan on Antimicrobial Resistance. Geneva, WHO, 2015, PP:1-28. Available from: http://www.wpro.who.int/entity/drug_resistance/resources/global_action_plan_eng.pdf.
[75] WHO (n.d.), Library of AMR national action plans, https://www.who.int/teams/surveillance-prevention-control-AMR/national-action-plan-monitoring-evaluation/library-of-national-action-plans (accessed on 11 August 2023).
[30] WHO-UNEP (2013), State of the Science of Endocrine Disrupting Chemicals 2012, Inter-Organisation Programme for the Sound Management of Chemicals, United Nations Environment Programme and the World Health Organization, https://apps.who.int/iris/handle/10665/78101.
[115] Windsor, F., S. Ormerod and C. Tyler (2018), “Endocrine disruption in aquatic systems: up-scaling research to address ecological consequences”, Biological Reviews, Vol. 93/1, https://doi.org/10.1111/brv.12360.
Annex 3.A. Suggestions for an international research agenda
Understanding the impacts of EDC pollution at ecosystem level. Current research is mostly focused on the effects on individual species. At higher levels of biological organisation (i.e., the effects of EDCs to species, communities and ecosystems), our understanding declines. The cascade of consequences of EDCs on the trophic system, including the aquatic food web, is uncertain (Saaristo et al., 2018[114]; Windsor, Ormerod and Tyler, 2018[115]).
Characterising and prioritising mixtures that impact water quality. The complexity of managing and regulating chemical mixtures lies in the combined effect of chemicals, the multitude of sources (agriculture, urban wastewater, industry, landfills), and uncertainties surrounding the effects of mixtures (Kortenkamp and Faust, 2018[116]).
Cross-species comparisons - using sentinel species such as zebrafish to draw conclusions on the impacts on other organisms such as humans - are a limitation in understanding the true impacts on other species. “Laboratory species may not always be relevant to the species of concern and data from human health risk assessments may not accurately reflect the risk to fish and wildlife” (Hotchkiss et al., 2008[117]). However, this uncertainty may not hinder any monitoring efforts to detect potential risks in water, as such effects can be validated through further analysis.
Making an economic case for controlling EDCs in freshwater. The cost of inaction, or acting after the damage is done, is likely more expensive than preventive measures, but this has not been established with certainty. While there have been attempts to define the economic costs of endocrine disruption, the data is characterised by uncertainty and most likely represents an underestimate of the actual costs (Malits, Naidu and Trasande, 2022[118]; Attina et al., 2016[119]; Trasande et al., 2016[120]; Cordner et al., 2021[121]). Moreover, the economic costs have not been defined when it comes to the loss of biodiversity and ecosystem services caused by endocrine disruption. A strengthened economic rationale informs the cost-effectiveness of policy decisions such as developing routine monitoring programmes and mitigation actions, such as upgrading wastewater treatment plants.
Bioassay development and EDC research have traditionally focused on the EATS (Estrogen, Androgen, Thyroid and Steroidogenesis) modalities. However, other hormones and endocrine axes are somewhat neglected. One example is the glucocorticoid receptor, for which many studies detect activity in freshwater samples. Moreover, even within the EATS modalities, not all the axes are equally developed. On the one hand, bioassays for estrogenic effects are in a very advanced state of development and could easily be deployed. In contrast, there are currently no standardised in vitro bioassays for the thyroid axis as pointed out by some participants. However, thyroid disruption is well studied and is notably known for disrupting metamorphosis in amphibians. More efforts are needed to develop and validate bioassays for EATS and non-EATS modalities (OECD, 2022[58]).