This chapter applies a well-being lens to the agricultural sector and, more broadly, to food systems. It first proposes a change in perspective towards policy making that places climate mitigation, the protection of the environment and human health at the same level of priority as economic objectives. Such an approach highlights the synergies and trade-offs between climate and other well-being priorities, with some examples presented in the chapter. The second part of the chapter proposes a set of indicators that can contribute to tracking progress and steering policies towards the multiple priorities discussed. It then discusses the relationship of these indicators proposed with the indicators attached to the Sustainable Development Goals and the OECD Framework for Measuring Well-being and Progress, and their potential value in providing a more comprehensive picture of synergies and trade-offs between climate and other well-being goals.
Accelerating Climate Action
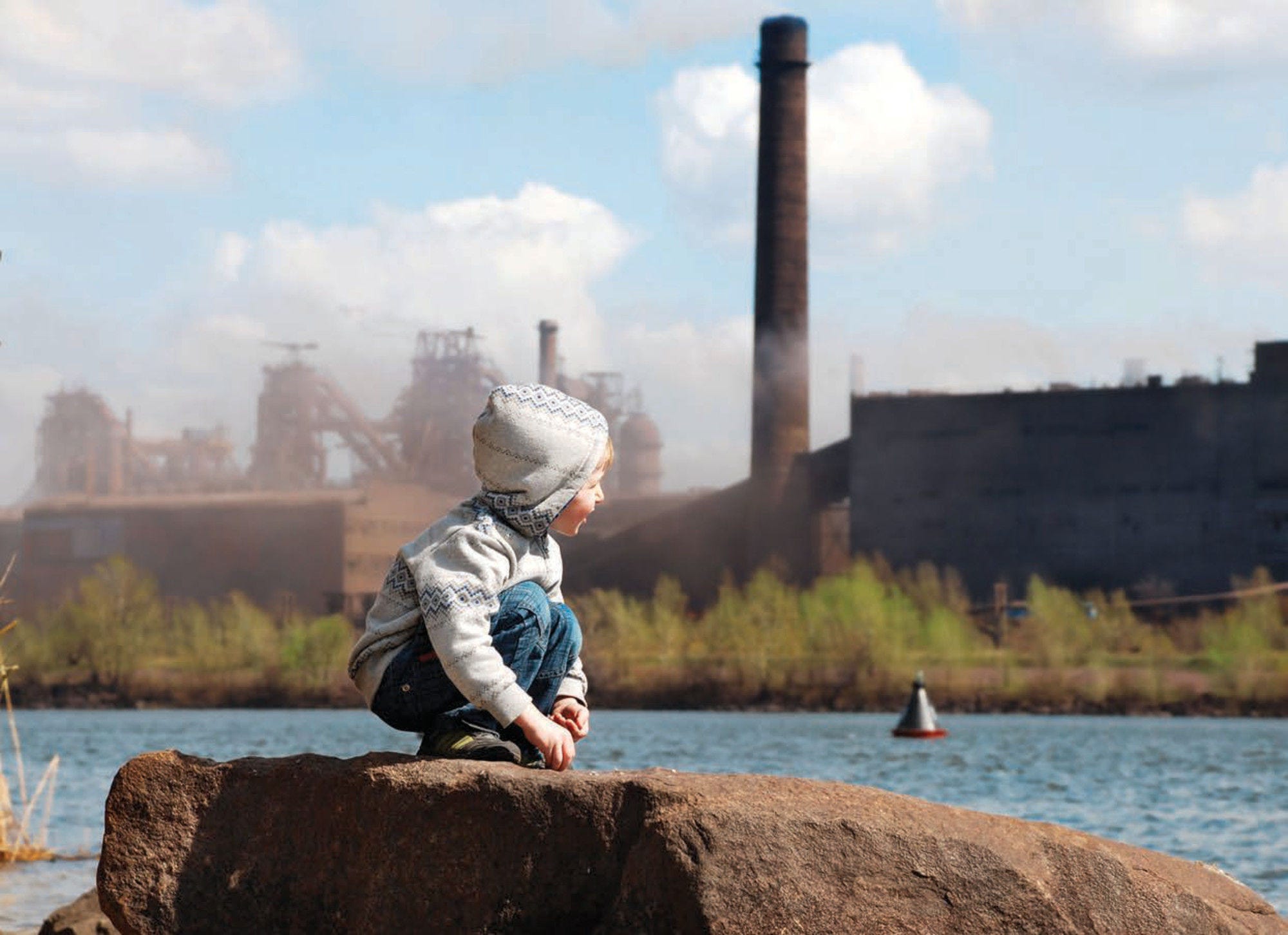
6. Creating a sustainable food system
Abstract
In Brief
Agriculture has achieved major success in fighting hunger, feeding the world and contributing to economic development, including by providing employment to 28% of workers worldwide. Agriculture also provides agro-environmental services to society, such as flood risk mitigation, and resilience to droughts. However, this success has come at a price. Many of the undesirable impacts on the environment and on human health stem from the intensification of farming practices to meet growing global food demand (e.g. excessive use of fertilisers, pesticides and antibiotics).
The food system is a major contributor to climate change, responsible for around 30% of global GHG emissions, including methane from ruminants’ digestion and paddy rice cultivation, nitrous oxide emissions arising from fertilisers and animal waste and indirect emissions from land-use change. Agriculture uses one-third of the land surface and is a major driver of deforestation. If unchecked, climate change impacts such as heatwaves, droughts and floods will threaten food security and the viability of current agricultural production patterns.
Furthermore, the current food system does not provide a healthy diet for everyone, even if it has the necessary capacity and produces sufficient total calories. Malnutrition remains a global issue and obesity rates are growing: 159 million children under the age of 5 suffer from stunted growth; 1.9 billion adults are overweight or obese. Meanwhile, one-third of the produced food is wasted or lost.
Importantly, agriculture and forestry have the potential to remove carbon dioxide from the atmosphere, which could significantly increase the feasibility of stringent mitigation goals. The most efficient options include afforestation, land restoration and the development of sustainable bioenergy. The latter can contribute to mitigation in other sectors but require rigorous life-cycle assessment to avoid damaging land-use changes and associated GHG emissions and biodiversity loss.
A shift in perspective is needed to better integrate growing challenges to the sustainability of the food system. Economic criteria (GDP, trade, farmers’ income) are currently the main drivers for decisions in agriculture and associated food systems. Integrating wider social objectives (e.g. healthy diets, climate, sustainable resource management) as priorities is key. Addressing the sustainability of the food sector also requires examining the whole food value chain, including the demand side as well as the institutions and markets in which these are embedded.
Applying a well-being lens can help governments make visible the hidden costs of the current food system and identify the potential to achieve synergies (i.e. health, improved environment, carbon storage) and better manage potential trade-offs (e.g. jobs, food access and affordability) between climate and broader well-being goals. For instance, a particular focus on workers’ protection and training might facilitate the sector’s transition.
New indicators will be needed to measure and monitor performance and to facilitate the achievement of two-way alignment between climate and other well-being goals. For example, the development of reliable indicators on food accessibility and affordability, especially for lower-income households, would help decision-makers to address relevant trade-offs, thus improving two-way alignment. To inform policy development, performance measurement also needs to evolve towards full-cost accounting. This shift in perspective offers a framework for designing more efficient and more comprehensive policies for the food system.
Infographic 6.1. Creating a sustainable food system

6.1. Introduction
Chapter 1 argues for applying a well-being lens to policy making as a central step to reflecting and assessing the synergies and trade-offs of climate policies, and thus for achieving a two-way alignment1 between climate and broader well-being objectives. Adopting a well-being lens implies that:
Societal goals are defined in terms of well-being outcomes (including limiting climate change through mitigation) and are systematically reflected in decision-making across the economy.
The decisions taken consider multiple well-being objectives, rather than focusing on solving a single objective or a very narrow range of objectives.
The relations between the different sectors and elements of the system in which a policy intervenes are well understood.
This chapter builds on that discussion and applies a well-being lens to the agriculture sector and the food system.
The agriculture sector of the 21st century represents one of the most important achievements of human civilisations in terms of producing large amounts of relatively affordable food that is theoretically more than sufficient to feed the world’s growing population. Yet the food system2 as a whole also faces some major challenges, both in terms of environmental sustainability and human well-being.
The negative impact of the current food system on aspects of well-being, such as health or the environment, have been underestimated as the sector has been mainly steered by income, markets and productivity goals. The current food system puts pressures on the very resources (water, soil quality) and ecosystems on which it depends, threatening its own sustainability. Many of these pressures are linked to the intensification of farming practices to meet growing global food demand (e.g. excessive use of fertilisers, pesticides and antibiotics, industrial livestock systems, unsustainable grazing), the specialisation and uniformity of landscapes, and land conversion for agriculture (Hardelin and Lankoski, 2018[1]).
Agricultural production is responsible for around 10‑12% of global greenhouse gases (GHGs).3 The combined agriculture, forestry and land-use sectors are responsible for around one-quarter of global GHG emissions (Smith et al., 2014[2]). Most of the direct emissions from agriculture are due to methane from enteric fermentation of ruminants (39% of the global GHG emissions from agriculture in 2016, in CO2eq4), manure applied to pasture (16%) and rice cultivation (10%). Synthetic fertilisers, which emit nitrous oxide (N2O) into the atmosphere, account for 13% of GHG emissions from the agricultural sector world-wide (i.e. just under 2% of global GHG emissions). In parallel, the net carbon sink caused by environmental changes due to human activity, including the increased fertilisation due to more carbon in the atmosphere, is the equivalent of 29% of total human-caused CO2 emissions (Arneth et al., 2019[3]).
Climate mitigation is vital for the food system: a 2 degree Celsius (°C) increase in world temperature above the late 20th-century level would pose major risks for food security (Field et al., 2014[4]). With the potential for yields to be negatively affected (though heterogeneously world-wide, as some regions may actually benefit), surface water and groundwater resources will decrease, endangering the viability of irrigation systems in some parts of the world. More frequent and intense extreme-weather events (heat waves, extreme precipitation, coastal flooding) related to climate change could also threaten agriculture production.
Crucially, the importance of the agriculture sector for climate change mitigation lies not only in the potential to reduce GHG emissions, but also in its potential contribution to removing carbon dioxide from the atmosphere. According to the Intergovernmental Panel on Climate Change (IPCC) (IPCC, 2018[5]), smoother emission pathways for a 1.5°C scenario include net negative emissions by the second half of the 21st century. The most efficient options for these types of emissions lie in agriculture and forestry, notably through afforestation and the development of sustainable bioenergies, which contribute to mitigation in other sectors.
Bioenergies have the potential to mitigate climate change, under certain conditions, and are therefore included in many low-emission development strategies (see, for instance, (Popp et al., 2017[6])). However, a large range of literature shows that the production of biofuels might emit more GHGs than they store, notably owing to changes in land use (see (Fargione et al., 2008[7]) and (Searchinger and Heimlich, 2015[8])). The most recent report of the IPCC on climate change and land (Arneth et al., 2019[3]) highlights the fact that the land use for bioenergy can conflict with food production and hence jeopardise food security, with higher population growth increasing this risk. The deployment of land-based mitigation measures such as bioenergy and afforestation, is therefore limited. In the case of bioenergy, land-use competition could be alleviated by using advanced (second- and third-generation) biofuels. Ensuring the sustainability of bioenergies through a life-cycle assessment of their emissions is therefore crucial.
Agricultural production also affects well-being more broadly, notably through the pressure exerted on biodiversity, and thus on a number of ecosystem services,5 such as pollination or natural control of plant pests.6 Land-use change owing to the expansion of arable land is also an important contributor to carbon dioxide (CO2) emissions (IPCC, 2018[5]) and biodiversity loss (Díaz et al., 2019[9]) (Newbold et al., 2014[10]), at a time when agricultural land already covers one-third of the terrestrial land surface (Díaz et al., 2019[9]). To what extent these pressures can be managed, while also providing adequate diets for a growing population and meeting the potentially fast-growing demand for bioenergy products, is the key challenge.
Stimulating yields in order to provide enough commodities while limiting land use can be part of the answer. According to (Arneth et al., 2019[3]), increasing food productivity could contribute significantly to the mitigation of GHG emissions in agriculture, as it would reduce the pressure for agriculture land expansion. Sustainable intensification, which consists in restoring already degraded land to increase food production and carbon sequestration, would benefit both climate and ecosystems, but its feasibility varies significantly according to the ecosystem and the area. It should be noted, however, that higher yields do not necessarily result in less agricultural expansion. (Rudel et al., 2009[11]) find that agricultural intensification has not generally led to a country stabilising or reducing its cropland area (see also (Ewers et al., 2009[12])): the predominant experience is that an increase in productivity has gone hand in hand with an increase in the agricultural area. Moreover, the higher use of inputs might compensate the climate benefits of land sparing, as showed the intensified production of rice and pigs in Vietnam in the last two decades (Arneth et al., 2019[3]).
Furthermore, the current food system does not entirely succeed in meeting the objective of providing a healthy diet for everyone, even though it has the necessary capacity and produces sufficient total calories. The World Health Organization (WHO) (WHO, 2018[13]) estimates that 452 million adults world-wide are underweight, and 159 million children under the age of five suffer from stunted growth; meanwhile, 1.9 billion adults are overweight or suffer from obesity. Considering the criticality of the food system to human development and the challenges it still faces, “zero hunger” is the second of the 17 Sustainable Development Goals (SDGs) (see Section 6.3).
The importance of the agriculture sector for climate change mitigation lies not only in the potential to reduce emissions, but also in its potential contribution to removing carbon dioxide from the atmosphere.
Policy makers have becoming increasingly aware in recent years of the new challenges facing agriculture, and efforts have been made to integrate environmental objectives in agriculture policies. The 2016 Declaration on Better Policies to Achieve a Productive, Sustainable and Resilient Global Food System, signed by the ministers and representatives of 47 countries, states several shared goals for agriculture and the food sector, including:
access to safe, healthy and nutritious food
enabling producers everywhere, big and small, male and female, to operate in an open and transparent global trading system, and to seize available market opportunities to improve their standards of living
sustainable productivity and resource use
the provision of public goods and ecosystem services
inclusive growth and development.
However, no strong global shift in agriculture policies or their management has been observed since (see, for instance, (OECD, 2019[14]), which monitors public support for agriculture).
Putting this declaration into practice, the adoption of a well-being lens7 for agriculture requires a broader perspective on policy making, which calls for strengthening other dimensions of the food system beyond market and income criteria. A comprehensive, multi-criteria approach emphasises broader priorities, such as providing access to a healthy diet, ensuring a healthy and safe environment, mitigating the risks of climate change and sustainably managing natural resources (land, water, soils and genetic diversity).
Such an approach can prevent inefficient policies, enabling policy makers to identify measures that enhance synergies, anticipate trade-offs and ultimately facilitate two-way alignment between climate change mitigation and other SDGs (discussed in Chapter 1). In this perspective, policy makers can take decisions in full knowledge of the associated difficulties, which they may choose to curb or compensate. For instance, a particular focus on workers’ protection and training might facilitate the sector’s transition.
The necessary change in perspective entails taking a food system approach that analyses change in multiple levers, including the supply (agriculture) and demand sides (final consumption). The system should respect natural cycles (water, nitrate) on the production side, as well as emphasise access to a healthy diet for all.
To create policies that lead to a sustainable food system, performance measurement needs to evolve towards full-cost accounting, i.e. a comprehensive set of indicators reflecting the impact of the food system on multiple well-being dimensions, in line with policy priorities discussed. Such indicators would help policy makers establish targets and track progress in delivering such priorities. They can also contribute to setting criteria for policy decisions, and facilitate the necessary co-ordination of actions between sectors and between countries.
Section 6.2 covers climate change mitigation policies for agriculture. It discusses how the change in perspective might help redefine the relative priorities and trade-offs between different goals. It emphasises policy measures that may have only a small – or even negative – impact on total production, but could be highly beneficial in terms of promoting healthier diets and a healthier environment. It also discusses how different policy-design, evaluation and compensatory actions can vitally enhance synergies and help minimise potential negative effects when implementing the climate policies, potentially enhancing public acceptability.
6.2. Agriculture through a well-being lens
Agricultural production can generate both positive and negative effects (Table 6.1). The provision of food is the essential function of agricultural production and is a precondition for human well-being. At the same time, agricultural production may have a negative impact on many dimensions of present and future well-being, including the sustainability of agriculture and the future availability of food. For instance, the intensive use of fertilisers or pesticides has harmful consequences on the environment, including water and soil quality, and biodiversity (OECD, 2018[15]). Agricultural production also generates direct GHG emissions (Smith et al., 2014[16]). The extension of agricultural land causes carbon releases due to deforestation or the destruction of other types of ecosystems (like peatland or savannah).
As the environment provides ecosystem services, agriculture in turn can provide agro-environmental services to society, such as flood risk mitigation and resilience to droughts (by improving agricultural soil quality)8, carbon sequestration (e.g. by improving soil quality, since good soil contains more organic matter, or putting additional plants like trees or hedges on agriculture land), water cycling and habitat for a diversity of species. Table 6.1 presents a number of positive and negative impacts from agriculture.
The impacts of agricultural production can also create a feedback effect on the medium- to long-term sustainability of agriculture, affecting future food availability and the sector’s capacity to provide sustainable bioenergies that could be used to generate negative CO2 emissions. Agriculture depends on biodiversity9 for a multiplicity of supporting services, such as pest and disease control; soil fertility and animal pollination; provisioning services, such as food, fibre, medicines or freshwater; and regulating services, such as soil and air quality, climate regulation or pollination. The sector is also strongly impacted by climate change (see Box 6.1). For example, a global warming of 1.5°C will probably decrease yields locally in tropical regions and is also likely to affect food nutritional quality, which may significantly impact on food security and the viability of livestock rearing in some regions; in a 2°C warming scenario, yields in temperate regions would also be decreased (IPCC, 2018[5]).
Table 6.1. Selected impacts of agricultural production and the food system on well-being
|
Positive effects |
Negative effects |
---|---|---|
Climate |
Carbon sequestration in agricultural soil Potential contributions of bioenergy to decarbonisation in other sectors |
GHG emissions, coming mainly from livestock and fertilisers (see Box 6.1) Land-use change related to agriculture leads to a loss of carbon sink |
Health |
Food security Nutritious food Genetic |
Occupational hazard for farmers, owing to their exposure to pesticides and strenuous work Risk of polluted food Spread of bacteria having developed resistance to antimicrobials and antibiotics used increasingly on intensive livestock farms Zoonotic diseases, exacerbated by intensive livestock production Unhealthy dietary patterns resulting in increasing rates of overweight and obesity, diets with insufficient micronutrients |
Ecosystems |
Ecosystem restoration in the agricultural system |
Loss of ecosystem services due to soil degradation: agriculture damages soils: i) physically (soil erosion due to wind exposure, compaction due to tillage and heavy machinery); ii) chemically (acidification due to excessive application of ammonium-based fertilisers and pesticide contamination in soils); and iii) biologically (loss of soil organic matter and fauna) (FAO, 2015[17]) Habitat loss due to deforestation: agriculturally driven habitat loss is a factor in the declines of a vast majority of the threatened mammal and bird species |
Water |
Flood risk mitigation in the agricultural system Groundwater recharge |
Water pollution (phosphorous and nitrate water pollution from chemical fertiliser use) Groundwater depletion from intensive irrigation |
Air |
|
Atmospheric pollution due to emissions of reactive nitrogen (ammonia, nitrogen oxides and PM 2.5) from agricultural land and biomass burning; pesticide pollution |
Socio-economic and cultural dimension |
Landscape structure Increased income for actors of the food system (wages, profits and rents, taxes) Tourism and leisure |
Box 6.1. Climate and the food system
As show in Figure 6.1, agriculture, forestry and other land use accounts for as much as 25% of global anthropogenic GHG emissions ( (Vermeulen, Campbell and Ingram, 2012[18]); (Smith et al., 2014[2])). Of this total, emissions related to land-use change account for 7-14% of global anthropogenic GHG emissions (or 36% of food system-related emissions), mainly from carbon releases due to deforestation or the conversion of peatlands into agricultural land.
Direct emissions account for 10-12% of global anthropogenic GHGs (or 46% of food system-related emissions) (Lankoski, Ignaciuk and Jésus, 2018[19]). These include N2O emissions from soils, fertilisers, and manure and urine from animals, and methane production from ruminant animals and paddy rice cultivation (Herrero et al., 2013[20]).
Figure 6.1. GHG emissions from food systems

Note: Pre-production includes fertiliser manufacture, energy use in animal-feed production and pesticide production. Post-production includes primary and secondary processing, storage, packaging, transport, refrigeration, retail activities, catering and domestic food management, and waste disposal. The midpoint of the range was used whenever a range of emissions was provided in (Vermeulen, Campbell and Ingram, 2012[18]).
Source: Authors, based on (Smith et al., 2014[2]) and (Vermeulen, Campbell and Ingram, 2012[18]).
Feeding a rapidly growing population has been achieved in part through the increased use of synthetic fertilisers. This has amplified the global nitrogen cycle (OECD, 2018[15]), with resulting environmental problems at different spatial and temporal scales. In particular, increased emissions of N2O, a powerful and relatively long-lived GHG, have caused a small but significant climate forcing between 1750 and 2011 (about 0.17 Watt per meter square [Wm-2,] compared to an estimated 1.68 Wm-2 for carbon dioxide ([O2]) (see (IPCC, 2013[21])). Methane (CH4) emissions have also increased rapidly owing to the growing number of ruminants, but also to oil and rice cultivation emissions. This powerful, but short-lived GHG also increases in tropospheric ozone levels, threatening human health and damaging ecosystems.
The lifetime of different GHGs determines their global warming potential (GWP) and hence the appropriate climate mitigation strategy. CH4 is a short-lived gas that remains in the atmosphere for 12 years (Pierrehumbert, 2014[22]); its GWP over the course of a century is 28 times higher than that of CO2 (Myhre et al., 2013[23]). N2O, on the other hand, remains in the atmosphere for 114 years, and its GWP is 265 times superior to that of CO2. Consequently, the reduction of N2O emissions is a priority for long-term mitigation strategies, compared to the reduction of methane emissions, which will have quicker but more limited effect over the longer term. In other words, a one-off reduction of a very long-lived GHG like CO2 is equivalent to a permanent reduction of the emissions rate of a short-lived GHG like methane.
Animal farming is responsible for the lion’s share of direct agricultural emissions (Blandford and Hassapoyannes, 2018[24]), with ruminants accounting for more than 80% of total livestock emissions (Herrero et al., 2013[20]). In addition to direct emissions, livestock farming and the crops produced to feed livestock also contribute to deforestation. Post-production emissions arise from the food processing and retail sectors relying increasingly on abundant synthetic packaging (Alpro and Murphy-Bokern, 2010[25]), and from the “food miles” racked up to deliver the highly processed and unseasonal products to which consumers have become accustomed (Schnell, 2013[26]).
Capturing GHGs in biomass, notably through sustainable bioenergy production, is an important potential mitigation option for the future, particularly to achieve stringent mitigation targets such as 1.5°C (IPCC, 2018[5]). According to the IPCC, the easier scenarios for limiting global warming to a 1.5°C increase by 2100 include net negative emissions in the second half of the century, which means that more carbon will be removed from the atmosphere than emitted. Several options exist for removing carbon from the atmosphere. The more easily available and plausible options involve forestry and agriculture. The feasibility and potentially negative consequences of other options – including afforestation and reforestation, bioenergy with carbon capture and storage (BECCS), and changed agricultural practices (e.g. biochar, soil carbon sequestration) – are uncertain. According to (Smith et al., 2016[27]), BECCS is the plausible option with the highest storage potential, while bioenergies have the advantage of reducing emissions in other sectors by providing a lower-emitting fuel.
As the question arises of ensuring a food system capable of feeding the world population (SDG 2), as well as contributing to wider well-being and SDGs, a well-being approach encompassing several priorities integrates the numerous well-being dimensions at stake. This perspective is consistent with the definition of food security set at the World Food Summit in 1996: “Food security exists when all people, at all times, have physical and economic access to sufficient, safe and nutritious food that meets their dietary needs and food preferences for an active and healthy life”.
An analysis of agriculture policies would integrate the following priorities: ensuring food security and contributing to healthy diets, limiting climate change, maintaining a healthy and safe environment, and ensuring the sustainable management of natural resources. Table 6.3 presents the links between these priorities and the SDGs, as well as with the domains and dimensions of well-being set by the OECD Framework for Measuring Well-being and Progress (henceforth the OECD well-being framework).
A comprehensive view of the food system, integrating all of its dimensions and actors, is necessary to achieve these objectives in an efficient way. Taking a broader perspective not only leads to a realignment of objectives, but also encourages an integrated vision of the whole system that goes beyond the agriculture sector and encompasses transformation, distribution and consumption. Figure 6.2 provides a comprehensive view of the food system, the different actors and the four capital stocks at stake, underlining the complexity of the relationships and the need for a global approach.10
Figure 6.2. Capital stocks and value flows in eco-agri-food systems
Policies and measures focusing on a single objective or only on the agriculture sector might overlook important trade-offs – e.g. between climate mitigation policies and food security – and miss opportunities for synergies. (Fujimori et al., 2019[29]) highlights that “carelessly designed climate mitigation policies” would increase the number of people at risk of hunger by 160 million by 2050 if nothing is done to prevent it.11 Similarly, policies aiming for food-price competitiveness may appear inefficient if they result in cutting production costs at the expense of the environment or human health. Furthermore, other sectors, such as the health system or the water system, pay for the damage wrought by agriculture on food and the environment. Specifically, the food provided by the current system may have unwanted health outcomes that are costly for the population and are not accounted for (see Box 6.2).
Current production is also focused on energy-rich staples (like wheat or corn), at the expense of legumes and a broad range of minor crops with higher nutritional value (Hawkes, 2006[30]) (DeFries et al., 2015[31]). Furthermore, systems with less breeding efforts focused on productivity have resulted in increased nutritional density (Barański et al., 2014[32]) (AFSSA, 2003[33]).Two billion people world-wide have diets with insufficient micronutrients, leading to a range of health problems throughout their lifespans, such as risks of stunting, reduced immune function (and resulting risks of infection), loss of productivity, reduced mental capacity and chronic disease (Bailey, West Jr. and Black, 2015[34]); (Schaible and Kaufmann, 2007[35]); (IFPRI, 2016[36]). The Health Plus Programme copes with this issue by introducing crops that are naturally higher in nutrients in emerging and developing countries. Box 6.2 presents some studies that have examined the hidden costs of the current food system providing large quantities of food at low prices.
Box 6.2. The hidden costs of the food system
While food prices have decreased relative to income and become more affordable (Dorward, 2013[37]), the food system induces costs to human health and the environment that are not included in food prices, and whose weight is instead spread across society. Even though no single approach has allowed drawing an estimation of the total hidden costs of food system, there exists a wide literature that estimates them. The below examples of such estimations show the amounts at stake for countries and hence, the relevance of investing in policies:
The use of chemicals in agriculture involves costs borne by health systems:
A study estimated annual endocrine disrupting chemicals-related health costs incurred in the United States through pesticide exposure alone at USD 42 billion (US dollars) (Attina et al., 2016[38]). In the European Union, organophosphate pesticides were estimated to produce the costliest outcomes in terms of exposure to endocrine disrupting chemicals, amounting to USD 121 billion per year.
In the United States, antimicrobial-resistant infections have been linked to 8 million additional hospital days and health costs of USD 20-34 billion per year (Paulson et al., 2015[39]).
Malnutrition (which encompasses both under- and over-consumption) affects an estimated 2 billion people, putting a heavy price on society not only in terms of health expenses, but also in terms of cognitive-skill loss.
IFPRI (2016[36]) estimates this cost at USD 3.5 trillion globally, i.e. 11% of world GDP.
The WHO highlights that stunting in early childhood not only affects future health (mortality and morbidity), but also cognitive development (WHO, 2017[40]).
In terms of unhealthy diets, a report by the McKinsey Global Institute concluded that, based on “disability-adjusted life years” data, obesity has an economic impact of about USD 2 trillion, or 2.8% of global GDP (McKinsey Global Institute, 2014[41]).
The WHO estimated the direct costs of diabetes alone, often resulting from obesity, at more than USD 827 billion per year globally (WHO, 2016[42]). In the United States, the annual cost of diabetes in 2017 was estimated at USD 327 billion, including USD 237 billion in medical costs and USD 90 billion in reduced productivity (American Diabetes Association, 2018[43]).
Agricultural practices may threaten the ecosystem services from which they benefit and hence, future profitability. A major illustration is the drastic reduction in insect populations worldwide, which is mainly due to habitat loss and land-use change to intensive agriculture, as well as urban sprawl and pollution, mainly from agriculture inputs (Sánchez-Bayo and Wyckhuys, 2019[44]).
The global economic value of pollinators to the agricultural sector has been estimated at USD 235-577 billion per year (Potts et al., 2016[45]).
Similarly, (Sandhu et al., 2015[46]) estimate that the global value of biological pest control and nitrogen mineralisation due to ecosystem services for targeted crops (peas, beans, barley and wheat) amounts to USD 34 billion per year.
Using literature (Costanza et al., 2014[47]), the latest Group of Seven (G7) report on biodiversity estimates that the total cost of ecosystem services (climate regulation, pollination and water regulation) amounts to USD 125-140 trillion per year (OECD, 2019[48]).
A shift towards a healthier and more sustainable diet is necessary address the challenges outlined above, creating important synergies between climate and wider well-being goals. Policies encouraging less emission-intensive food baskets may have a significant mitigation potential ( (Poore and Nemecek, 2018[49]); (Bajželj et al., 2014[50]); (Wollenberg et al., 2016[51])) while also benefitting health. Diets in many countries are not in line with the nutritional recommendations of the WHO: meat and sugar consumption exceeds dietary guidelines, while fruits and vegetables consumption is below the recommended intake (OECD forthcoming, 2019[52]). For instance, 70% of adults over 18 were overweight12 in the United States in 2016, 67% in the United Kingdom, 64% in Mexico, 61% in Romania and 51% in Guatemala. In Singapore, according to (Epidemiology & Disease Control Division, Ministry of Health and Institute for Health Metrics and Evaluation., 2019[53]), dietary risks were among the leading risk factors affecting health. In Europe, the Institut du Développement Durable et des Relations Internationales (IDDRI), a French think tank, estimates that shifting towards diets in line with WHO nutritional recommendations would result in a 40% reduction of GHG emissions from agriculture and better health outcomes (Poux and Aubert, 2018[54]). The EAT-Lancet Commission on Food Planet and Health (EAT-Lancet Commission, 2018[55]) recently reported that reaching a healthy diet globally would require dividing the global consumption of red meat (beef, lamb and pork) by nearly three (more than six in North America).13
A dietary shift towards more plant-based proteins can contribute significantly to climate objectives, as the production of plant-based proteins generally emits smaller amounts of GHGs14 than that of animal-based proteins – of which beef emits the most. (Popp, Lotze-Campen and Bodirsky, 2010[56]) highlight that, if the current dietary trends and population growth were to continue, non-CO2 emissions (methane and N2O) would triple by 2055. By contrast, scenarios that would limit climate change to 1.5°C by the end of the century all include a rapid drop of methane emissions before 2025, and most include a decrease in N2O (IPCC, 2018[5]). This involves a slump in the numbers of ruminant livestock, as most methane emissions come from enteric fermentation. Such a change in dietary patterns would mitigate climate change through two distinct channels: first, it would reduce direct emissions from animals; second, it would ease pressure on land use, since a large proportion of crops are grown to feed livestock. In its report on climate change and land (Arneth et al., 2019[3]), the IPCC estimates that changing diets has a major GHG reduction potential (from 3 GtCO2eq per year for a Mediterranean diet to 8 GtCO2eq per year for a vegan diet).
Taking a broader perspective not only leads to a realignment of objectives, but also encourages an integrated vision of the whole system that goes beyond the agriculture sector and encompasses transformation, distribution and consumption.
Analysing the food system as a whole highlights synergies between actors and objectives. A good way to ensure that farmers implement environmental practices over the long term is to ensure that these practices are economically viable. This implies mobilising the whole value chain and reorganising markets to create new opportunities. First, all actors would need to promote the provision of healthier and more sustainable food (e.g. through the development of labels by farmers and agro-industrial companies, and highlighting these labels on packaging and in stores’ shelf placement). Second, the new patterns of production would need to be accompanied by a reorganisation of key actors. In particular, practices striving for a more sustainable agriculture, notably due to higher crop rotation or legumes used as intermediate crops (see Part 2 of this report for details on sustainable agricultural practices), would need to be based on a higher level of diversity in the supply of grains and plants. This new diversity has to be managed and valued by the whole value chain, from the harvest, through transformation by the agro-industry, to the final retailers. The worldwide development of organic farming and food is a good example of how accumulated changes at every link of the food chain lead to the development of a parallel market chain. As consumer demand grows for healthier and more sustainable food, farmers and cooperatives, supported by public subsidies, respond through advertising, new labelling schemes, a greater variety of products, and so on. Part 2 of this report provides more details on organic agricultural practices.
A holistic approach to the food system aiming for a sustainable use of resources advocates a greater integration of waste management at every stage of the food chain, using different levers. According to the World Resources Institute (WRI, 2018[57]), based on Food and Agriculture Organization of the United Nations (FAO) estimates, nearly one-third of the global food production in terms of weight, and one-quarter in terms of energy content, is not consumed, wasting the resources used to produce it. Reducing waste at every level by using sustainable practices from production to final consumption is the optimal solution, as it reduces the need for food production and releases the pressure for higher yields in order to meet food security goals. It is a lever to reduce environmental pressures from the overexploitation of resources. Figure 6.3 shows the distribution of lost food and wasted food, highlighting that the bulk of waste in developed countries comes from consumption (61% in North America and Oceania, 46% in industrialised Asia and 52% in Europe), but there are also significant losses during production, handling and storage (23% in North America and Oceania, 40% in industrialised Asia and 35% in Europe). In developing countries, the main problems concern production, handling and storage. When the waste cannot be avoided, it may be re-used by reintegrating it into the natural cycles of nutrients. Synthetic fertiliser can be replaced by organic waste from municipal waste or crop residues, which has the potential to mitigate GHG emissions if managed properly. Finally, as these wastes have an organic component, they can be used to create energy, with proper infrastructures connecting the power plants to the (gas or electricity) energy grid (see examples in Chapter 4 on the residential sector). This has a strong mitigation potential, since waste may appear as a substitute not only for fossil fuels, but also for biofuels, which are responsible for deforestation and land pressure.
Figure 6.3. Food loss and waste in developing and developed countries

Note: % underneath x-axis is the share of total food available that is lost or wasted (in terms of weight per year).
Source: WRI analysis, based on FAO.
Numerous questions remain on how governments structured around functional or sectoral ministries, often with different budgets and sometimes contradictory agendas, can apply such a holistic approach. Shared policy goals would lead to more co-ordination between institutions and ministries. Most of the time, agriculture policies are supported by one ministry specialised in the agriculture sector and decisions are taken accordingly, often to support farm incomes and production. Taking a well-being approach would require other ministries (e.g. environment and health ministries) and local institutions to demonstrate a greater degree of involvement in decision-making. Showing the benefits of such a holistic approach, Table 6.2 synthesises the main synergies and trade-offs in the food system highlighted by a well-being lens integrating multi-dimensional objectives and a systemic view.
Table 6.2. Potential two-way alignment benefits from applying the well-being lens to the agriculture sector
Other policy priority |
Contributing to limiting climate change |
|
---|---|---|
Generating synergies |
Avoiding/reducing trade-offs |
|
Food security and the provision of a healthy diet |
Intensifying production by hectare can prevent conversion of unmanaged land to agriculture and hence maintain carbon sinks. Healthier diets by reducing intake of meat and animal products can result in significant GHG emission reductions. Growing leguminous crops has the potential to contribute to a stable climate (since they can be used to fertilise soil and therefore limit the use of chemical fertilisers), resulting in more diversified diets and higher nutrition. Ecological practices can provide job opportunities while reducing GHG emissions and improving on-farm carbon sequestration. |
Climate policies may increase food prices, leading to affordability and accessibility issues, especially for low-income households. In particular, there might be a conflict for land use between bioenergies and food that can make food prices higher and more dependent of energy prices. This trade-off between mitigation and food security might strongly hinder the deployment of bioenergies (Arneth et al., 2019[3]). Sustainable practices are more labour-intensive and may therefore increase food prices. Policies encouraging healthier and more climate-friendly diets can curb the detrimental effects on households’ purchasing power, e.g. through reductions in the amount of animal protein in diets in favour of plant proteins (legumes, oil seeds), which are much cheaper. They would also reduce the pressure put on land, since livestock occupies lot of land, both for grazing and growing crops to feed the animals. Employment in agriculture may be affected by any reshaping of the food system. For instance, jobs in livestock could be destroyed if meat consumption decreases. According to (Jean Chateau, 2018[58]), farm workers would among the most impacted by the implementation of a carbon tax. However, climate mitigation practices in agriculture are more labour-intensive than conventional practices, and thus have the potential to offset any negative impacts in the livestock-labour market. Training could help facilitate this transition of jobs and workers. |
Maintain a safe and healthy environment |
Reducing fertiliser use could result in reduced nutrient run-off and water pollution, leading to healthier aquatic ecosystems. It also reduces ammonia volatilisation, which participates in the formation of particulate matters and therefore improves air quality. |
Tillage is a practice that helps remove the weeds and alleviates topsoil compaction. While reducing tillage may contribute to GHG mitigation, it may also increase the need for pesticides. Conservation tillage, which consists in letting the previous year’s crop residue on fields before and after planting, reduces soil erosion, helps to reduce the impact of food production on soil structure and avoid run-off. It can be considered as an alternative in many cases. |
Sustainable management of natural resources |
Forest conservation efforts can maintain carbon sinks and reduce GHG emissions while benefitting other ecosystem services Restoration of agriculture land and agroforestry can increase carbon storage while providing habitat for on-farm biodiversity. |
|
6.3. Indicators for monitoring agriculture’s contribution to well-being
This section presents and discusses different indicators that can contribute to steering food systems towards sustainability. These indicators could be used together, as criteria to guide policy decisions, but also as a way to track the performance of a given food system and the effectiveness of policies in delivering the goals defined in Section 6.2: food security defined as access to a healthy diet, the limitation of climate change, a healthy and safe environment, and the sustainable management of natural resources.
The food system is an essential component of the SDGs of the 2030 Agenda for Sustainable Development and constitutes SDG 2, “zero hunger”. That goal is divided into five different sub-targets:
end hunger and ensure access by all people … to safe, nutritious and sufficient food all year-round
end all forms of malnutrition … and address the nutritional needs of adolescent girls, pregnant and lactating women and older persons
double the agricultural productivity and incomes of small-scale food producers
ensure sustainable food production systems and implement resilient agricultural practices
maintain the genetic diversity of seeds, cultivated plants and farmed and domesticated animals and their related wild species … and promote access to and fair and equitable sharing of benefits arising from the utilisation of genetic resources.
As highlighted in Chapter 1, the SDG targets and the well-being priorities defined in section 6.2 overlap substantially, since both approaches aim to be inclusive and place economic goals on the same level of priority as others. Sustainability and access to healthy diets are central to both approaches. The global indicator framework developed for the SDGs – especially SDG 2 – provides a useful set of indicators to monitor well-being. Other SDGs relating to the food system provide some indicators in line with a well-being analysis of the food system: SDG 3 (good health and well-being), SDG 6 (clean water and sanitation), SDG 8 (decent work and economic growth), SDG 14 (life below water), SDG 9 (industry, innovation and infrastructure), SDG 11 (sustainable cities and communities) and SDG 12 (responsible production and consumption). However, they often require complementary information: even though agriculture may affect these SDG indicators, they do not explicitly mention its specific impact. Table 6.3 summarises the links between these policy priorities and the SDGs, as well as with different dimensions and domains of the OECD well-being framework. Sustainability and access to healthy diets are central to both approaches. The global indicator framework developed for the SDGs, especially SDG 2, provides a useful set of indicators to monitor well-being.
The summary tables in each section links a number of useful indicators to the SDGs and the OECD well-being framework. These tables are not exhaustive, but aim to open discussions. Most of the indicators presented have been developed nationally, or at international organisations like the OECD. Although the OECD well-being framework provides information on dimensions (air quality or water quality) impacted by agriculture, it does not include any information on the specific impact of agriculture and hence cannot be used as a unique criterion. The Biodiversity Indicator Partnership (BIP) listed 64 indicators to track the progress towards the Aichi Biodiversity Targets,15 and more particularly towards a sustainable agriculture, aquaculture and forestry (Aichi Target 7) and the limitation of pollutions detrimental to ecosystem functions and biodiversity (Aichi Target 8). The European Union developed its own set of indicators to monitor its agriculture policies. Current discussions on the next EU common agricultural policy for 2020-24 include nine objectives16 and quantitative targets EU Member States must reach through their own national strategic plans. Thus, indicators17 measuring the targets underlie the EU agricultural policy in order to assess its efficiency. The United States Department of Agriculture (USDA) has also developed a set of indicators to track the environmental impacts of agriculture (Hellerstein, Vilorio and Ribaudo, 2019[59]).
The summary tables also include many of the agri-environmental indicators developed by the OECD to monitor the environmental performances of its (then 34) member countries (OECD, 2013[60]). The agri-environmental indicators have the potential to compare the environmental performance of agriculture in these countries and monitor progress over time since the 1990s. They cover many dimensions of the environmental impacts of agriculture, such as land cover, nutrient balance, pesticide use, water abstraction and water quality, GHG emissions and soil quality.
Table 6.3. Policy priorities for the agriculture sector and their links to the SDGs and the OECD well-being framework
Policy priority |
Sub-objectives |
SDG goal and target |
OECD Well-being domain |
OECD Well-beingdimension |
---|---|---|---|---|
Ensure food security and healthy diets |
Food production. |
2. End hunger, achieve food security and improved nutrition and promote sustainable agriculture. |
The well-being framework does not include any dimension on food availability. |
The well-being framework does not include any dimension on food availability. |
Food affordability and accessibility. |
2. End hunger, achieve food security and improved nutrition and promote sustainable agriculture. |
The well-being framework does not include any dimension on food availability. |
The well-being framework does not include any dimension on food availability. |
|
Healthy diets. |
2.2. By 2030, end all forms of malnutrition. |
Future well-being: resources. Current well-being: quality of life. |
Human capital Health status |
|
Farmers' living conditions and skills. |
2.3. By 2030, double the agricultural productivity and incomes of small-scale food producers. |
Current well-being: material conditions. |
Income and wealth Job and earnings |
|
Limit climate change |
Reduce GHG emissions in agriculture. |
13. Take urgent action to combat climate change and its impact. |
The well-being framework does not include any dimension on food availability. |
Resources – future |
Carbon sequestration. |
The well-being framework does not include any dimension on food availability. |
Resources – future |
||
Bioenergy contribution to mitigation in other sectors. |
The well-being framework does not include any dimension on food availability. |
The well-being framework does not include any dimension on food availability |
||
Maintain healthy and safe environment by minimising the pollution of air, water and soil from agriculture |
Air quality. |
3.9. By 2030, substantially reduce the number of deaths and illnesses from hazardous chemicals and air, water and soil pollution and contamination. |
Current well-being: quality of life. |
Environmental quality. |
Water quality. |
3.9. By 2030, substantially reduce the number of deaths and illnesses from hazardous chemicals and air, water and soil pollution and contamination. |
Current well-being: quality of life. |
Environmental quality. |
|
Reduced degradation of soils. |
Current well-being: quality of life. Future well-being: resources. |
Environmental quality. |
||
Maintenance of biodiversity and ecosystem services. |
Future well-being: resources. |
Natural capital. |
||
Sustainable management of the planet’s natural resources – land, water and virgin raw materials |
Circular economy. |
12. Ensure sustainable consumption and production patterns. |
Future well-being: resources. |
Natural capital. |
Efficient use of water resources. |
Future well-being: resources. |
Natural capital. |
||
Efficient material use. |
12. Ensure sustainable consumption and production patterns. |
Future well-being: resources. |
Natural capital. |
6.3.1. Ensuring food security and healthy diets
Four types of indicators – total food production, food affordability and accessibility, dietary health impacts and farmers’ living conditions – track the food system’s capacity to provide food security and healthy diets. All these dimensions are necessary to reach long-term food security and Table 6.4 proposes a set of indicators to track them. Organisations tracking agricultural performance have long mainstreamed indicators on food production and single-factor agricultural productivity (e.g. yields), often driving policy decisions regarding agriculture While the SDG indicator framework includes the volume of production per labour unit of farming or pastoral enterprise size, other institutions focus on indicators of production (value added of agriculture in the OECD Green Growth Dashboard) or the factor productivity. Altogether, these indicators are complementary and provide comprehensive information on the agriculture sector’s capacity to produce efficiently, in terms of productive marketed factors (land, labour, intermediate inputs and capital). However, these indicators are not sufficient to estimate the overall efficiency of agricultural production, since they overlook unpriced factors (e.g. biodiversity) and potential negative externalities (water pollution), which leads to overestimating factor productivity.18 To answer this issue, research by the International Platform on Biodiversity and Ecosystem Services (IPBES) or TEEB to measure unpriced ecosystem services may help integrate them in sectoral accounts and the measure of factor productivity. For instance, IPBES assessed that pollination service created a value estimated at USD 235-577 billion in global agriculture in 2015 (Potts et al., 2016[45]). As such, it should be included as a factor of production in the calculation of the sector’s total factor productivity, which would automatically decrease.
Indicators from the OECD well-being framework or the SDG framework provide little information on food affordability. The existing indicators in the SDG framework and other international organisations focus on the variability of food prices. Although a high volatility of food prices may strongly harm consumer well-being, information on the general level of prices is more important, particularly for low-income or otherwise vulnerable groups. The FAO has introduced the concept of food insecurity, which is used by several countries to track policies. The United States, for instance, measures food insecurity through answers to a representative household survey, capturing the concept’s different dimensions (i.e. availability, accessibility, utilisation and stability). However, applying a well-being approach entails clearly assessing and disentangling different factors, particularly the economic barriers to a healthy and adequate diet. Understanding these barriers is crucial to analysing the potential effects of environmental and climate mitigation measures on households’ capacity to pay for a healthy diet. An indicator comparing households’ food expenses to income would be an accurate measure in this respect. Going further, the price of a healthy food basket for households (that is nutritionally adequate as defined, for instance, by (Willett et al., 2019[61])) relative to income would add precious information on the affordability of healthy food.19 The availability of this indicator by decile of income would also provide useful information concerning the distributional impact of policies.
The SDG framework includes useful indicators on the prevalence of undernourishment and the populations that suffer from food shortages. However, proper and precise indicators on other kinds of malnutrition are hard to find. This is because a healthy diet is defined as a balance between nutrients for a given individual. As a result, aggregate data on nutrient inputs are insufficient, since they do not tackle inequality issues: the total input can be enough to feed the population, but may hide strong inequalities. Besides, many of the diseases (e.g. obesity, cardiovascular problems, diabetes) linked to unbalanced diets are determined by several factors (people’s daily activity, genetic propensity), and the actual role of food is hard to determine. Nevertheless, the indicators on the prevalence of these diseases appear as imperfect but useful proxies of malnutrition issues, as they can stand as a proxy to track the adoption of healthier diets, and can be completed by more accurate estimates of the role played by food and diets. Tracking general health indicators, like life expectancy, may also provide relevant information on how agriculture may affect different well-being dimensions.
Table 6.4. Summary table: Indicators for monitoring progress in ensuring food security and healthy diets and links to SDGs and OECD well-being framework
Policy priority |
Sub-objectives |
Proposed indicators |
SDG indicators |
OECD Well-being domain/dimension |
OECD Well-being indicators |
---|---|---|---|---|---|
Ensuring food security and healthy diets |
Food production |
Value added in agriculture.2 Total factor productivity in agriculture.4 |
Volume of production per labour unit by classes of farming/pastoral enterprise size. |
Framework does not have related indicators. |
Framework does not have related indicators. |
Food affordability and accessibility |
Commodity price variability.4 Consumer price of food products (index).4 Food security index (FAO). Share of food expenses or cost of a healthy food basket for households generally and for poor-income households specifically. |
Indicator of food-price anomalies. |
Future well-being: resources. Human capital. Health status. Current well-being: quality of life. |
Framework does not have related indicators. |
|
Healthy diets |
Extent to which food education is mainstreamed in: national education policies curricula teacher education; and student assessment |
Prevalence of undernourishment. Prevalence of moderate or severe food insecurity in the population. Prevalence of stunting among children under 5 years of age. Prevalence of malnutrition among children under 5 years of age, by type (wasting and overweight). Mortality rate attributed to cardiovascular disease, cancer, diabetes or chronic respiratory disease. |
Future well-being: resources. Current well-being: quality of life. Human capital. Health status. |
Obesity prevalence. Life expectancy. |
|
Farmers' living conditions and skills |
Agricultural entrepreneurial income.4 Rural employment rate.4 Degree of rural poverty.4 Rural GDP per capita.4 Workers’ turnover (proportion of workers entering and leaving the profession). Proportion and number of children engaged in child labour in agriculture. Life expectancy for agricultural workers. Specific training in agricultural schools on sustainable practices. |
Average income of small-scale food producers, by sex and indigenous status. |
Current well-being: material conditions. Income and wealth. Job and earnings. |
Household income. Employment Earnings. Job strain. |
1. This indicator is included in the OECD well-being framework.
2. This indicator is included in the OECD Green Growth Dashboard.
3. This indicator was developed by OECD as an agri-environmental indicator.
4. This indicator was developed to monitor the EU common agricultural policy.
5. This indicator was developed by the USDA.
6. This indicator was listed by the BIP to track progress towards the Aichi Targets.
Climate change mitigation in the food system also requires action on the consumer side. Informing people on the health, environmental and climate-related impacts of food is therefore crucial. Public education seems like an efficient lever. Hence, this report proposes monitoring demand policies through an indicator on food education.
Farmers’ living conditions determine the long-term viability of agriculture and are therefore key determinants for food security. Ensuring a sustainable food production requires maintaining and developing a skilled workforce. For this reason, the SDG framework include farmers’ income as an indicator for SDG 2 (“zero hunger”). However, information on incomes should be completed by information on the many other dimensions of working conditions, including the agriculture sector’s compliance sector with international labour rights, farm workers’ life expectancy relative to the rest of the population and occupational risks.
Finally, implementing a sustainable agriculture calls for more technical training for farmers. Sustainable practices often require a sound knowledge of ecosystems and agronomy, as precision agriculture relies on optimising and reducing inputs and takes into account environmental factors. Indicators on farmers’ training to estimate the sector’s ability to respond the sustainability challenges should therefore be developed and tracked.
6.3.2. Limiting climate change
The food system can contribute to climate change by emitting GHGs; by sequestering carbon in soils and in agricultural production (plants and animals); and by contributing to the mitigation of emissions from other sectors, through the sustainable production of bioenergy (see section 6.1). While the SDG framework does not feature an indicator on GHG emissions, such information can be easily found – even at the sectoral level – as other institutions (e.g. the FAO) have developed indicators on GHG emissions in agriculture enabling for international comparisons. Table 6.5 proposes a set of indicators to monitor agriculture’s contribution to climate change.
A well-being approach calls for developing an indicator on carbon footprints. Although carbon footprints are difficult to estimate because they require information on the whole food chain, they have the potential to provide useful information if determined by a sound methodology. One limitation of considering national carbon emissions is that this indicator focuses on the production side at the national level and hence do not account for the climate impact of imported food.
Information on carbon sequestration and land-use change caused by agriculture is hard to collect, since many factors are at play. The carbon sequestration capacity of agricultural soil depends on farming practices (e.g. agroforestry sequesters carbon) and initial soil quality, neither of which is reflected in existing indicators. Such an indicator is crucial for climate-policy makers as sequestration capacity should be estimated regularly, ideally at the parcel level.
The climate effect of the food system also arises from how the sector affects land use. Estimating the precise impact of agriculture on deforestation is not straightforward. The impact differs strongly among the different regions of the world, as urban sprawl also disturbs the distribution of land and can indirectly pressure forest areas by displacing agricultural land. As proof of this heterogeneity, almost all of the deforestation (-7 million hectares) between 2000 and 2010 occurred in tropical areas; by contrast, forested land increased in North America, Europe and Northeast Asia, while agricultural land decreased (FAO, 2016[62]). Consequently, an adequate analysis of the role of agriculture in the loss of natural land can be provided by using two complementary indicators, developed in the OECD Green Growth Dashboard: i) the share of land conversion from natural or semi-natural land to cropland; and ii) the share of land conversion from cropland to artificial lands. The analysis and comparison of the trends identified by both indicators could help identify the drivers of land conversion.
The production of bioenergies is crucial for climate stability. It needs to be monitored in a life-cycle assessment of both bionergies’ direct mitigation impact, and their likely conflict with food production or natural lands over land use. A first set of indicators could track the production of first-generation and advanced bioenergies through their contribution to the energy sector, i.e. the units of energy produced (as a total or as a share of the total energy produced). Another set of indicators could monitor land use for bioenergy as a total, as a share of total land use and as a share of agricultural land.20 The share of agricultural land would be particularly useful to assess land-use conflict with food, as the production of bioenergy does not necessarily correlate with its land use. Some bioenergies are made through intermediate crops, crop residuals (for second-generation biofuels) or the methanisation of manure, and therefore do not conflict directly with food production.
Table 6.5. Summary table: Indicators for monitoring progress in limiting climate change and links to SDGs and OECD well-being framework
Policy priority |
Sub-objectives |
Proposed indicators |
SDG indicators |
OECD Well-being domain/ dimension |
OECD Well-being indicators |
---|---|---|---|---|---|
Limiting climate change |
Reduce GHG emissions in agriculture |
Nitrogen use efficiency Energy use in agriculture3 CO2 emission per unit of value added in agriculture: methane emissions, N2Oemissions GHG emissions in agriculture:3 4 methane emissions, N2O emissions Food consumption carbon footprint |
Framework does not have related indicators. |
Future well-being: resources. Natural capital |
GHGs from domestic production CO2 emissions from domestic consumption |
Carbon sequestration |
Natural and semi-natural vegetated land, % total2 Cropland, % total2 Conversion from natural and semi-natural land to cropland, % since 1992 Ratio of agricultural land consumption rate to population growth rate Carbon sequestration per hectare of agriculture land |
Framework does not have related indicators. |
Future well-being: resources. Natural capital |
Forest area. |
|
Bioenergy contribution to mitigation in other sectors |
Bioenergy production (in GWh or as a total of produced energy and as a share of the total arable land) Biofuel production by type (bioethanol and biodiesel) and generation (first and second) and as a share of the total arable land in GWh |
Framework does not have related indicators. |
Future well-being: resources. Natural capital. |
Framework does not have related indicators. |
1. This indicator is included in the OECD well-being framework.
2. This indicator is included in the OECD Green Growth Dashboard.
3. This indicator was developed by OECD as an agri-environmental indicator.
4. This indicator was developed to monitor the EU common agricultural policy.
5. This indicator was developed by the USDA.
6. This indicator was listed by the BIP to track progress towards the Aichi Targets.
6.3.3. Maintaining a healthy and safe environment (air, water, soil and biodiversity)
A healthy and safe environment is provided by good air, water and soil, as well as high levels of biodiversity. All these dimensions are intrinsically related, since each is determined by the others (for instance, biodiversity cannot flourish without good air, water and soil quality, and reciprocally).Table 6.6 proposes a set of indicators to monitor them.
Collecting indicators on agriculture’s impacts on air and water quality is no easy task. First, determining the precise role of agriculture is complicated by the fact that many other factors, like industrial, household or transport pollutants, may affect air and water quality. Second, measuring air or water quality has many different dimensions, given the many potential pollutants (nitrate, phosphorous, sulphur, pesticides, etc). A comprehensive indicator should therefore include all these dimensions. For instance, the ecological quality of water basins defined in the European Commission’s water directive21 encompasses physiochemical and ecological criteria that were to be reached by 2015. The OECD provides indicators on the pollution of waters in agricultural areas, but does not cover agricultural pollutants in other areas. Developing a similar indicator on air quality would be useful, although it may impose high collection costs, given the precision of the information needed.
The SDG framework provides good information on soil quality by including an indicator on the share degraded land, but it is still under development and does not specify the type of degraded land (e.g. forest or agricultural land). Besides, many factors may cause the degradation, including the changing climate. The specific impact on agriculture can be estimated by using other existing indicators, such as the share of agricultural land with erosion risk or the share of organic matter in arable land. Neither the SDG nor the OECD well-being frameworks feature an indicator on the role played by certain agricultural practices on land erosion, owing to the difficulty to estimate this effect. As a proxy, the SDG framework proposes a soil carbon indicator, which is still under development. The USDA follows the evolution of the share of conservation tillage, which aims to reduce the negative impact of tillage on soil quality (see Table 6.2).
Tracking the biodiversity impacts of agriculture requires developing indicators on the state of biodiversity and the pressure exerted on it. Few existing indicators allow precise monitoring of impacts, although these impacts are generally well-known and documented. Biodiversity has many dimensions and several other environmental aspects can be tracked, like genetic diversity (within the same species), species diversity and habitat diversity (OECD, 2013[60]). The SDG framework indicators tackle genetic and species diversity, focusing on the diversity of plant and animal production in agriculture. The BIP suggests monitoring Aichi Target 7 (a sustainable agriculture, aquaculture and forestry) through the Living Planet Index for farmland (following population trends for vertebrates species) and the farmland birds index, which is also provided in the OECD agri-environmental indicators. The farmland birds index measures fluctuations in the populations of certain species of birds that depend on agricultural land for nesting or breeding. Farmland birds are considered good indicators of ecosystem health, as their population changes can reflect changes in the populations of other types of biodiversity (e.g. insects) that are more difficult to measure. This index is broadly used, and enables both time-based and international comparisons. However, it can only be considered as a proxy of the impact of agriculture on overall biodiversity or the biodiversity of agricultural areas. Among its shortcomings is that it does not cover the impact of agriculture on biodiversity in other areas. Moreover, the collection of this indicator relies on volunteer groups and is therefore subject to their availability (OECD, 2019[63]).
Table 6.6. Summary table: Indicators for monitoring progress in maintaining a healthy and safe environment and links to SDGs and OECD well-being framework
Policy priority |
Sub-objectives |
Proposed indicators |
SDG indicators |
OECD Well-being domain/ dimension |
OECD Well-being indicators |
---|---|---|---|---|---|
Maintaining a healthy and safe environment |
Air quality |
Mortality rate attributed to household and ambient air pollution. |
Current well-being: quality of life. Environmental quality |
Air quality |
|
Water quality |
|
Mortality rate attributed to unsafe water, unsafe sanitation and lack of hygiene. Index of coastal eutrophication and floating plastic debris density. |
Current well-being: quality of life. Environmental quality |
Water quality |
|
Reduced degradation of soils |
Proportion of land that is degraded over total land area. |
Future well-being: resources. Natural capital |
|||
Maintenance of biodiversity and ecosystem services |
Number of plant and animal genetic resources for food and agriculture secured in either medium- or long-term conservation facilities. Proportion of local breeds classified as being at risk, not at risk or at unknown level of risk of extinction. |
Future well-being: resources. Natural capital |
Threatened species |
1. This indicator is included in the OECD well-being framework.
2. This indicator is included in the OECD Green Growth Dashboard.
3. This indicator was developed by OECD as an agri-environmental indicator.
4. This indicator was developed to monitor the EU common agricultural policy.
5. This indicator was developed by the USDA.
6. This indicator was listed by the BIP to track progress towards the Aichi Targets.
6.3.4. A sustainable management of the planet’s natural resources
Sustainably managing resources requires using them efficiently and potentially moving towards a circular economy. The SDG indicators are insufficient to tackle both issues and should therefore be completed. Table 6.7 proposes a set of indicators.
The SDG framework has limited information on the degree of efficiency in the use of water or material resources in agriculture. The share of abstraction for agriculture from renewable sources seems crucial indicator on the sustainability of water use, but does not provide information on water-use efficiency, which is also a lever to reduce the impact of agriculture on water resources. More efficient irrigation practices limit irrigation water losses in watersheds. They also induce the growth of species that are less dependent on irrigation.
In this section, we propose indicators to measure water and material use efficiency as the quantity of resource consumed per added value in agriculture (consumption here corresponds to the share of water that does not go back into its environment once used). These indicators would inform policy makers on how policies and practices can improve resource efficiency, and – if international comparisons are provided – on the possibility of implementing more efficient practices elsewhere. However, a growing number of empirical studies show that resource-efficiency measures might have unwanted effects on the total availability of freshwater (Scheierling and Tréguer, 2018[64]), which should be carefully anticipated and monitored.
Monitoring how non-organic material is used in the overall food system should also be considered, since a large share of waste in the food system is attributable not to food, but to packaging. These wastes may create severe environmental damages and therefore should be reduced. Hence, the present paper proposes devising indicators that measure the material footprint of consumed food, defined as the weight of material needed for final food consumption.
The SDG framework provides information on the share of food loss and food waste, but fails to tackle the issue of circularity. Complementary indicators should provide information on how food waste is managed, i.e. the share of waste that is re-used in the agricultural sector (as fertiliser) or outside the sector (e.g. in the energy sector). Similarly, indicators on the uses of materials and water, and their efficiency, can be completed by indicators on how unused and wasted resources are treated.
Table 6.7. Summary table: Indicators for monitoring progress in a sustainable management of the planet’s natural resources and links to SDGs and OECD well-being framework
Policy priority |
Sub-objectives |
Proposed indicators |
SDG indicators |
OECD Well-being domain/dimension |
OECD Well-being indicators |
---|---|---|---|---|---|
A sustainable management of the planet’s natural resources |
Circular economy |
Share of recycled and managed food waste. |
Food loss index and food waste index. National recycling rate, tonnes of material recycled. |
Future well-being: resources. Natural capital. |
Framework does not have related indicators. |
Efficient use of water resources |
Change in water-use efficiency over time. Level of water stress: freshwater withdrawal as a proportion of available freshwater resources. |
Future well-being: resources. Natural capital. |
Freshwater abstraction. |
||
Efficient material use |
|
Material footprint, material footprint per capita, and material footprint per GDP. |
Future well-being: resources. Natural capital. |
Framework does not have related indicators. |
1. This indicator is included in the OECD well-being framework.
2. This indicator is included in the OECD Green Growth Dashboard.
3. This indicator was developed by OECD as an agri-environmental indicator.
4. This indicator was developed to monitor the EU common agricultural policy.
5. This indicator was developed by the USDA.
6. This indicator was listed by the BIP to track progress towards the Aichi Targets.
6.3.5. Comprehensive indicators
As the well-being approach aims to analyse policies with a comprehensive view, trans-sectoral indicators on the food sector can be a useful complement to the indicators detailed above. The SDG framework includes an indicator on the share of sustainable agricultural production, as well as several indicators on practices adopted by policy makers and firms to achieve more sustainability. However, there exists no proper, consensual definition of what constitutes sustainable agriculture, since the concept encompasses many different notions (environmental impact, viability, etc.) and is likely to vary depending on the prevailing resources and conditions (including climatic conditions). The FAO, in collaboration with the Global Strategy to improve agricultural and rural statistics, has been developing an indicator since 2015. The indicator is multidimensional and encompasses 11 sub-indicators and themes, covering productivity, profitability, risk, soil, water, fertiliser, pesticide, biodiversity, wages, food insecurity and land property rights. For example, GHG emissions can be reduced through policies promoting healthier diets. The need for social policies accompanying price increases due to the application of a GHG tax also becomes more evident when analysing social and economic impacts at the level of the food system. A broad and balanced set of policy measures, covering the entire food system, offers the greatest potential for climate change mitigation relative to current approaches, while at the same time improving the sector’s sustainability, ensuring food security and benefitting other well-being goals.
Sustaining well-being depends on keeping a balance between food provision and other ecosystem services (e.g. climate regulation and water quality), including those that will allow the production of food in the future. The measurement system used to inform policy decisions and track progress needs to reflect the impact of the policy actions on the different dimensions of well-being in both the present and the future. Only then can sustainability considerations be embedded in policy making.
A key issue for the agricultural sector – and food systems more generally – is ensuring that land use contributes to durable carbon sequestration, and that the mitigation demand for bioenergy emanating from other sectors of the economy does not undermine food security or drive unsustainable land-use conversion and associated CO2 emissions. The most stringent mitigation scenarios foresee a major role for bioenergy, which may raise challenges for food security and affordability, as well as for other areas of well-being. The trade-offs between mitigation and other well-being goals may be reduced if countries are able to take early mitigation action that decreases the need for the large-scale deployment of CO2 removal technologies (such as BECCS), and can constrain the growth in energy demand and achieve behavioural shifts resulting in different dietary choices (IPCC, 2018[5]).
By being aware of which situations produce a trade-off, what these trade-offs are and where they arise, policy makers can strike a balance between the provision of present and future services, as well as between visible (e.g. food provision) and invisible (e.g. nutrient cycle) services. Although specific goals will need to be prioritised, trade-offs can be minimised and synergies fostered, resulting in more effective, politically acceptable and coherent policies (i.e. two-way alignment).
6.4. Conclusion
The agriculture sector we have inherited in the 21st century represents one of the most important achievements of human civilisation, as it produces large amounts of food, which are more than sufficient to feed the world population. Paradoxically, it also results in some of the greatest challenges to the environment, health and overall well-being.
The way food is currently produced has significant environmental and climatic impacts. Furthermore, the degradation of biodiversity – and thus ecosystem services – caused by agricultural production threatens its own viability. In order to transition to a sustainable agriculture sector, this chapter argues that agriculture and climate change mitigation policies need to:
Take a food system approach that analyses change levers on both the supply (agriculture) and demand sides;
Look beyond food production and GHG emissions to analyse the sector’ sustainability in terms of ecosystem services and, more broadly, well-being.
Food systems are at the nexus of health and well-being, poverty alleviation, climate change and nature protection. Hence, they offer a unique opportunity to reduce GHG emissions and store carbon, while minimising trade-offs and creating synergies with other well-being goals. For example, GHG emissions can be reduced through policies promoting healthier diets. The need for social policies accompanying price increases due to the application of a GHG tax also becomes more evident when analysing social and economic impacts at the level of the food system. A broad and balanced set of policy measures, covering the entire food system, offers the greatest potential for climate change mitigation relative to current approaches, while at the same time improving the sector’s sustainability, ensuring food security and benefitting other well-being goals.
Sustaining well-being depends on keeping a balance between food provisions and other ecosystem services (e.g. climate regulation and water quality), including those that will allow the production of food in the future. The measurement system used to inform policy decisions and track progress needs to reflect the impact of policies on all the different dimensions of well-being in both the present and the future. Only then can sustainability considerations be embedded in policy making.
A key issue for the agricultural sector – and food systems more generally – is ensuring that land use contributes to durable carbon sequestration, and that the mitigation demand for bioenergy emanating from other sectors of the economy does not undermine food security or drive unsustainable land-use conversion and associated CO2 emissions. The most stringent mitigation scenarios foresee a major role for bioenergy, which may raise challenges for food security and affordability, as well as for other areas of well-being. The trade-offs between mitigation and other well-being goals may be reduced if countries are able to take early mitigation action that decreases the need for the large-scale deployment of CO2 removal technologies (such as BECCS), and can constrain the growth in energy demand and achieve behavioural shifts resulting in different dietary choices (IPCC, 2018[65]).
By being aware of which situations produce a trade-off, what these trade-offs are and where they arise, policy makers can strike a balance between the provision of present and future services, as well as between visible (e.g. food provision) and invisible (e.g. nutrient cycle) services. Although specific goals will need to be prioritised, trade-offs can be minimised and synergies fostered, resulting in more effective, politically acceptable and coherent policies (i.e. two-way alignment).
References
[33] AFSSA (2003), Evaluation nutritionnelle et sanitaire des aliments issus de l’agriculture biologique, http://www.afssa.fr (accessed on 27 August 2019).
[25] Alpro, |. and D. Murphy-Bokern (2010), Sustainable Eating-Part Understanding the Carbon Footprint of our Food, http://www.wrap.org.uk (accessed on 25 June 2019).
[43] American Diabetes Association (2018), “Economic Costs of Diabetes in the U.S. in 2017.”, Diabetes care, Vol. 41/5, pp. 917-928, http://dx.doi.org/10.2337/dci18-0007.
[3] Arneth, A. et al. (2019), IPCC Special Report on Climate Change, Desertification, Land Degradation, Sustainable Land Management, Food Security, and Greenhouse gas fluxes in Terrestrial Ecosystems Summary for Policymakers, IPCC, https://www.ipcc.ch/site/assets/uploads/2019/08/4.-SPM_Approved_Microsite_FINAL.pdf.
[38] Attina, T. et al. (2016), “Exposure to endocrine-disrupting chemicals in the USA: a population-based disease burden and cost analysis.”, The lancet. Diabetes & endocrinology, Vol. 4/12, pp. 996-1003, http://dx.doi.org/10.1016/S2213-8587(16)30275-3.
[34] Bailey, R., K. West Jr. and R. Black (2015), “The Epidemiology of Global Micronutrient Deficiencies”, Annals of Nutrition and Metabolism, Vol. 66/2, pp. 22-33, http://dx.doi.org/10.1159/000371618.
[50] Bajželj, B. et al. (2014), “Importance of food-demand management for climate mitigation”, http://dx.doi.org/10.1038/NCLIMATE2353.
[32] Barański, M. et al. (2014), Higher antioxidant and lower cadmium concentrations and lower incidence of pesticide residues in organically grown crops: A systematic literature review and meta-analyses, http://dx.doi.org/10.1017/S0007114514001366.
[24] Blandford, D. and K. Hassapoyannes (2018), “The role of agriculture in global GHG mitigation”, OECD Food, Agriculture and Fisheries Papers, No. 112, OECD Publishing, Paris, https://dx.doi.org/10.1787/da017ae2-en.
[47] Costanza, R. et al. (2014), “Changes in the global value of ecosystem services”, Global Environmental Change, Vol. 26, pp. 152-158, https://doi.org/10.1016/j.gloenvcha.2014.04.002.
[31] DeFries, R. et al. (2015), Metrics for land-scarce agriculture, http://dx.doi.org/10.1126/science.aaa5766.
[9] Díaz, S. et al. (2019), IPBES, Summary for policymakers of the global assessment report on biodiversity and ecosystem services of the Intergovernmental Science-Policy Platform on Biodiversity and Ecosystem Services, https://www.ipbes.net/sites/default/files/downloads/spm_unedited_advance_for_posting_htn.pdf.
[37] Dorward, A. (2013), “Agricultural labour productivity, food prices and sustainable development impacts and indicators”, Food Policy, Vol. 39, pp. 40-50, http://dx.doi.org/10.1016/J.FOODPOL.2012.12.003.
[55] EAT-Lancet Commission (2018), Summary Report of the EAT-Lancet Commission: Healthy Diets From Sustainable Food Systems, https://eatforum.org/content/uploads/2019/01/EAT-Lancet_Commission_Summary_Report.pdf (accessed on 30 January 2019).
[53] Epidemiology & Disease Control Division, S. Ministry of Health and Institute for Health Metrics and Evaluation. (2019), The Burden of Disease in Singapore, 1990–2017: An overview of the Global Burden of Disease Study 2017 results | Institute for Health Metrics and Evaluation, Seattle W.A, IHME, http://www.healthdata.org/policy-report/burden-disease-singapore-1990-2017 (accessed on 28 June 2019).
[12] Ewers, R. et al. (2009), “Do increases in agricultural yield spare land for nature?”, Global Change Biology, Vol. 15/7, pp. 1716-1726, http://dx.doi.org/10.1111/j.1365-2486.2009.01849.x.
[62] FAO (2016), Forests and Agriculture: Land-use Challenges and Opportunities, FAO, http://www.fao.org/3/a-i5588e.pdf (accessed on 4 June 2019).
[17] FAO (2015), Status of the World’s Soil Resources, Food and Agriculture Organisation - Intergovernmental Technical Panel on Soils (ITPS), http://www.fao.org/3/i5199e/I5199E.pdf (accessed on 1 April 2019).
[7] Fargione, J. et al. (2008), “Land clearing and the biofuel carbon debt.”, Science (New York, N.Y.), Vol. 319/5867, pp. 1235-8, http://dx.doi.org/10.1126/science.1152747.
[4] Field, C. et al. (2014), IPCC, 2014: Summary for policymakers. In: Climate Change 2014: Impacts,Adaptation, and Vulnerability. Part A: Global and Sectoral Aspects. Contribution of Working Group II to the Fifth Assessment Report of the Intergovernmental Panel on Climate Change, https://www.ipcc.ch/site/assets/uploads/2018/02/ar5_wgII_spm_en.pdf (accessed on 28 June 2019).
[29] Fujimori, S. et al. (2019), “A multi-model assessment of food security implications of climate change mitigation”, Nature Sustainability, Vol. 2, pp. 386-396, http://dx.doi.org/10.1038/s41893-019-0286-2.
[66] Gove, M. (2018), Secretary of State Michael Gove Speech: Farming for the next generation, https://www.gov.uk/government/speeches/farming-for-the-next-generation (accessed on 12 March 2019).
[68] Gustavsson, J. et al. (2011), Global Food Losses and Food Waste, http://www.fao.org/3/a-i2697e.pdf (accessed on 27 June 2019).
[1] Hardelin, J. and J. Lankoski (2018), “Land use and ecosystem services”, OECD Food, Agriculture and Fisheries Papers, No. 114, OECD Publishing, Paris, https://dx.doi.org/10.1787/c7ec938e-en.
[30] Hawkes, C. (2006), Uneven dietary development: Linking the policies and processes of globalization with the nutrition transition, obesity and diet-related chronic diseases, http://dx.doi.org/10.1186/1744-8603-2-4.
[59] Hellerstein, D., D. Vilorio and M. Ribaudo (2019), Agricultural Resources and Environmental Indicators, 2019, USDA, http://www.ers.usda.gov (accessed on 21 May 2019).
[20] Herrero, M. et al. (2013), “Biomass use, production, feed efficiencies, and greenhouse gas emissions from global livestock systems”, Proceedings of the National Academy of Sciences, Vol. 110/52, pp. 20888-20893, http://dx.doi.org/10.1073/pnas.1308149110.
[36] IFPRI (2016), Global Nutrition Report 2016 From Promise to Impact Ending Malnutrition by 2030, http://dx.doi.org/10.2499/9780896295841.
[65] IPCC (2018), IPCC: Global Warming of 1.5 C, https://ipcc.ch/report/sr15/ (accessed on 17 October 2018).
[5] IPCC (2018), IPCC: Global Warming of 1.5°C, https://ipcc.ch/report/sr15/ (accessed on 17 October 2018).
[21] IPCC (2013), Climate Change 2013: The Physical Science Basis. Contribution of Working Group I to the Fifth Assessment Report of the Intergovernmental Panel on Climate Change, https://www.ipcc.ch/report/ar5/wg1/ (accessed on 20 March 2019).
[58] Jean Chateau, R. (2018), “Impact of Green Growth Policies on Labour Markets and Wage Income distribution”, OECD Environment Working Paper, Vol. 137.
[19] Lankoski, J., A. Ignaciuk and F. Jésus (2018), “Synergies and trade-offs between adaptation, mitigation and agricultural productivity: A synthesis report”, OECD Food, Agriculture and Fisheries Papers, No. 110, OECD Publishing, Paris, https://dx.doi.org/10.1787/07dcb05c-en.
[41] McKinsey Global Institute (2014), Overcoming obesity: an initial economic analysis, http://www.mckinsey.com/mgi. (accessed on 18 February 2019).
[23] Myhre, G. et al. (2013), “Anthropogenic and Natural Radiative Forcing”, in Climate Change 2013: The Physical Science Basis. Contribution of Working Group I to the Fifth Assessment Report of the Intergovernmental Panel on Climate Change, Cambridge University Press, http://www.climatechange2013.org/images/report/WG1AR5_Chapter08_FINAL.pdf (accessed on 25 June 2019).
[10] Newbold, T. et al. (2014), “A global model of the response of tropical and sub-tropical forest biodiversity to anthropogenic pressures”, Proceedings Royal Society, Vol. 281/1792, http://dx.doi.org/10.1098/rspb.2014.1371.
[14] OECD (2019), Agricultural Policy Monitoring and Evaluation 2019, OECD Publishing, Paris, https://dx.doi.org/10.1787/39bfe6f3-en.
[63] OECD (2019), Biodiversity: Finance and the Economic and Business Case for Action, OECD, http://www.oecd.org/environment/resources/biodiversity/G7-report-Biodiversity-Finance-and-the-Economic-and-Business-Case-for-Action.pdf (accessed on 4 June 2019).
[48] OECD (2019), “Report prepared for the G7 Environment Ministers’ Meeting, 5-6 May 2019”, in Biodiversity: Finance and the Economic and Business Case for Action.
[15] OECD (2018), Human Acceleration of the Nitrogen Cycle: Managing Risks and Uncertainty, OECD Publishing, Paris, https://dx.doi.org/10.1787/9789264307438-en.
[60] OECD (2013), OECD Compendium of Agri-environmental Indicators, OECD Publishing, Paris, https://dx.doi.org/10.1787/9789264186217-en.
[52] OECD forthcoming (2019), “Towards policies encouraging healthier food choices”.
[39] Paulson, J. et al. (2015), “Nontherapeutic Use of Antimicrobial Agents in Animal Agriculture: Implications for Pediatrics”, PEDIATRICS, Vol. 136/6, pp. e1670-e1677, http://dx.doi.org/10.1542/peds.2015-3630.
[22] Pierrehumbert, R. (2014), “Short-Lived Climate Pollution”, Annual Review of Earth and Planetary Sciences, Vol. 42/1, pp. 341-379, http://dx.doi.org/10.1146/annurev-earth-060313-054843.
[49] Poore, J. and T. Nemecek (2018), “Reducing food’s environmental impacts through producers and consumers.”, Science (New York, N.Y.), Vol. 360/6392, pp. 987-992, http://dx.doi.org/10.1126/science.aaq0216.
[6] Popp, A. et al. (2017), “Land-use futures in the shared socio-economic pathways”, Global Environmental Change, Vol. 42, pp. 331-345, http://dx.doi.org/10.1016/J.GLOENVCHA.2016.10.002.
[56] Popp, A., H. Lotze-Campen and B. Bodirsky (2010), “Food consumption, diet shifts and associated non-CO2 greenhouse gases from agricultural production”, Global Environmental Change, Vol. 20/3, pp. 451-462, http://dx.doi.org/10.1016/J.GLOENVCHA.2010.02.001.
[45] Potts, S. et al. (2016), Summary for policymakers of the assessment report of the Intergovernmental Science-Policy Platform on Biodiversity and Ecosystem Services on pollinators, pollination and food production, https://www.ipbes.net/system/tdf/downloads/pdf/ipbes_4_19_annex_ii_spm_pollination_en.pdf?file=1&type=node&id=28363 (accessed on 18 February 2019).
[54] Poux, X. and P. Aubert (2018), Une Europe agroécologique en 2050 : une agriculture multifonctionnelle pour une alimentation saine, IDDRI, http://www.iddri.orgUneEuropeagroécologiqueen2050:uneagriculturemultifonctionnellepourunealimentationsaine (accessed on 30 January 2019).
[11] Rudel, T. et al. (2009), Agricultural intensification and changes in cultivated areas, 1970-2005, http://www.pnas.org/cgi/content/full/ (accessed on 4 March 2019).
[44] Sánchez-Bayo, F. and K. Wyckhuys (2019), Worldwide decline of the entomofauna: A review of its drivers, http://dx.doi.org/10.1016/j.biocon.2019.01.020.
[46] Sandhu, H. et al. (2015), “Significance and value of non-traded ecosystem services on farmland”, PeerJ, http://dx.doi.org/10.7717/peerj.762.
[35] Schaible, U. and S. Kaufmann (2007), “Malnutrition and infection: complex mechanisms and global impacts.”, PLoS medicine, Vol. 4/5, p. e115, http://dx.doi.org/10.1371/journal.pmed.0040115.
[64] Scheierling, S. and D. Tréguer (2018), Beyond Crop per Drop - Assessing Agricultural Water Productivity and Efficiency in a Maturing Water Economy, World Bank Group, http://documents.worldbank.org/curated/en/352321530075399351/pdf/127625-PUB-Date-6-28-2018-PUBLIC-Beyond-Crop-per-Drop.pdf (accessed on 27 June 2019).
[26] Schnell, S. (2013), “Food miles, local eating, and community supported agriculture: putting local food in its place”, Agriculture and Human Values, Vol. 30/4, pp. 615-628, http://dx.doi.org/10.1007/s10460-013-9436-8.
[8] Searchinger, T. and R. Heimlich (2015), “Avoiding Bioenergy Competition for Food Crops and Land”, World Resources Institute, https://www.wri.org/publication/avoiding-bioenergy-competition-food-crops-and-land (accessed on 25 June 2019).
[2] Smith, P. et al. (2014), Agriculture, Forestry and Other Land Use (AFOLU), IPCC, https://www.ipcc.ch/site/assets/uploads/2018/02/ipcc_wg3_ar5_chapter11.pdf (accessed on 30 January 2019).
[16] Smith, P. et al. (2014), AR5 Chapter11: Agriculture, Forestry and Other Land Use (AFOLU), IPCC, https://www.ipcc.ch/site/assets/uploads/2018/02/ipcc_wg3_ar5_chapter11.pdf (accessed on 4 June 2019).
[27] Smith, P. et al. (2016), “Biophysical and economic limits to negative CO2 emissions”, Nature Climate Change, Vol. 6/1, pp. 42-50, http://dx.doi.org/10.1038/nclimate2870.
[28] TEEB (2018), TEEB for Agriculture & Food: Scientifc and Economic Foundations, http://teebweb.org/agrifood/wp-content/uploads/2018/11/Foundations_Report_Final_October.pdf (accessed on 2 August 2019).
[67] UNEP (1994), Convention on Biological Diversity, UNEP, Geneva.
[18] Vermeulen, S., B. Campbell and J. Ingram (2012), “Climate Change and Food Systems”, http://dx.doi.org/10.1146/annurev-environ-020411-130608.
[13] WHO (2018), Malnutrition, https://www.who.int/news-room/fact-sheets/detail/malnutrition (accessed on 24 July 2019).
[69] WHO (2018), Obesity and overweight, World Health Organisation, https://www.who.int/news-room/fact-sheets/detail/obesity-and-overweight (accessed on 24 July 2019).
[40] WHO (2017), Stunted Growth and Development. Context, Causes and Consequences, WHO, https://www.who.int/nutrition/childhood_stunting_framework_leaflet_en.pdf?ua=1 (accessed on 4 June 2019).
[42] WHO (2016), Global report on diabetes, http://www.who.int/about/licensing/copyright_form/index.html (accessed on 18 February 2019).
[61] Willett, W. et al. (2019), “Food in the Anthropocene: the EAT-Lancet Commission on healthy diets from sustainable food systems”, The Lancet Commissions, http://dx.doi.org/10.1016/S0140-6736(18)31788-4.
[51] Wollenberg, E. et al. (2016), “Reducing emissions from agriculture to meet the 2 °C target”, Global Change Biology, Vol. 22/12, pp. 3859-3864, http://dx.doi.org/10.1111/gcb.13340.
[57] WRI (2018), Creating a sustainable food future, World Resources Institute, https://www.wri.org/our-work/project/world-resources-report/publications. (accessed on 20 February 2019).
Notes
← 1. Creating two-way alignment between climate action and the broader goals of human well-being and sustainable development means that: i) action in non-climate policy areas should support, rather than undermine, the pursuit of climate change mitigation goals; and ii) climate change mitigation will be more attractive when it also meets other important societal goals (e.g. clean air and improvements in health) that are likely to materialise over a shorter timescale.
← 2. “Food systems” are defined here as all the stages needed to feed the population, from producing, to transforming, distributing, consuming and disposing of food (Gustavsson et al., 2011[68])
← 3. This figure excludes CO2 emissions from agriculture. Fossil-fuel use for machinery is captured in the energy sector.
← 4. Source: FAOstat.
← 5. Ecosystem services are the gains humans get from properly functioning ecosystems. They include supporting services (habitat), provisioning services (food, freshwater), regulating services (climate regulation, pest control) and cultural services (tourism, recreation, folklore).
← 6. See, for example the UK Secretary of State for the Department of the Environment, Food and Rural Affairs’ speech of 4 January 2018: “The pressures placed on our global environment by this growth … will be formidable – whether it’s greenhouse gas emissions in our atmosphere contributing to global warming, desertification and soil erosion reducing the space for cultivation, deforestation leading to the disappearance of valuable carbon sinks and precious habitats, air pollution from traditional industry and intensive agriculture adding to health costs, waste poisoning our oceans or iconic landscapes under threat from the need for further development. Without action we face the progressive loss of the natural capital on which all growth – natural, human and economic – ultimately depends” (Gove, 2018[66]).
← 7. See Chapter 1 for more information on adopting a well-being lens.
← 8. The water storage capacity of soils increases in soils with higher organic matter.
← 9. Biodiversity is defined here as “the variability among living organisms from all sources including, inter alia, terrestrial, marine and other aquatic ecosystems and the ecological complexes of which they are part; this includes diversity within species, between species and of ecosystems” (UNEP, 1994[67]).
← 10. This figure is from TEEB, a framework modelled by the United Nations Environment Programme which provides a full-cost accounting and allows better understanding of the origins of potential trade-offs in the food system.
← 11. The study also notes that the direct impact of climate change on yields was not assessed and the benefits of climate mitigation in terms of avoided yield loss could be substantial.
← 12. Overweight is defined by the World Health Organization as a body mass index superior to 25 (WHO, 2018[69]).
← 13. This is an average number for the world population and does not apply to the whole population everywhere, as many populations face undernutrition issues.
← 14. There can be exceptions, e.g. in the case of plants grown in heated greenhouses or transported by airfreight, which can emit more GHG per unit of protein than some animal products.
← 15. The 20 Aichi Targets were set at the 2010 Convention on Biodiversity as part of the 2011-2020 Strategic Plan for Biodiversity.
← 16. Ensure fair income, increase competitiveness, rebalance power in food chain, climate change action, environmental care, preserve landscapes and biodiversity, support generational renewal, vibrant rural areas, protect food and health quality.
← 18. To respond to these issues, the OECD launched the Network on Agricultural Total Factor Productivity and the Environment in 2017, gathering experts in order to build a co-ordinated framework for an “environmentally adjusted total factor productivity” indicator that allows international comparisons.
← 19. Research on such an indicator is part of the ongoing Changing Access to Nutritious Diets in Africa and South Asia project conducted by the Friedman School of Nutrition at Tufts University.
← 20. Agricultural land includes arable land (temporary crops, temporary meadows, land under market, kitchen gardens and temporary fallows), permanent crops and permanent pastures.
← 21. Directive 2000/60/EC of the European Parliament and of the Council of 23 October 2000 establishing a framework for Community action in the field of water policy.