This chapter demonstrates that urban passenger transport plays a vital role in curbing greenhouse gas emissions, boosting access to opportunities and spurring economic recovery from the Covid-19 pandemic. It presents three scenarios for future urban passenger transport demand and resulting CO2 and local pollutant emissions, as well as the impacts on accessibility for citizens. It also discusses how decarbonisation initiatives can help to reduce inequalities and make urban transport systems more resilient.
ITF Transport Outlook 2021
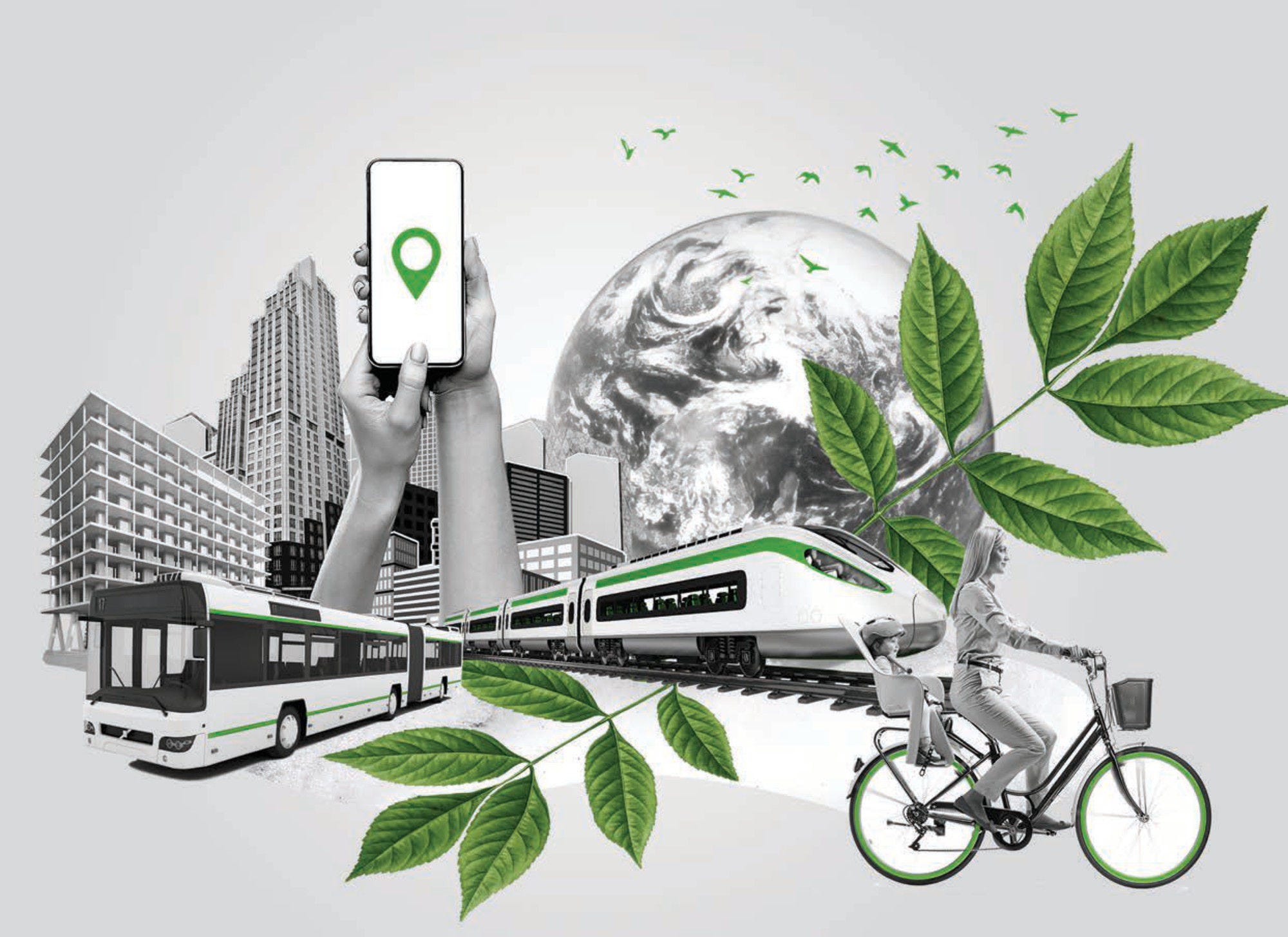
3. Urban passenger transport: Cities can make mobility sustainable, equitable and resilient
Abstract
In Brief
Continued urbanisation demands sustainable, accessible and resilient transport
Urban travel is responsible for 40% of all greenhouse gas emissions from passenger transport. Between 2015 and 2050, demand for urban passenger transport is poised to more than double after a temporary dip due to Covid-19. Unless cities succeed in cutting their transport emissions, the increase in urban mobility could jeopardise the climate goals of the Paris Agreement.
Urban passenger transport emissions could be cut nearly 80% by 2050 if more ambitious actions are taken than those foreseen under current commitments - despite the growing mobility demand cities will have to accommodate in the face of continuing urbanisation. Under ambitious scenarios, citizens will travel in smarter and more sustainable ways while enjoying better access to their desired destinations. By contrast, under current policies urban transport emissions would be at about the same level 30 years from now, decreasing by only 5%.
Avoiding unnecessary trips, shifting to more sustainable transport and improving vehicle and fuel technologies will prove decisive. Reducing our reliance on cars in cities is pivotal to decarbonise urban mobility. Three‑quarters of all emissions from urban passenger transport come from private vehicles. In 2015, they accounted for half of global urban travel, or 2.6 times all public transport activity. The economic, environmental, and social costs are significant: excessive car use causes health problems, increases social inequalities, cements our dependence on fossil fuels, and perpetuates congestion.
Improved public, shared and active transport services, coupled with fewer incentives to use private vehicles in cities, would accelerate decarbonisation and make the opportunities cities offer more accessible for a greater number of citizens. Integrating land-use planning with transport policy will support less costly, less emitting and less space-consuming ways to travel around cities than cars.
Our urban transport systems would also become more resilient under stringent climate policies. A greater variety of travel choices for citizens means less reliance on one form of transport and thus flexibility to absorb disruptions. The pathways to sustainable, equitable and resilient urban transport lie before us. Now we need ambitious policies in place that steer us in the right direction.
Policy recommendations
Empower cities to decarbonise urban mobility and enhance accessibility to improve well-being.
Prioritise funding for sustainable urban transport over investment in city roads.
Improve the quality of public transport to create more inclusive and reliable services.
Pursue integrated land-use and transport planning for sustainable, neighbourhood-based urban development.
Create incentives for greening urban vehicle fleets.
Nurture transport innovation and collaborate with providers of new urban mobility services to maximise benefits and minimise costs.
Combine transport decarbonisation and resilience measures now to meet future demand in sustainable ways and withstand disruptions.
As the world becomes progressively more urban, passenger transport faces growing demand in cities across the globe. Urban trips far outnumber all other passenger trips worldwide. Under current policies, ITF estimates a 163% global increase in travel activity by 2050 compared to 2015 levels. Cities have long been hubs for creativity and innovation, thanks to their density of infrastructure, people, and services. Despite the uncertainties of pandemic recovery, they are uniquely positioned to be at the forefront of equitable climate mitigation solutions that meet increasing demand sustainably. Under the right conditions growing urbanisation could be an opportunity, instead of a challenge, for decarbonising transport
Urban passenger transport is responsible for 40% of all passenger transport greenhouse gas (GHG) emissions. ITF scenarios show that by adopting highly ambitious decarbonisation policies and leveraging pandemic recovery to focus on decarbonisation efforts, urban passenger transport-related CO2 emissions could be reduced by nearly 80% by 2050, compared to 2015. Integrated transport and land-use planning to create denser neighbourhoods with transit-oriented development (TOD) and provisions for safe active and micromobility are vital to reducing trip lengths and making sustainable modes a convenient choice. Estimates suggest that disruptions such as shared mobility and micromobility have an increasingly important role in the sustainable mobility landscape, if well integrated with public and active transport. Improving vehicle technologies in private and public fleets, as well as reallocating and redesigning road space to better support sustainable modes is also imperative.
Covid-19 had an unprecedented impact on urban transport. Cities saw public transport use, road traffic and everyday mobility collapsing to record low levels due to containment measures. However, the suppression of demand will probably not last in the long term. Travel by private vehicles recovered considerably in many cities worldwide between containment efforts while public transport did not. It may suffer longer-term losses without policy intervention. Despite the challenges of the pandemic, recovery does present potential opportunities to reshape our future trajectory. The ability to capitalise on these opportunities will depend on local governments' initiatives and funding support from national stimulus packages.
Cities are at a crossroads. They are striving to recover economically from the pandemic. They face the ever-mounting consequences of climate change. They are on the front line in the fight to tackle rising social inequality. Urban transport has a vital role in economic recovery, climate change mitigation and reducing social inequalities. But economic, environmental and social policies must align. Such alignment will also enhance public support and cost-effectiveness of the required policies. A change in perspective is needed to align policy goals: away from a siloed approach with a single objective and negative externalities; towards system-wide thinking that analyses the impacts of policies on multiple objectives and considers the interdependencies between them.
So what does this shift in perspective mean for transport policy-making?
Policy should shift from accommodating increasing traffic growth and transport volumes to improving access to opportunities. Authorities can do so by supporting integrated approaches to land‑use and transport planning and prioritising demand-side policies that reduce the need for travel or shift travel to more sustainable modes. A more equitable system that allows residents to access a variety of opportunities and services via sustainable modes, conveniently, affordably, and over shorter distances is central to meeting environmental objectives as well. Authorities face massive challenges as they develop new policy agendas under the added uncertainty of pandemic recovery. This Transport Outlook assesses what urban transport might look like under three different global policy scenarios. The results show potential changes in transport activity, CO2 emissions, and local pollutants under different policies. CO2 emissions represent total GHGs as CO2 equivalents. The results provide a starting point for these decisions.
Decarbonising urban passenger transport: The state of play
Three-quarters of all urban transport GHG emissions came from passenger transport in 2015, according to ITF estimates. The high density of infrastructure, people and services in cities provides greater potential for non-motorised, shared, and public-transport-based mobility compared to non-urban areas. Yet many urban areas are dominated by individual motorised transport with associated problems of GHG emissions, air pollution, noise, traffic injuries and congestion. These externalities lead to adverse health outcomes, social inequalities and affect the overall well‑being of urban dwellers. The related economic, environmental and social costs are too big to overlook.
Authorities around the world are paying increased attention to urban transport decarbonisation within their broader transport policy commitments. Almost 40% of countries mention some form of urban passenger transport-related measures in their nationally determined contributions (NDCs) under the 2015 Paris Agreement (ITF/OECD, 2018[1]). They include 54 developing and fast-growing economies (GIZ, 2017[2]). Measures proposed by local authorities in select urban areas worldwide further add to their countries’ commitments. For instance, 167 cities across the planet have committed to collaborating on actions to reduce GHG emissions in all major sectors, including transport. Out of these, 54 have developed climate action plans compatible with the Paris Agreement (C40, 2020[3]).
Urban transport systems are at risk of disruption due to climate change and other events like pandemics. Resilient systems designed to resist, absorb and adapt to disruptions' impacts without halting delivery of transport services become increasingly important as the planet faces a rise in extreme natural events due to climate change (Ahmed and Dey, 2020[4]). Floods, rainstorms, droughts or higher‑than‑normal variations in urban temperatures have immediate and long-term negative impacts on transport infrastructure and services (Zhou, Wang and Yang, 2019[5]; CDP, 2020[6]). Furthermore, transport is more dependent than ever on Information and Communication Technologies (ICT). Disruptions in transport, communication or power systems could reduce or even temporarily eliminate access for inhabitants of affected urban areas.
In an equitable transition to sustainable transport, environmental ambitions go in hand with promoting wider well‑being. Any efforts to decarbonise the sector must not unfairly burden certain groups over others. A shift from traditional mobility-focused planning to one that prioritises accessibility will be instrumental in promoting both goals. Mobility is not an end in itself but rather a means to an end. It provides adequate access to jobs, education, health centres and other essential, thus improving citizens’ well‑being. (OECD/ITF, 2019[7]; OECD, 2019[8]). Vulnerable populations are already the most disadvantaged in terms of transport access and climate impacts, shouldering disproportionate costs from the travel decisions of others (Banister, 2018[9]; Sustainable Development Commission, 2011[10]; Gough, 2011[11]). Cities should take special care to reverse, not exacerbate, this trend with decarbonisation policies.
Routes to decarbonisation will differ between countries and cities. The challenges of developing equitable, sustainable, and resilient urban transport systems vary from country to country, from city to city. Current levels of per-capita emissions differ dramatically between OECD and non-OECD countries, and urbanisation patterns that drive demand for transport also differ between world regions.
City-dwellers in OECD countries have the largest transport carbon footprint. The highest emitting cities produce 28 times those in the least emitting. Urban inhabitants of OECD countries emit the most CO2 per person, while people living in cities across Africa and some parts of Asia emit the least. Measures in OECD and some fast-growing economies will need to decrease urban transport-related emissions per capita. Non-OECD economies will need to focus on limiting the increase of per capita emissions while meeting growing transport demand. Figure 3.1 shows the average levels of CO2 per capita generated by urban passenger transport worldwide in 2015, split into eight categories.
Figure 3.1. CO2 emissions per capita of urban passenger transport in 2015
Kilograms CO2 emissions per capita

Note: Figure depicts ITF modelled estimates. Averages by region are calculated as averages across all urban areas.
A growing world population combined with fast-paced urbanisation will inevitably increase transport demand in cities. By 2050, almost seven billion people will live in cities, approximately the entire world population of 2015 (UN, 2018[12]). Cities in developing countries will grow most over the next thirty years. Sub-Saharan Africa's urban population will increase at the fastest pace, almost tripling between 2020 and 2050. In Asia, the urban population will nearly double in the same period. Authorities in these regions will be hard-pressed to meet this growing demand in sustainable ways.
Individual motorised transport dominates most cities. In 2015, more than a third of passenger trips were made by private vehicles, 2.5 times those made with public transport. These trips accounted for more than half of all urban passenger-kilometres in that year. The adverse health effects, social inequalities, fossil fuel dependence and congestion caused by excessive car use entail high economic, environmental and social costs. Yet projections see the global private passenger vehicle fleet growing by more than 30% between 2020 and 2030, reaching 1.4 billion vehicles by 2050 (IEA, 2020[13]). Already in 2015, private vehicle use generated three-fourths of all urban passenger transport-related GHG emissions worldwide (Figure 3.2). This is mostly the result of continued growth in both private vehicle ownership and increasing average vehicle size. The United States and Canada, taken together as one region, have 733 vehicles per 1 000 inhabitants and the highest share of emissions from private car use in international comparison (OICA, 2020[14]). The growing demand for larger sports utility vehicles (SUVs) is further challenging emission reduction. Nearly half of all cars sold in the United States in 2018 were SUVs, and worldwide the share of new SUVs has doubled compared to a decade ago. (IEA, 2019[15]).
How can cities handle growing mobility demand?
Policies to avoid unnecessary travel or shorten trips are crucial but must still provide good access. Some urban decarbonisation strategies rely heavily on the development and uptake of zero-emission technologies. To achieve the climate objectives of the Paris Agreement targets, avoid and shift policies must be equally central if the aim is to establish an equitable as well as a sustainable transport system. Shifting necessary travel to less carbon- and space-intensive modes reduce environmental, social and economic side effects. Cities will also need to foster the adoption of improved technologies and increase average vehicle fuel efficiency. These policies are complementary and should be applied in a balanced manner (Gota et al., 2019[16]) based on what is most appropriate for the region.
Integrated planning of transport and land use is essential to making journeys shorter and sustainable. Compact urban development patterns co‑ordinated with public transport planning prevents inefficient and costly patterns of development. Mixed land-uses and compact development allow residents to access their needs without travelling long distances. Transit-oriented development (TOD), commonly defined as mixed-use urban development within close proximity (walking distance) to mass-transit facilities, can deliver on this goal. It concentrates higher-density, mixed development near access points for public transport. This makes using public transport convenient, encourages ridership and decreases car dependency.
The amount of space given to different transport modes does not match their relative importance for sustainable transport
Cars should be allocated less urban space. The amount of space given to different transport modes does not match their relative importance in a sustainable transport offer. Cars are the most space-intensive mode of transport, and space in many cities is devoted mainly to cars. As an example, around 60% of road space in Freiburg (Germany) was dedicated to cars in 2016, while they made only 30% of trips. Cycling also accounted for around 30% of trips, but cycling infrastructure only made up around 4% of all road infrastructure (Gössling et al., 2016[17]). This brings negative environmental but also social and economic consequences. For instance, they are fast and heavy, making them potentially dangerous for more vulnerable road users such as pedestrians, cyclists and, increasingly, users of micromobility (ITF, 2021[18]). Reallocating road space to active modes, installing priority lanes for public transport and limit parking space can help cities to shift the mode share away from cars. The rising popularity of micromobility is putting pressure on existing roads and limited cycling infrastructure. Successfully integrating them with the transport network makes space reallocation even more important. Shared mobility will also change the way cities manage pavement space. Increasingly, pavements will resemble flexible, multi-use spaces that allow for pick-up and drop-off of passengers as priorities shift away from parking for private cars in dense city areas (ITF, 2018[19]).
Managing road space depends on evolving passenger and freight transport needs. Deliveries by light commercial vehicles are growing. Bicycle couriers and growing micromobility put additional pressure on urban space. However, jointly managing urban passenger and freight transport offers an opportunity for better allocating road space and reducing congestion. At the same time, it improves the distribution of transport flows (Pimentel and Alvelos, 2018[20]). The main trends in urban freight transport across the globe are discussed in Chapter 5. A better understanding of the linkages between passenger and freight urban transport activities and measures will come from more research into these issues.
Car users must pay the real cost of parking and driving. Most drivers only pay a fraction of the costs associated with urban car traffic; car use thus greatly exceeds the optimum (ITF, 2021[18]). This inefficiency will persist as long as prices are not equal to the marginal social costs or other measures constrain traffic. Various economic instruments and regulatory measures can optimise demand and mitigate congestion. These include different forms of road charges, parking pricing, vehicle restriction schemes and others. Carbon pricing would apply to all CO2-emitting modes but particularly target private cars with internal combustion engines, which emit most CO2 per passenger-kilometre. All efforts to reduce car use should be accompanied by investments in low-carbon alternatives to car travel.
Uptake of new technologies requires investment and incentives. Policies to promote technologies that offer alternatives to private vehicles include purchase incentives for electric and other low-carbon vehicles, investment in charging infrastructure, fuel economy standards (GIZ, 2019[21]). New services such as shared mobility can operate more efficiently than private vehicles through optimised routing, increased load factors and better capacity use.
Self-driving cars and electric vehicles are no panacea for curbing emissions. Future transport emissions will not fall to the required levels through automation and electrification alone (Fulton et al., 2017[22]). Automated and electrified cars are only a part of the solution, not the solution, because of implementation challenges and the externalities they create. For example, the fast-growing share of electric vehicles in some developed and fast-growing economies do not address the negative externalities from congestion, regardless of their energy efficiency. Also, electric vehicles reduce local emissions and improve air quality, but they will only contribute to decarbonisation if powered with clean electricity. Automated cars bring the risk of increasing congestion in cities, among others by facilitating empty runs. Because of their limitations, technical improvements like automation and electrification will only yield sustainable gains in transport decarbonisation if combined with other measures in a holistic approach. This includes policies aimed at reducing demand and a shift to sustainable modes.
Fleet improvements in mass passenger transport systems will be particularly important in developing nations. In 2015, Latin America and the Caribbean and Sub-Saharan Africa were the only two global regions where public transport was responsible for most GHG emissions, given the technologies used in both formal and paratransit services (see Figure 3.2). The average fleet age in these regions varies from city to city but can be as high as 20 years in cities such as Lima in Peru and Conakry in Guinea (Salazar Ferro, 2015[23]). Fleet improvement and electrification programmes are essential in these regions to decrease emissions in the future. However, the current regulatory ambiguity of paratransit service operations poses challenges to fleet renewal (The World Bank, 2019[24]).
New shared mobility services have great potential to reduce the need for private vehicles. In combination with alternative fuels, such innovative services could achieve significant emission reductions. A lot of uncertainty surrounds the widespread adoption of shared mobility, however (Fulton et al., 2017[22]). It will require solid supportive policies and financial incentives to ensure that services with higher load factors are succeeding, rather than services that create additional traffic (ITF, 2020[25]; ITF, 2016[26]). Currently, shared mobility services are offered mainly by private-sector operators. Examples of collaboration with local authorities exist, however. In Mexico City, shared mobility operator Jetty was working with the city to fill service gaps in public transport. This contributed to a shift from private cars to Jetty’s vanpooling service, particularly among higher-income users. Other forms of “agile mobility”, for instance, electrified two- and three-wheelers, have appeared in urban areas in developing countries such as Nepal and Colombia. These services can often complement existing public transport services for the last mile (ITF, 2019[27]).
Figure 3.2. Mode share of urban passenger CO2 emissions by world region in 2015

Note: Figure depicts ITF modelled estimates. Active mobility and micromobility include walking, biking, scootersharing, and bikesharing. Public transport includes PT rail, metro, bus, LRT, and BRT. Paratransit includes informal buses and PT three-wheeler. Shared vehicle includes motorcycle and carsharing. Private Vehicle includes motorcycles and cars. Shared mobility includes taxis, ridesharing, and taxi buses. EEA: European Economic Area. LAC: Latin America and the Caribbean. MENA: Middle East and North Africa. OECD Pacific: Australia, Japan, New Zealand, South Korea. SSA: Sub-Saharan Africa. Transition economies: Former Soviet Union and non-EU South-Eastern Europe.
Public authorities must manage urban transport innovation so it will deliver the maximum social and environmental benefits (ITF, 2019[28]). This requires digital integration that facilitates ticketing, fare and routing co‑ordination with existing public transport, as well as the integration of schedules and physical urban space that allow for seamless modal transfers. Mobility as a Service (MaaS) solutions show promise and could serve as an example for this, but no implemented best practices exist yet. An unregulated approach could result in adverse environmental impacts. Forms of shared mobility could bring about between 28% and 62% reductions of CO2 emissions, respectively, for the Helsinki and the Lisbon metropolitan areas, if integrated and in co‑operation with existing public transport services, ITF simulations show (ITF, 2020[25]).
Implementation of some of the transport policy measures during recovery from the Covid-19 pandemic will prove difficult. In contrast, others may be easier to put into place due to changes in behaviour during the pandemic and the substantial investment into the recovery. The following section details the impact of the Covid-19 pandemic on urban transport and revisits policy interventions from the context of recovery.
Mastering the pandemic: Challenges and opportunities for urban mobility after Covid‑19
The global response to the Covid-19 pandemic ranged from limits on gatherings to strict national lockdowns. As a result, passenger transport activity in cities almost came to a halt. In April 2020, cities such as Milan, New York City or London registered less than 10% of typical mobility levels (Citymapper, 2020[29]). The ITF estimates overall urban transport activity in 2020 at 19% of previously anticipated annual demand. As the pandemic lingers, many uncertainties about its impact on urban mobility remain. Public transport has become a major casualty of Covid-19. Walking, cycling and micromobility are enjoying a surge, on the other hand, supported by many city authorities. The pandemic will likely leave a legacy of transformative change and policy makers will need to channel that impetus towards outcomes that set the planet on the right trajectory. The policies implemented as part of the pandemic recovery will determine whether decarbonisation is thwarted or tacks to the fast lane. A list of opportunities for and challenges to, decarbonisation in the long term is given in Table 3.1, together with a summary of the short-term impacts of Covid-19 on urban transport.
Table 3.1. Potential challenges and opportunities for decarbonising urban transport post-Covid-19
Impacts |
Potential opportunities for decarbonisation |
Potential challenges for decarbonisation |
---|---|---|
Short‑term impacts |
|
|
Long‑term/structural changes |
|
|
Note: Short-term impacts are based on observed changes in travel behaviour during the pandemic that hurt or hinder decarbonisation efforts. Most long‑term and structural opportunities rely on well-designed recovery policies, while challenges add constraints to future decarbonisation.
Cities at a standstill
Teleworking became the new norm” during the lockdown”. In the United States and European Union, approximately 48% and 42% of the workforce, respectively, was working from home (Sostero et al., 2020[30]; Bloom, 2020[31]). Confinement measures led to drastic declines in commuting and leisure trips (Google LLC, 2020[32]). For those unable to telework, economic consequences have been significant. In developing economies, where informal work is more prevalent, stay-at-home orders had particularly adverse impacts on incomes.
Lower traffic volumes rapidly reduced air pollution in cities from Manchester to Mumbai. Lockdowns resulted in a 60% reduction in NO2 and a 31% reduction in particulate matter levels in a study of 34 countries. The NO2 reductions, in particular, are credited to the dramatic drops in traffic (Venter et al., 2020[33]). Congestion and its externalities were virtually eliminated in cities like Beijing and Mumbai. Other urban centres recorded substantial reductions at least during the height of lockdown measures (TomTom, 2020[34]).
Public transport was a primary casualty of the pandemic. Typically the backbone of a sustainable and efficient urban transport system, passenger numbers on public transport fell drastically and have not yet recovered. To curb the spread of Covid-19, authorities urged citizens to use alternative modes to enable better social distancing. Many cities observed a 70‑95% drop in ridership, while some faced as much as a 97% loss (Puentes, 2020[35]). The corresponding decrease in revenue is severe, and in some cases, public transport services were drastically cut as a result. Routes were suspended, schedules reduced, and occasionally mass transport was completely suspended (Dormer, 2020[36]; de la Garzia, 2020[37]; BBC, 2020[38]).
Reductions in public transport services hit essential workers and vulnerable groups particularly hard. According to a US-based study, 36% of public transport commuters under normal conditions are essential workers, and 67% of them are from ethnic minorities (TransitCenter, 2020[39]). Privately owned paratransit services shut down because of a lack of riders. This eliminated a connectivity option, notably for poorer neighbourhoods in many developing countries, and left many low-income workers with no choice but to walk or cycle long distances (IGC, 2020[40]).
Public transport operators have pivoted and adapted their operations to maintain services during the Covid-19 crisis. Measures aimed at ensuring essential services, especially for essential workers. In many cities, buses and trains continued to operate with reduced capacity limits, often only 15% of the maximum. They quickly installed plastic barriers to ensure separation and protection of transport personnel. Ending on-board ticket sales and front-door boarding and adding markings to communicate distancing requirements were other popular measures (McArthur and Smeds, 2020[41]; UITP, 2020[42]).
Shared mobility was also hit by the pandemic. Demand for ridesharing and vehicle sharing programmes fell substantially, leading to a temporary suspension of services in most cities at the height of the pandemic. Many have since resumed operation with enhanced sanitary and barrier measures in place. Some ridesharing companies have introduced new services such as food delivery to regain lost revenue (Ibold et al., 2020[43]).
Walking and cycling are booming. For citizens uncomfortable using public transport for fear of exposure to the Coronavirus, active mobility became the transport option of choice. Large numbers of people made use of empty streets to run errands and exercise. Since the start of the pandemic, 1 800 cities have deployed temporary cycling and pedestrian infrastructure, closed roads, changed signalling and introduced other measures to support this shift (Goetsch and Quiros, 2020[44]). Bicycle suppliers, bikeshare operators, repair shops and cycle-to-work schemes have reported strong demand increases (BBC, 2020[45]). Data for walking is lacking.
Recovery risks – and opportunities
Orchestrating the recovery from a global pandemic is new territory. Uncertainty abounds about how long the pandemic threat will last and what life will look like as we learn to live with the virus. Covid-19 has introduced substantial risks for cities’ sustainable transport agendas, as witnessed by the hit taken by public transport. Yet there are also opportunities to seize, notably to lock in positive behavioural change adopted by citizens, for now, by the pandemic.
Teleworking and its interdependence with transport dominates the discussion about post‑pandemic cities. Much debate currently centres on telework's potential to bring down commuting levels and thereby decrease urban emissions. It is not yet certain what the net impact of teleworking will be. An increase in non-work trips often accompanies a drop in commuting, which may offset any gains (Hook et al., 2020[46]; Zhu et al., 2018[47]). Similarly, it is unclear whether the increase in energy use at home associated with teleworking will also cancel out some CO2 reductions (IEA, 2020[48]). Even if teleworking has net CO2 savings, it is still limited disproportionately to well-educated individuals in higher‑paying jobs in developed economies which typically have a greater share of knowledge-sector workers (Dingel and Neiman, 2020[49]). The negative impact on social equity became clear during the pandemic when only those enjoying the privilege of working from home did not suffer income losses (Bloom, 2020[31]; Guyot and Sawhill, 2020[50]).
The pandemic is the opportunity to adopt a more sustainable funding model which properly reflects the social and environmental benefits that only public transport provides
Public transport operators are reeling at the loss of fare revenues during the pandemic. These losses will continue for the immediate future as operators comply with distancing rules that keep ridership down and operating costs up. The situation has prompted a massive budget crisis in public transport. Transport for London estimated a GBP 6.4 billion (USD 8.9 billion) funding shortfall between 2020 and 2022. Hong Kong’s Mass Transit Railway estimates losses during the first half of 2020 to be HKD 400 million (USD 51.6 million) (McArthur and Smeds, 2020[41]). In Brazil, the National Association of Transport Companies was predicting daily losses of over BRL 1 billion (USD 184 million) across its members (Ibold et al., 2020[43]). Bailouts have been negotiated. Beyond the emergency, the crisis highlights fundamental issues in public transport funding, specifically the overreliance on fare revenues. The pandemic is the opportunity to adopt a more sustainable funding model, which properly reflects the social and environmental benefits that only public transport provides.
Reverting to pre-Covid-19 funding schemes will not be possible if the networks are to maintain an acceptable level of service. Cities are already announcing cuts to services due to the lack of fare revenues (CBC, 2020[51]; de la Garzia, 2020[37]). The current funding usually is a mix of ticket revenues, government funding, some form of taxes and other sources depending on the city. In developing countries with large shares of paratransit, these operators rely solely on fares. Transitioning from over-reliance on fares to more stable revenue sources will be necessary as public transport builds back from the crisis (McArthur and Smeds, 2020[41]). Land-value capture provides a potential mechanism. It seeks to monetise the windfall gains landowners can realise from land in proximity to newly developed public transport and to use it to pay for the network (Medda, 2012[52]; Transport for London, 2017[53]). Support from the national level for local governments to fund good public transport is another mechanism that will also benefit the economy by creating and connecting people to jobs, enabling better labour force participation (Sclar, Lönnroth and Wolmar, 2016[54]).
Cuts to public transport services now would roll back years of progress. Regaining pre-pandemic levels of public transport ridership will be difficult. If lack of funding forces operators to cut services or increase fares, this may become impossible (Steer, 2020[55]). It would undo years of progress in shifting urban mobility to sustainable transport options (McArthur and Smeds, 2020[41]). Efforts to renew public transport fleets with cleaner vehicles may be at risk, as they slip down the priorities list in the face of severely squeezed funding.
Public transport funding needs new prioritisation, not new money. Savings from building fewer new roads, car ownership and energy costs will exceed public transport investment needs (Fulton et al., 2017[22]). The patterns of public transport use will change, but its role in society remains the same: as an essential service and one of the most environmentally sustainable forms of transport (ITF, 2020[56]). It offers individuals with no access to a private vehicle the freedom to satisfy their needs. Disproportionately, regular public transport users are women, younger or older people, have lower incomes, face mobility restrictions, and come from minority backgrounds (Banister, 2019[57]).
Adapting to new travel patterns is an opportunity for better-integrated and equitable land-use and transport planning. There is much uncertainty about what travel, commuting, and urban development patterns will look like in the future. The rise of teleworking has raised concerns that commuting to central business districts will plummet. The current peak-hour, commuter-based public transport planning approach will need to adapt to remain relevant for the off-peak neighbourhood-based trips that may replace the traditional commute. Such a shift could result in a more equitable public transport system than before. An all-day public transport schedule and a system that connects neighbourhood centres would enhance public transport’s role as the backbone of a transport system that aims to provide access to opportunities for all. Often, users from more marginalised groups are off-peak users, but traditional public transport planning does not prioritise their needs (Sustainable Development Commission, 2011[10]). Serving neighbourhood based trips throughout the day would enable a worker to access a shift job at odd hours, a senior citizen to visit the doctor mid-afternoon, or a mother to run errands between her job and picking up her child without having to wait for the bus for 20 minutes.
Spatially decentralised cities could lead to increased car dependency – but not necessarily. If teleworking becomes more accepted, citizens could choose to relocate from city centres to suburbs, possibly suburbs less serviced by public transport. The result could be more reliance on cars, exacerbating by growing urban sprawl. Required office trips would be longer, and running errands in a less dense neighbourhood would necessitate car travel. That said, a decentralisation of urban areas is not incompatible per se with the goal of a sustainable city. A well-managed decentralisation process that successfully shifts certain behaviours could pave the way for an even more equitable urban transport system (Chu, 2020[58]). The city of Paris has publicly announced their goals of a “15-minute city”, which aims to give all residents access to their needs within a 15-minute walk from home (Moreno, 2020[59]; Paris en Commun, 2020[60]). The idea of the neighbourhood centre is not new, but recovery from the pandemic may be a unique opportunity to fast‑track such initiatives. Shifts in land use to create neighbourhood centres in traditionally residential areas could start supporting new businesses and services around public transport hubs that increasingly connect neighbourhoods rather than primarily shuttling people in and out of the centre. Encouraging transit-oriented development will help keep public transport the first choice for trips unsuited to walking, cycling or scootering.
Linking transport modes will boost public transport. Integrating different transport options will be crucial to increasing public transport use again. Bus and rail can provide a strong backbone, with shared mobility services and micromobility covering the first and last mile and providing affordable alternatives to public transport during times of low demand. Mobility-as-a-Service (MaaS) platforms that use digital technologies to offer integrated scheduling and ticketing across different mobility options to simplify transfers could become increasingly important.
Protecting and promoting trust in public transport will be a challenge. A Swiss study found that between 22% and 28% of people plan to use public transport and shared modes less than before the pandemic (Deloitte, 2020[61]). An Ipsos (2020[62]) poll in the People’s Republic of China found that approximately half of the respondents who had used bus and metro before the pandemic no longer do. Prioritising sanitation and protection is one way to regain some public trust in the short term (UITP, 2020[42]). In the long run, it will be crucial to maintain or improve service levels despite funding pressures and to increase the attractiveness of the public transport offer, notably through good integration with other modes.
Urban car use is recovering rapidly, at the expense of public transport. According to the study from Switzerland cited above, up to a quarter (24%) of people surveyed plan to use their private car or motorcycle more in future (Deloitte, 2020[61]). In China, where the peak of the pandemic occurred months before it reached other parts of the world, traffic in March 2020 surpassed average 2019 traffic in Beijing, Shanghai, and Guangzhou, while user numbers for metro systems were 29% to 53% below pre-Covid levels (Bloomberg News, 2020[63]). The intention to purchase new cars is also on the rise, and 77% of potential purchasers are doing so due to health concerns (Ipsos, 2020[62]). Providing public transport in the wake of the pandemic that users perceive as safe, efficient and affordable will be a key to preventing further motorisation of city traffic.
Government investment in clean technologies remains vital. Mainstreaming clean technologies and lowering entry costs for consumers requires research and development (IEA, 2020[64]). With lower private‑sector R&D budgets due to the pandemics’ impact on businesses, government stimulus packages offer a lifeline. Government spending that gives clean energy technologies a boost provides a good return on investment for taxpayers and correlates with employment growth (Calvino and Virgillito, 2018[65]; Dowd, 2017[66]). Incentives and subsidies for automotive technologies will primarily benefit higher-income consumers looking to purchase a cleaner car (PWC Strategy, 2020[67]). Adding conditions to recovery measures to encourage sales and investment in charging infrastructure and shared fleets would better align with the overall social and environmental goals of equitable mobility (Buckle et al., 2020[68]; Goetz, 2020[69]).
Cities have made a head start on infrastructure for active and micromobility – now is the time to make it permanent. Many cities had ambitious long‑term plans for active mobility pre-pandemic. Now, where they have demonstrated success during the pandemic, cities need to capitalise on the opportunity and make temporary installations permanent in order to fast-track pre-pandemic plans.
Recover, Reshape, Reshape+: Three possible futures for urban passenger transport
This section explores potential development paths for urban passenger mobility to 2050. Its projections, presented in subsequent sections, are based on three different policy scenarios: Recover, Reshape, and Reshape+. These scenarios represent increasingly ambitious efforts by policy makers to reduce CO2 emissions in cities and decarbonise urban travel.
The definition of policies within these scenarios are based on ITF research, input from experts in the form of a policy scenario survey disseminated to policy experts from all regions of the world in early 2020, and from ITF workshops held for projects under the ITF Decarbonisation Initiative in 2020. Table 3.3 details the assumed uptake of policy measures in the scenarios. All scenarios include the same baseline economic assumptions to reflect the impact of the Covid-19 pandemic: a five-year delay in GDP and trade projections compared to pre-Covid-19 levels.
The results are based on the ITF Urban Passenger Transport Model, which simulates the development of transport activity and mode shares in cities as well as emissions of transport CO2 and local pollutants in urban areas to 2050 from the base year 2015. Box 3.1 offers a detailed description of the ITF Urban Passenger Transport Model and changes to previous versions.
Box 3.1. The International Transport Forum urban passenger transport model 2020
The International Transport Forum (ITF) urban passenger transport model assesses transport supply and demand in all regions in the world. It does so for more than 9 200 macro Functional Urban Areas (FUA). It estimates trips, mode shares, passenger-kilometres, vehicle-kilometres, energy consumption and CO2, SO4, NOx and PM emissions for 18 modes1 for the period from 2015 to 2050 in five-year increments. The current version enables an assessment of the impact of 23 policy measures and technology developments which are specified for each of the 19 regional markets included in the model. The model developed at ITF was first presented in 2017 and is constantly updated and improved. Some of the key features that were updated since 2019 are described below. These changes are partially responsible for differences in model assumptions and baseline values between the 2021 and 2019 editions of the Transport Outlook.
Where available, socio-economic and mobility data, including GTFS data, have been collected for the FUAs. Where unavailable, the model replaces missing data with synthetic data estimated using regression analysis from similar FUAs. Inputs such as GDP per capita, geographic area and energy costs are updated for each model iteration.
In each iteration, the model first updates transport supply characteristics, which includes information on vehicle ownership, the availability of road infrastructure, public transport and other mobility services. Second, it generates trips. Third, a mode split module calculates mode shares using a discrete choice model that accounts for cost, time and accessibility attributes of the different modes. Last, transport emissions are estimated based on vehicle load factor and average vehicle emissions depending on the local vehicle fleet composition.
Table 3.2. Summary of urban model updates
2019 version |
2021 version |
|
---|---|---|
Urban population and cities |
3.3 billion people in 11 099 cities |
3.6 billion people in 9 234 macro Functional Urban Areas2 (FUA) (United Nations, 2019[70]; OECD/European Commission, 2020[71]) |
Demographic model |
External input |
Internal demographic urban model representing population evolution for 36 age and gender groups3 (WorldPop, 2020[72]) for each macro FUA |
Land-use evolution |
For each FUA, a growth rate is estimated. |
For each macro FUA, different growth rates are estimated for the macro FUA centre and for its suburbs |
Environmental performance |
Average tank‑to‑wheel vehicle emissions based on the ICCT Roadmap Model (ICCT, 2019[73]) for local pollutants and the IEA Mobility Model (IEA, 2020[13]) for CO2. |
Include both tank-to-wheel and well‑to‑tank CO2 emissions based on the IEA Mobility Model (IEA, 2020[13]). Includes local pollutants based on the ICCT Roadmap Model (ICCT, 2019[73]). |
Trip generation model |
Average trip rates |
Trip rate calculated based on five distances, five ages and two gender categories |
Estimation of car and motorcycle demand |
Over-estimation of car and under-estimation of motorcycle passenger kilometres particularly in Asia and Latin America and the Caribbean |
Reduction of car passenger kilometres and increase of motorcycle passenger kilometres, resulting in similar total demand but lower CO2 emissions in the related world regions. |
Walk access and egress trip legs |
Not considered |
Non-active modes include an additional walking component for access and egress. |
1. The 18 modes included in the model are: walk, bike, private motorcycle, private car, taxi, public transport (PT) rail, PT metro, PT right rail transit, PT bus rapid transit, PT bus, informal bus, informal three-wheelers, scooter sharing, bikesharing, ridesharing, motorcycle sharing, carsharing, and taxi-bus.
2. Macro FUAs are aggregations of FUAs defined by the joint EC-OECD Cities in the World project and identified in the UN DESA World Urbanization Prospect 2018 project
3. Disaggregation of the city population in 36 age and gender categories
Urban mobility in the Recover scenario
In the Recover scenario, pre-pandemic thinking in terms of policies, investment priorities and technologies shapes urban mobility in the coming decade. Governments prioritise and reinforce primarily established economic activities to buttress the recovery. The main objective is the return to a pre-pandemic “normal”. Recover is a more ambitious version of the Current Ambition scenario in the ITF Transport Outlook 2019.
The pandemic’s impact on urban travel during 2020 gradually disappears by 2030 in the Recover scenario. On the positive side, policies are implemented to ensure public transport ridership returns to earlier levels. On the negative side, climate-friendly behaviours also revert to pre-pandemic practices by 2030: the shift to active mobility which helped lower CO2 emissions during the pandemic, proves to have been temporary, for instance.
CO2 mitigation policies in place by the start of the pandemic or about to be implemented are honoured. Pre-pandemic policies to reduce private car use continue, for instance. Carbon pricing is in place for all modes and ensures that the cost of use reflects their CO2 emissions. No further efforts to decarbonise transport are made, however.
Technological progress in the Recover scenario occurs at a moderate rate. Changes in the electrification of vehicle fleets follow the Stated Policies Scenario (STEPS) of the International Energy Agency (IEA, 2020[13]).
Some cities continue to implement policies to reduce excessive car use, but change does not happen on a grand scale. Some cities and suburbs densify while others sprawl. Neighbourhoods around public transport hubs experience a modest increase in density and diversity of use. On some city streets, new bicycle and pedestrian infrastructure, speed limits, and the prioritisation of public transport help to continue a shift away from car use. Yet this remains exceptional. Some cities also restrict car use through urban vehicle restriction schemes, parking pricing and regulations, and road pricing mechanisms. Again, implementation is not widespread.
A few cities encourage low-emission vehicles through incentives and infrastructure investment. Carsharing, carpooling and shared transport modes are encouraged as alternatives to private vehicles. Public transport receives moderate investment. On average, there is little change to existing rail corridors. Bus and paratransit services improve slightly. Some cities increase their service network but do not integrate it efficiently with other modes.
Paradigm change: urban mobility in the Reshape scenario
In the Reshape scenario, the impacts of Covid-19 on urban travel also gradually disappear by 2030, as under Recover. Reshape differs in that policy makers set ambitious climate goals and implement stringent policies in their pursuit. Also, these more ambitious policies are put in place worldwide to different extents depending on the region. Reshape is a more ambitious version of the High Ambition scenario in the ITF Transport Outlook 2019.
Carbon prices are higher under Reshape than in the Recover scenario across all regions and modes.
Urban sprawl is stopped. The density of cities is maintained or increases, both in city centres and suburbs. Transit-oriented development is more pronounced than under Recover, increasing density and diversity around transport hubs.
Car travel is deprioritised. Street space in cities is reallocated away from cars more radically. Speed limits are reduced further. Dedicated lanes or signalling gives priority to at least parts of the public transport networks in all cities. Infrastructure for cyclists and pedestrians expands and improves dramatically in more cities. Urban vehicle restriction schemes, road and parking pricing and regulations reduce car use considerably more than in Recover.
Existing transport capacity is used more efficiently. Incentives for carpooling, carsharing and ridesharing have a more noticeable impact on average load factors and the availability of shared mobility.
The infrastructure for electric and other low-emission vehicles improves thanks to targeted incentives and investments, resulting in a marked reduction of average CO2 emissions in some cities. The vehicle fleet composition follows the technology evolution assumptions of the IEA Sustainable Development Scenario (SDS) (IEA, 2020[13]).
Public transport offers a highly integrated service with seamless transfers between other modes through Mobility as a Service (MaaS) applications. Paratransit services are gradually regulated and integrated with formal public transport or shared-mobility systems, resulting in cleaner fleets in developing regions.
Reshape+: Reinforcing Reshape
In the Reshape+ scenario, positive decarbonisation trends from the pandemic are locked in through policies that lead to permanent change. As in the other two scenarios, the negative impacts of Covid‑19 on urban mobility transport are overcome by 2030. As in the Reshape scenario, governments set ambitious decarbonisation targets and implement policies that can deliver them. However, governments further seize opportunities for decarbonisation that emerged during the pandemic. By aligning economic stimuli with climate and equity objectives, they leverage economic recovery for environmental and social sustainability. They do so by implementing some Reshape policies more strongly or on a faster timeframe.
More teleworking reduces the number of commuting trips while supporting economic productivity.
Transit-oriented development on a large scale fosters positive attitudes towards public transport and counteracts any potential impact from people moving away from city centres.
Bicycle and pedestrian infrastructure is widely available. Temporary “pop-up” infrastructure for active mobility initiated during the pandemic is made permanent.
Increased incentives for the purchase of low-emission vehicles are funded from stimulus packages. The benefits of Reshape are moved forward, allowing the cities to reach decarbonisation sooner and with more certainty.
Reshape and Reshape+ are optimistic scenarios that show what could be done if we harness the opportunity to transform transport in a pandemic recovery. The policies are technically feasible, but ITF recognises some constraints that may limit regions from implementing every one of the measures. ITF does not seek to be prescriptive in the combination of policies. It highlights opportunities for economic stimulus packages to prioritise the creation of equitable cities while mitigating emissions.
Table 3.3. Scenario specifications for urban passenger transport
Shading denotes policies with stronger implementation in Reshape+
Measure/Exogenous factor |
Description |
Recover |
Reshape |
Reshape+ |
---|---|---|---|---|
Economic instruments |
||||
Carbon pricing |
Pricing of carbon-based fuels based on the emissions they produce. |
Carbon pricing varies across regions: USD 150‑250 per tonne of CO2 in 2050 |
Carbon pricing varies across regions: USD 300-500 per tonne of CO2 in 2050 |
|
Road pricing |
Charges applied to motorised vehicles for the use of road infrastructure. |
0% to 7.5% increase of non-energy related car use costs by 2050, half for motorcycles. |
2.5% to 25% increase of non-energy related car use costs by 2050, half for motorcycles. |
|
Parking pricing and restrictions |
Regulations to control the availability and price of parking spaces for motorised vehicles. |
5% to 50% of a city area subject to parking constraints, and 0% to 60% increase in parking prices by 2050. |
7% to 75% of a city area subject to parking constraints and 20% to 150% increase in parking prices by 2050. |
|
Enhancement of Infrastructure |
||||
Land-use planning |
Densification of cities. |
Density variation of -10% to +20% for the city centre of urban areas over 300 000 inhabitants. Density variation of -10% to +10% for cities under 300 000 inhabitants and for suburbs of urban areas over 300 000 inhabitants. |
Density variation of 0% to +40% for the city centre of urban areas over 300 000 inhabitants. Density variation of 0% to +20% for cities under 300 000 inhabitants and for suburbs of urban areas over 300 000 inhabitants. |
|
Transit-Oriented Development (TOD) |
Increase in mixed-use development in neighbourhoods around public transport hubs. |
Increases the land-use diversity mix and increases the accessibility to public transit by 5% by 2050. |
Increases the land-use diversity mix and increases the accessibility to public transit by 7.5% by 2050. |
Increases the land-use diversity mix and increases the accessibility to public transit by 10% by 2050. |
Public transport priority measures and express lanes |
Prioritising circulation of public transport vehicles in traffic through signal priority or express lanes. |
0% to 40% of bus, light rail transit and bus rapid transit network prioritised by 2050. |
10% to 60% of surface public transport network prioritised by 2050. |
|
Public transport service improvements |
Improvements to public transport service frequency and capacity. |
-10% to +10% service improvement for rail or corridor based public transport systems resulting in a -1% to +1% speed variation by 2050. 10% to 30% service improvement for bus and paratransit transport systems resulting in a 0.25% to 0.7% speed variation by 2050. |
10% to 15% service improvement for rail or corridor based public transport systems resulting in a 1% to 1.5% speed variation by 2050. 20% to 50% service improvement for bus and informal public transport systems resulting in a 0.5% to 1.25% speed variation by 2050. |
|
Public transport infrastructure improvements |
Improvements to public transport network density and size. |
0% to 100% growth increase for the public transport network by 2050. |
0% to 200% growth increase for the public transport network by 2050. |
|
Integrated public transport ticketing |
Integration of public transport ticketing systems. |
1.5% to 4.5% reduction of a public transport ticket cost, and 2.5% to 7.5% reduction of public transport monthly subscription cost by 2050. |
1.5% to 7.5% reduction of a public transport ticket cost, and 2.5% to 12.5% of public transport monthly subscription cost by 2050. |
|
Bike and Pedestrian infrastructure improvements |
Increase in dedicated infrastructure for active mobility. |
20% to 300% increase in road space available to active modes by 2050 and a simultaneous increase in the speed of active modes, including micromobility |
40% to 500% increase in road space available to active modes by 2050 and a simultaneous increase in the speed of active modes, including micromobility. |
50% to 600% increase in road space available to active modes by 2050 and a simultaneous increase in the speed of active modes, including micromobility. |
Speed limitations |
Traffic calming measure to reduce speed and dominance of motor vehicles through low-speed zones or infrastructure. |
2% to 30% reduction of speed on main roads, by 2050 |
5% to 50% reduction of speed on main roads, by 2050 |
|
Regulatory instruments |
||||
Urban vehicle restriction scheme |
Car restriction policies in certain areas and during certain times to limit congestion. Typically applied in the city centre. |
0% to 17.5% reduction of car ownership by 2050, Reduction of the car and carsharing speeds while increasing the car and motorcycle access time. |
3.5% to 25% reduction of car ownership by 2050, Reduction of the car and carsharing speeds while increasing the car and motorcycle access time. |
|
Low-emission vehicles incentives and infrastructure investment |
Financial incentives for the purchase and use of alternative fuel vehicles and investment in charging infrastructure. |
Decreases average vehicle-kilometres made with diesel, gasoline and methane fuels between 0% and 4% by 2050. |
Decreases average vehicle-kilometres made with diesel, gasoline and methane fuels between 0% and 36% by 2050. |
Decreases average vehicle-kilometres made with diesel, gasoline and methane fuels between 0% and 45% by 2050. |
Stimulation of innovation and development |
||||
Electric/alternative fuel vehicle penetration |
Degree of uptake of electric/alternative vehicles in an urban vehicle fleet |
Follows the IEA STEPS Scenario |
Follows the IEA SDS Scenario |
|
Carsharing incentives |
Incentives to encourage car rental schemes where members have access to a pool of cars as needed, lowering car ownership |
0% to 15% increase in shared car availability per capita, and 0% to 40% increase in shared motorcycle availability per capita, by 2050. |
5% to 30% increase in shared car availability per capita, and 10% to 60% increase in shared motorcycle availability per capita, by 2050. |
|
Carpooling policies |
Carpooling policies encourage consolidating private vehicle trips with similar origins and destinations. |
3.5% to 8.3% increase in average load factor by 2050. |
7.6% to 16.7% increase in average load factor by 2050. |
|
Ridesharing/shared mobility |
Increased ridership in non-urban road transport (car & bus) |
25% to 200% increase of ridesharing vehicles per capita growth by 2050. Load factor evolution from -50% to +25% by 2050. |
25% to 300% increase of ridesharing vehicles per capita growth by 2050. Load factor increase from 0% to 100% by 2050. |
|
Mobility as a Service (MaaS) and multimodal travel services |
Improved integration between public transport and shared mobility (app integration, as well as physical infrastructure, ticketing and schedule integration). Increase in availability and load factors of shared mobility |
1.7% to 10% reduction of a public transport ticket cost, and 1.0% to 6.0% reduction of shared mobility cost by 2050. Increase in the number of shared mobility vehicles and stations |
3.3% to 20% reduction of a public transport ticket cost, and 2.0% to 12.0% reduction of shared mobility cost by 2050. Significant increase in the number of shared mobility vehicles and stations |
|
Exogenous factors |
||||
Autonomous vehicles* |
Introduction of vehicles with level 5 autonomous capabilities |
The percentage of autonomous vehicles in use varies across regions: for car 0% to 3%, for bus 0% to 1.5%, for shared vehicles 0% to 6%. |
||
Teleworking |
Reduces business and commuting trips, while increasing short non-work trips. |
2.5% to 20% of the active population could telework by 2050. |
3.5% to 30% of the active population could telework by 2050. |
5% to 40% of the active population could telework by 2050. |
Note: Range of values reflect the varying degrees of implementation of policy measures across the different world regions in each scenario. Unless otherwise specified, a % change indicates an alteration of a certain variable in a given year compared to the absence of a policy. For example, PT ticket costs are endogenously calculated for each city and year by the model, indexed to GDP, assuming no policy action. An X% decrease would be applied to the ticket price of the specific city and year.*Autonomous vehicles are considered but are not a primary factor in any of the scenarios. All scenarios assume a constant level of introduction of vehicles with Level 5 autonomy. The ITF Transport Outlook 2019 focussed more specifically on transport disruptions, including autonomous vehicles, and assessed related scenarios.
Demand for urban travel: Managing mobility in growing cities
Demand for urban mobility depends on several factors. The most significant are population size, economic activity and land-use patterns. Population growth increases total mobility volumes (measured in passenger‑kilometres), while travel per capita tends to grow as incomes increase (Rodrigue, Comtois and Slack, 2009[74]). How this travel is undertaken – by which transport mode and to which destinations – will influence total travel volumes and their associated emissions, as well as other outcomes relevant for human well‑being.
The actual distances travelled are primarily influenced by land-use patterns and the density of mixed developments. Cities, where jobs are located close to residences and commercial areas will result in fewer kilometres travelled than those with sprawling, segregated patterns of development. More transport activity, therefore, is not an indicator of greater well‑being. What influences the quality of life is accessibility, which considers individual needs, the locations of opportunities and the transport services between them. Higher transport volumes are often due to limited accessibility, which results in longer trip distances and higher costs both in terms of time and budget. It also increases CO2 emissions and air pollution.
Total urban passenger demand is projected to grow by 59% to 2030 and 163% by 2050 from the base year 2015 under the Recover scenario. This is higher than previously projected (ITF, 2019) due in part to improvements to the model (see Box 3.1.), such as a higher urban population and accounting for active access/egress components of motorised trips. These changes increase passenger-kilometres, while lower economic growth projections due to the Covid-19 pandemic and new policy commitments made by governments in the past two years reduce demand.
The increase in urban travel demand would be limited to 116% under Reshape and 104% under Reshape+, if even more ambitious policies were put in place between 2015 and 2050. A combination of shorter trips due to land-use changes and fewer work trips as a result of more teleworking are behind this result. These changes increase accessibility, well‑being and economic growth despite lower overall transport volumes. Reshape+, in particular, assumes the most ambitious land-use changes and rates of telework. Some work trips are replaced by an increase in local non-work trips, but in a well-managed land‑use scenario, they are assumed to be shorter in nature and are expected to have a net reduction on urban kilometres travelled.
Shortening travel distances is key to curb car use in cities. More than half of global urban passenger-kilometres travelled in 2015 were made with private vehicles. By 2050, however, the more ambitious policies simulated in the Reshape+ scenario could limit demand for private vehicle passenger-kilometres to one-third of 2050 global totals (Figure 3.3). Policies to limit private vehicle use and decrease car ownership achieve the most pronounced mode shift away from private vehicles. Car restriction schemes, pricing mechanisms for parking, road use and carbon, and the reallocation of road space away from cars all decrease the relative attractiveness of private car use vis-à-vis active mobility, public transport and shared mobility.
Changes in land use and transit-oriented development (TOD) allow for shorter travel distances and may determine whether citizens choose to drive or not. Private vehicles are more attractive to those with inferior alternatives and those travelling longer distances or linking several destinations.
In the Reshape+ scenario, integrated land-use planning and TOD have particularly positive results in shifting shorter trips away from private cars. For distances between one and ten kilometres, private vehicle shares are 7 to 9 percentage points lower in 2050 under the Reshape+ scenario than under the Recover scenario (Figure 3.4). Private vehicle use is replaced mainly by forms of active and micromobility for shorter distances and shared transport for longer trips.
Figure 3.3. Demand for urban passenger transport by mode to 2050
Under three scenarios, billion passenger-kilometres

Note: Figure depicts ITF modelled estimates. Recover, Reshape and Reshape+ refer to the three scenarios modelled, which represent increasingly ambitious post-pandemic policies to decarbonise transport. Active mobility and micromobility include walking, biking, scootersharing, and bikesharing. Public transport includes PT rail, metro, bus, LRT, and BRT. Paratransit includes informal buses and PT three‑wheeler. Shared vehicle includes motorcycle and carsharing. Private Vehicle includes motorcycles and cars. Shared mobility includes taxis, ridesharing, and taxi buses.
Figure 3.4. Mode shares for urban trips of different length in 2050
Under three scenarios, percentage of trips

Note: Figure depicts ITF modelled estimates. Recover and Reshape+ refer to two scenarios modelled, represent current ambitions and much increased ambitions with regard to post-pandemic policies to decarbonise transport. The third scenario modelled, Reshape, is not shown as it results in very similar shares as Reshape+. Active mobility and micromobility include walking, biking, scootersharing, and bikesharing. Public transport includes rail, metro, bus, Light Rail Transit, and Bus Rapid Transit. Paratransit includes informal buses and public transport with three‑wheelers. Shared vehicle includes motorcycle and carsharing. Private vehicles includes motorcycles and cars. Shared mobility includes taxis, ridesharing, and taxi buses.
Active modes, shared mobility and public transport gain ground in ambitious scenarios. Trips by private car primarily shift to taxi, ridesharing and taxi-bus as well as shared vehicle ownership schemes for longer distances. Shared mobility grows from 1% of passenger-kilometres in 2015 to 10% in 2050 in the Recover scenario. Shared vehicles maintain a 2% share between 2015 and 2050. Under Reshape and Reshape+, shared vehicles account for 3% of passenger-kilometres, shared mobility accounts for one-fifth of passenger-kilometres by 2050. Public transport use grows by 184% by 2050 in Recover. Its share of total demand remains steady in 2050, as more of the shorter trips use active modes, especially with more ambitious decarbonisation policies in place. Walking, cycling and micromobility increase more than 2.5‑fold and make up 18% of total passenger-kilometres by 2050 in both Reshape and Reshape+, growing from 15% in 2015.
Paratransit will likely be absorbed by shared mobility and public transport. Paratransit is informal collective transport. It dominates urban mobility in many developing countries. Under the Recover scenario, the share of paratransit grows to 13% of total passenger-kilometres by 2050. Yet in Reshape and Reshape+ it plummets to only 5%, largely due to the formalisation of paratransit options in developing nations.
Asia remains the highest generator of urban transport demand. Total urban passenger transport demand varies considerably by region but is projected to grow in all regions under all policy scenarios (Figure 3.5). Asia contributed 40% of transport activity in 2015, the largest share of all regions. Strong economic growth, rapid urbanisation and fast motorisation of China and, to a lesser extent, India drive total urban passenger activity, which triples by 2050 in the Recover scenario. Policies in line with the Reshape scenario would cut 17% of demand compared to Recover in 2050 and Reshape+, 21%.
Significant scope exists to restrain urban mobility growth in North America. The United States and Canada were responsible for 20% of the global urban passenger-kilometres in 2015 due to low-density urban developments and longer travel distances. Cities in the region are often decentralised, requiring long commutes. Reshape policies would limit the growth of travel demand in cities to 13% above 2015 levels in 2050. Under Reshape+ policies, demand growth could be frozen at close to 2015 levels. The region comprising the European Economic Area (EEA) and Turkey, as well as the Middle East and North Africa (MENA) region, also show considerable potential to limit demand growth under higher ambition policies. Compared to 2015 totals, 2050 demand growth could be 19% and 30% under Reshape policies, but 8% and 20% with a Reshape+ agenda, respectively.
Population growth and economic development drive urban mobility demand in other regions. The highest relative growth in transport activity is projected for Latin America and the Caribbean (LAC) and Sub-Saharan Africa (SSA), driven by high economic growth in LAC and significant urban population growth in SSA. Under current policies, LAC’s urban transport activity is estimated to be 3.5 times higher by 2050 than in 2015 and 6.2 times higher in SSA. Mitigation potential is more limited in the region due to financial constraints, urbanisation patterns, and rising living standards. However, Reshape+ policies would enable these regions to achieve an 18% to 25% reduction in 2050 compared to a Recover scenario. A shift to sustainable options could allow these regions to leapfrog developed countries which are locked into unsustainable transport systems based on private vehicle ownership. Under Reshape+, LAC could see growth limited to 2.7 times 2015 values by 2050 and SSA 4.9 times.
Figure 3.5. Demand for urban passenger transport by world region to 2050
Under three scenarios, billion passenger-kilometres

Note: Figure depicts ITF modelled estimates. Recover, Reshape and Reshape+ refer to the three scenarios modelled, which represent increasingly ambitious post-pandemic policies to decarbonise transport. EEA: European Economic Area. LAC: Latin America and the Caribbean. MENA: Middle East and North Africa. OECD Pacific: Australia, Japan, New Zealand, South Korea. SSA: Sub-Saharan Africa. Transition economies: Former Soviet Union and non-EU South-Eastern Europe.
Per capita, transport demand is highest in the United States and Canada. In 2015, the United States and Canada generated 2.7 times more passenger-kilometres per person on average than individuals in Asia, the region with the largest total urban passenger demand (Figure 3.6). Urban mobility per inhabitant in the OECD Pacific region (Australia, Japan, Korea and New Zealand) is also significantly higher than in Asia, by a factor of 1.7. Compared to Sub-Saharan Africa (SSA), the region with the lowest urban travel per inhabitant, the average city-dweller in the United States and Canada generates 3.8 times as much demand, and individual travel in the OECD Pacific region is 2.3 times higher. This gap will narrow by 2050, but even then, the United States and Canada still generate 2.3 times the per capita travel demand of SSA, and the OECD Pacific region 1.9 times. The United States and Canada reduce per capita demand by 21% by 2050 under Reshape+, compared to 2015. The region comprising the European Economic Area (EEA) and Turkey achieves the second-highest reduction of 13%. By 2050, most other regions generate more travel activity per capita even under Reshape+ compared with 2015 levels.
Figure 3.6. Per capita demand for urban passenger transport by world region to 2050
Under three scenarios, passenger-kilometres per capita

Note: Figure depicts ITF modelled estimates. Recover, Reshape and Reshape+ refer to the three scenarios modelled, which represent increasingly ambitious post-pandemic policies to decarbonise transport. EEA: European Economic Area. LAC: Latin America and the Caribbean. MENA: Middle East and North Africa. OECD Pacific: Australia, Japan, New Zealand, South Korea. SSA: Sub-Saharan Africa. Transition economies: Former Soviet Union and non-EU South-Eastern Europe.
CO2 emissions from urban mobility: Improved services, smaller carbon footprint
Tank-to-wheel CO2 emissions from urban passenger transport are expected to decrease by 5% between 2015 and 2050 in the Recover scenario. This represents a drop from 1 755 million tonnes of CO2 to 1 674 million tonnes. Total urban passenger CO2 emissions in the baseline year 2015 are less than estimated in the ITF Transport Outlook 2019 due to model improvements with additional calibration data (see Box 3.1). If more ambitious policies are enacted, expected emissions by 2050 could fall to 394 million tonnes in Reshape and 373 million tonnes in Reshape+, a 78% and 79% drop from 2015.
Reshape+ policies help decarbonise faster. The Reshape+ scenario frontloads transport decarbonisation measures and assumes more significant behavioural change compared to Reshape. By 2030, Reshape+ achieves a 28% decrease in CO2 emissions from urban mobility compared to 2015, while under Reshape emissions fall by 25% compared to 2050. At that stage, both achieve similar reductions from 2015 emission levels of 78% and 79% respectively. What matters when comparing with climate emission targets, is the cumulative emissions. Under the policies of a Reshape+ scenario, the sector would emit 1.25 Gigatonnes CO2 less compared to a Recover trajectory by 2050.
Increases in load factors and fuel efficiency result in lower emissions. In addition to motorised passenger demand, emissions depend on how many people share a vehicle trip, known as the vehicle load factor, and the fuel efficiency of the vehicle. The preceding section describes the projected growth in demand from 2015 to 2050. In Recover, motorised travel holds 87% of the passenger-kilometre share by 2050, while in Reshape and Reshape+ it is responsible for 82% of travel thanks to mode shift to active modes. Figure 3.7 shows the CO2 emissions generated by mode for each scenario. In Recover, vehicle efficiency improves so that, on average, vehicles emit 57% less CO2 in 2050 compared to 2015, over the same distance. In Reshape and Reshape+, emissions per vehicle-kilometre are 86% lower in 2050 than in 2015. In addition, measures to increase vehicle load factors by shifting to mass and well-integrated shared transport, and carpooling incentives, mean that average vehicle load factors are 22% higher in 2050 than in 2015 in the Recover scenario, and 28% to 29% higher in the more ambitious scenarios. Therefore, CO2 emissions generated per passenger-kilometre drop by 65% by 2050 in Recover and by 89% in Reshape and Reshape+.
Emissions from private vehicles in cities can be more than halved. In 2015, emissions from private vehicle use made up three-quarters of urban passenger emissions. The share drops to 50% in Recover, primarily because of technological improvements and mode shift. In Reshape and Reshape+, they drop 56% and 57% by 2050 thanks to more pronounced mode shift, higher load factors and more ambitious expectations of new technology penetration in the vehicle fleet.
Well-integrated shared mobility is much less emitting. Most motorised modest reduce emissions by 2050 compared to 2015, in all scenarios. Shared mobility and paratransit are exceptions. The market penetration of shared mobility is very low in 2015, and as it gains mode share, its emissions appear to grow. With only minimal integration and management of shared mobility services in the Recover scenario, shared mobility emissions increase ten-fold between 2015 and 2050. However, in scenarios where shared mobility is well-managed and fully integrated into the transport system, its emissions grow only a little more than half as much (57% and 55% respectively for the Reshape and Reshape+ scenarios compared to the Recover outcome). Paratransit under a Recover scenario also emits more due to demand growth but fall under the more ambitious policies as these informal services are integrated into the official networks.
Shared vehicles and shared mobility allow faster adoption of clean technologies. Both have higher utilisation than a typical private car, and vehicles thus need to be replaced more often. In a well-integrated system, shared mobility fills gaps in the public transport network and augments the overall offer. Swaying users to give up private cars for shared mobility requires integrated fares, routing and schedules with existing public transport via mobile phone applications. The targeted reconfiguration of urban space to make transfers seamless will also help considerably. Its potential to offer a sustainable travel alternative depends on how well it is integrated with public transport, acting as a complement to, rather than replacement of, public transport. A poorly managed system that leads to the substitution of public transport could easily have the reverse effect on emissions as seen by the higher 2050 emissions by shared mobility in Recover. Box 3.2 indicates some of the factors which can contribute to having higher or lower GHG emissions from shared and micromobility services.
Figure 3.7. CO2 emissions from urban passenger transport by mode to 2050
Under three scenarios, million tonnes CO2 direct emissions (tank-to-wheel)

Note: Figure depicts ITF modelled estimates. Recover, Reshape and Reshape+ refer to the three scenarios modelled, which represent increasingly ambitious post-pandemic policies to decarbonise transport. Active mobility and micromobility include walking, biking, scooter sharing, and bike sharing. Public transport includes rail, metro, bus, Light Rail Transit, and Bus Rapid Transit. Paratransit includes informal buses and public transport with three-wheelers. Shared vehicle includes motorcycle and carsharing. Private vehicles includes motorcycles and cars. Shared mobility includes taxis, ridesharing, and taxi buses.
Box 3.2. Lifecycle impacts of micromobility
The International Transport Forum (ITF) (2020[56]) report Good to Go? Assessing the Environmental Performance of New Mobility assessed energy and greenhouse gas (GHG) emission impacts of new mobility forms, including personal and shared electric kick-scooters, bicycles, e-bikes, electric mopeds and ridesourcing, i.e. for-hire vehicle services with drivers that use smartphone apps to connect drivers with passengers.
Key findings indicate that energy use and GHG emissions from shared are comparable in magnitude to those of metros and buses if lifetime mileage of micromobility vehicles is sufficiently high and if energy use and GHG emissions from operational services are effectively minimised.
The report also highlights that, unless ridership is increased, empty vehicle travel is reduced and vehicles are switched to energy and GHG emission saving technologies, ridesourcing (like taxis) has the highest energy and GHG emission impacts per passenger kilometre of all urban mobility options.
To ensure that the deployment of new mobility comes with net benefits for transport decarbonisation, the report recommends the following solutions:
Maximise ridership, minimise deadheading and transition towards energy-efficient and low‑emission vehicles for ridesourcing (along with taxis).
Get this started from vehicles with high lifetime mileage, not just because of the highest impacts, but also because of better economics and positive spillovers to scale up and reduce costs for technologies that have a major role to play to decarbonise transport and diversify its energy mix, like electric vehicles.
Ensure greater transparency and access to info allowing the assessment of life-cycle impacts of micromobility.
Adopt sound design and operational practices to service micromobility vehicles.
Seize opportunities to help decarbonise transport from a better integration of public transport and shared micromobility (including through urban planning and Mobility as a Service).
Well-to-tank (WTT) emissions make up a larger portion of the total vehicle emissions as vehicles transition to alternative fuels. Even vehicles with low- or zero tailpipe, or tank-to-wheel, emissions cause indirect WTT emissions upstream during the production, processing and delivery of fuel. As the vehicle fleet’s direct CO2 emissions fall, the share of CO2 emitted from well-to-tank increases. In 2015, one quarter (23%) of total urban transport emissions were indirect tailpipe emissions. By 2050, their share could increase to more than one-third (36%) under Recover, and to almost half (45%) under the more ambitious scenarios. If electric mobility gains ground, indirect emissions depend on how clean or dirty the electricity grid in a region or country is. Thus, shifting to alternative fuels like electricity is not a panacea to reach climate goals. A green vehicle fleet by definition requires clean energy production, and the transport and energy sectors need to work together to achieve this. Figure 3.7 presents the simulation results for the direct tank‑to‑wheel emissions across the three scenarios. These do not include the energy for generating electricity, extracting fuels or transporting them. Figure 3.8 illustrates the split between indirect well‑to‑tank and tank‑to‑wheel emissions.
Figure 3.8. Evolution of tank-to-wheel vs. well-to-tank CO2 emissions from urban passenger transport to 2050
Under three scenarios, million tonnes CO2 emissions

Note: Figure depicts ITF modelled estimates. Recover, Reshape and Reshape+ refer to the three scenarios modelled, which represent increasingly ambitious post-pandemic policies to decarbonise transport. Tank-to-wheel emissions are emissions produced by using a vehicle (i.e. from the vehicle fuel consumption). Well-to-tank emissions are created during energy production. For instance, well-to-tank emissions for electric vehicles includes the emissions produced during electricity production, while tank-to-wheel emissions are null.
Reducing regional emissions requires a two-fold strategy. A look at the differences in urban emissions between different world regions by mode reveals two patterns. Developed regions will reduce urban passenger emissions even under a Recover trajectory, albeit not sufficiently to reach their climate objectives. Developing regions, on the other hand, would increase CO2 emissions by 2050 if action is not taken, due to fast-growing populations and economies.
Nearly half of the world’s urban passenger transport emissions came from the United States and Canada in 2015 (Figure 3.9). However, they could achieve a more than 90% reduction by 2050 under Reshape and Reshape+ policies. These would cut more than 730 million tonnes of CO2 in 2050, the largest absolute reduction of any region. In terms of the breakdown of emissions, those from private vehicles are expected to dominate under any scenario due to high car dependence in both countries.
Asia had the world’s second-highest urban transport emissions in 2015. While Asia generated more demand for mobility in cities than the United States and Canada, the related CO2 emissions were less than half the share of the North American countries, with 20% compared to 45%. Citizens in Asia use more active travel and micromobility to get around, as well as shared and public transport options. Not least, Asia has a high proportion of relatively low-emitting motorised two- and three-wheelers making up their private vehicle fleet, in contrast with the heavier vehicles in the United States and Canada.
The largest relative reduction in CO2 emissions under current policies to 2050 would happen in the EEA and Turkey, based on the Recover scenario assumptions. Under Reshape and Reshape+, the European Economic Area (EEA) and Turkey would generate the least CO2 of all world regions, with emissions 95% to 96% lower in 2050 than in 2015. The United States and Canada region and the OECD Pacific are also the only other parts of the world projected to decrease emissions even under a Recover policy agenda.
Sub-Saharan Africa will see the most substantial increase in urban emissions over the coming decades. Motorised travel demand there is projected to increase six-fold by 2050 in the Recover scenario as a result of rapidly-growing urban populations and economies. As a consequence, regional CO2 emissions from urban transport would be about five times 2015 levels. Reshape and Reshape+ policies would sharply reverse the trend and reduce emissions by 87% compared to Recover in 2050. LAC, MENA, Asia and Transition countries could also see significantly different decarbonisation outcomes depending on policies. Transition economies include countries of the former Soviet Union and non-EU south-eastern Europe. Under a Recover scenario, these regions will increase CO2 emissions by 2050, but with policies closer to a Reshape+ scenario, emissions could be 82% to 90% less than the Recover outcome in 2050. Asia could reduce emissions by more than 230 million tonnes CO2 in 2050 under Reshape and Reshape+ policies.
As shared mobility gains a greater mode share in Reshape and Reshape+, its share of urban emissions increase. This is the result of the desired effect, as shared mobility gains popularity when users shift from private car use to a shared system. It is particularly responsible for decreasing the share of private car emissions EEA and Turkey, OECD Pacific, and Transition countries.
The formalisation of paratransit helps drive urban emissions down in some world regions under the Reshape and Reshape+ scenarios. The LAC region, in particular, sees an almost complete formalisation of paratransit, as well as a shift to shared mobility, significantly decreasing emissions. Formalisation allows regulation of vehicle standards and the adoption of cleaner fleets which can successfully decarbonise the sector. Aside from the environmental benefits, formalisation raises some equity considerations that must be taken into account and are discussed later in the chapter.
Figure 3.9. CO2 emissions from urban passenger transport by world region in 2050
Under three scenarios, million tonnes CO2 direct emissions (tank-to-wheel)

Note: Figure depicts ITF modelled estimates. Recover, Reshape and Reshape+ refer to the three scenarios modelled, which represent increasingly ambitious post-pandemic policies to decarbonise transport. EEA: European Economic Area. LAC: Latin America and the Caribbean. MENA: Middle East and North Africa. OECD Pacific: Australia, Japan, New Zealand, South Korea. SSA: Sub-Saharan Africa. Transition economies: Former Soviet Union and non-EU South-Eastern Europe.
The United States and Canada could achieve big absolute urban emissions cuts per capita. All world regions reduce their per capita urban transport emissions between 2015 and 2050, under all scenarios (Figure 3.10). The emissions per person reveal a striking contrast between the United States and Canada as a region and the other world regions. The average city-dweller there generated 19 times as much CO2 in 2015 from moving around cities as did the average individual in a city in Asia, which had the second-highest total emissions in that year. By 2050, Asian emission will have grown, but the United States and Canada region will still generate 12 times as much CO2 per person from urban transport. That said, the reduction is still massive and represents the largest absolute drop in per-capita emissions of any region at 2 500 kg per person.
Ambitious policies could reduce per capita emissions by more than 90% in some regions by 2050, notably the EEA and Turkey region, followed by the United States and Canada and OECD Pacific. Under Reshape+ policies, the EEA and Turkey region could reduce its per capita emissions to the lowest of the world regions. The SSA region has the lowest urban transport emissions per capita and will likely also reduce them the least – but it could still eliminate two-thirds of them by 2050.
Figure 3.10. Per capita CO2 emissions from urban passenger transport by world region in 2050
Under three scenarios, kilograms direct CO2 emissions per capita (tank-to-wheel)

Note: Figure depicts ITF modelled estimates. Recover, Reshape and Reshape+ refer to the three scenarios modelled, which represent increasingly ambitious post-pandemic policies to decarbonise transport. EEA: European Economic Area. LAC: Latin America and the Caribbean. MENA: Middle East and North Africa. OECD Pacific: Australia, Japan, New Zealand, South Korea. SSA: Sub-Saharan Africa. Transition economies: Former Soviet Union and non-EU South-Eastern Europe.
Motorised transport is a significant source of local pollutants from fuel exhaust and non-exhaust mechanisms, such as brake, tyre and road wear. Urban transport contributes to the emission of nitrogen oxides (NOx), sulphates (SO4), and particulate matter of 2.5 microns or less (PM2.5). Local pollutants have acute negative health impacts. Cities can capitalise on synergies between reducing CO2 and improving air quality to combat these.
Air pollution has a massive health impact and a massively unequal one. In 2016, 4.2 million premature deaths due to cardiovascular disease, respiratory disease and cancers resulted from exposure to PM2.5. Of these premature deaths, approximately 91% were in developing countries (WHO, 2018[75]), exposing a glaring global inequality. Transport has a co-responsibility to address this issue, as one contributor to ambient air pollution along with power generation, waste management and industry.
Air pollution from transport is most serious in cities. The concentration of people exposed to elevated pollution levels and concentration of the pollution sources themselves is high (Slovic et al., 2016[76]). Communities with higher proportions of ethnic minorities, children and lower incomes are exposed to substantially more air pollution than white and wealthier cohorts of the population (Reichmuth, 2019[77]; Barnes, Chatterton and Longhurst, 2019[78]). This is the case even within cities in developed nations because poorer communities everywhere in the world tend to be found next to large motorways and other polluters.
Fuel technology is a major determinant for emissions of both CO2 and local pollutants. In the recent past, a preference for diesel cars in some regions helped to reduce CO2 emissions but also raised concerns over urban air pollution. Reducing the consumption of fossil fuels through low-carbon alternatives in transport also reduces exhaust-based pollutants. However, that cannot be the extent of change. PM2.5 is also derived from non-exhaust sources such as brake, tyre and road wear (Panko et al., 2019[79]; Amato et al., 2014[80]). Vehicle weight is a significant factor in determining the level of such emissions levels. As electric vehicles are typically heavier than traditional cars, their benefits with regard to reducing non‑exhaust particulate matter appear to be negligible (Soret, Guevara and Baldasano, 2014[81]). Figure 3.11 shows the pollutant emission results for NOx, PM2.5 and SO4, by world region. The MENA region has the highest levels of PM2.5 and SO4 emissions and is exceeded by LAC for NOx emissions. Projections from all future scenarios estimate dramatic drops due to newer fleets and reductions in motorised traffic share. Under Reshape+, the EEA and Turkey region achieves the largest improvement in NOx, PM2.5 and SO4 levels, dropping to 7%, 5% and 12%, respectively, of 2015 levels by 2050.
Some of the most significant improvements in air quality in LAC and SSA are due to the formalisation of paratransit services in the more ambitious scenarios. Formalisation allows for closer regulation of vehicle fleets. In Bogota, it led to the introduction of newer technologies which reduced pollutant emissions by 40% overall. The difference is most apparent in low-income neighbourhoods, which suffer from the worst air quality and are particularly reliant on paratransit (Bocarejo and Urrego, 2020[82]).
The averages for pollutant emissions presented in Figure 3.11 do not provide a complete picture of the exposure for individuals on the ground. Exposure risk is very localised and can vary drastically even within a city. More detailed in-situ evaluations are needed to determine the impacts and potential of individual interventions. Furthermore, the actual health impacts of local pollutant exposure depend on several factors, including geography and climate, which are not considered here.
Figure 3.11. Pollutant emissions from urban passenger transport by world region to 2050
Under three scenarios

Note: Figure depicts ITF modelled estimates. Recover, Reshape and Reshape+ refer to the three scenarios modelled, which represent increasingly ambitious post-pandemic policies to decarbonise transport. EEA: European Economic Area. LAC: Latin America and the Caribbean. MENA: Middle East and North Africa. OECD Pacific: Australia, Japan, New Zealand, South Korea. SSA: Sub-Saharan Africa. Transition economies: Former Soviet Union and non-EU South-Eastern Europe.
Equity and well‑being: Accessible cities and resilient networks
This section attempts to answer the following questions: How do transport decarbonisation policies affect accessibility and well‑being? How does shifting away from private vehicles and improving public transport and shared mobility affect equity? How do land use and prioritisation of urban space affect different groups? And, how resilient are low-emission transport systems?
Measures to decarbonise transport should not undermine equity objectives. It is vital to align decarbonisation with well‑being to ensure fairness while improving access for those whose needs have been historically neglected. Highly ambitious policies will only be acceptable to the public if they are perceived to improve quality of life, not hinder it. Policy makers will also need to consider how equitably costs and benefits of these measures are distributed across different socioeconomic groups.
Urban transport systems are inextricably linked to human well‑being and social equity. Economically disadvantaged groups also face transport inequalities and poor access. By increasing access to opportunities – goods, services and people – transport services can increase social and economic well‑being (OECD, 2019[8]). For instance, studies have shown that increasing access to public transport for lower-income communities could increase their access to formal job opportunities in Latin America (Moreno-Monroy, 2016[83]), Asia and the Pacific (Baker and Gadgil, 2017[84]) and Africa (Chen et al., 2017[85]).
Ambitious decarbonisation and accessibility for all
Improving access sustainably means improving the accessibility and quality of public transport and sustainable modes while shifting users away from less sustainable options. In its broadest sense, this means prioritising public transport and active mobility improvements while disincentivising car use. The goal is to provide more affordable, lower emission and less space-consuming ways to travel that do not come at the expense of accessibility and, therefore, well‑being.
There are several ways to measure accessibility. Typically accessibility indicators take into account travel times or distances between locations representing desired opportunities. The ITF Urban Passenger Transport Model calculates a simplified measure representing the average time it would take to reach a city's edge from its centre, both by car and by public transport. A lower travel time indicates greater access opportunities. The indicator is very simplified and does not take into account the actual spatial distribution of people and opportunities (ITF, 2019[86]; Geurs and van Wee, 2004[87]). However, it helps provide a global indicator comparing the evolution of access between car and public transport in cities.
Public transport becomes more competitive vis-à-vis cars as an access provider under Reshape+ policies. Public transport generally costs less than private cars, providing a more affordable mode for all users. However, it can be less attractive due to generally higher travel times than private vehicles, among other reasons. Figure 3.12 plots the improvement in accessibility, or the reduction in average travel time, by car and public transport in 2050 for all world regions under a Reshape+ scenario compared to Recover. Points above the dotted line indicate that travel times improve more for public transport than for cars. The figure shows that in most world regions, under Reshape+, public transport travel times improve more than car travel times. Travel times by car generally still improve under higher ambition policies, albeit at a lower pace than for public transport, because of reductions in private car use and therefore congestion. The EEA and Turkey region is the exception; here travel times by car worsen, and public transport accessibility remains unchanged.
Developing countries show high gains in access by public transport under Reshape+ policies. Policies to increase public transport investments have a greater impact on accessibility in developing countries. In the Asia, MENA, LAC, SSA and Transition countries, travel times by public transport are between 17% and 21% lower under Reshape+ than under Recover. In developed countries, improvements are more modest. In European cities, low time improvements could be due to an already high provision of public transport infrastructure. In cities in the United States and Canada, on the contrary, low changes could indicate the large travel distances, which hamper efforts to increase public transport use beyond the values considered for the scenario. These changes in the relative accessibility of public transport and private vehicles are partially due to pricing mechanisms to disincentivise private vehicle use and simultaneous improvements to public transport. Equity considerations of both are discussed in the following subsections.
Figure 3.12. Potential accessibility improvements for public transport and car travel in different world regions by 2050
Difference between the average travel time from the centre to the edge of a city, for cars and public transport, in the Reshape+ scenario compared to Recover scenario

Note: Figure depicts ITF modelled estimates. Recover and Reshape+ refer to two scenarios modelled, representing current ambitions and much increased ambitions with regard to post-pandemic policies to decarbonise transport. Accessibility is represented by the average time required to travel the radius of an urban area. Improvement in accessibility (or travel time) is the difference between values under a Reshape+ scenario and a Recover scenario. Values are averaged across urban areas in a region. EEA: European Economic Area. LAC: Latin America and the Caribbean. MENA: Middle East and North Africa. OECD Pacific: Australia, Japan, New Zealand, South Korea. SSA: Sub-Saharan Africa. Transition economies: Former Soviet Union and non-EU South-Eastern Europe.
The cost of car use is artificially low. Pricing schemes can internalise the negative social, economic and environmental externalities from driving cars (Litman, 2020[88]) and transfer the cost to the drivers if implemented in the right way. Pricing policies could encourage shifting trips to more sustainable travel options as well as to non-peak hours. Distributional impacts of these measures depend on the socio-spatial characteristics of cities, the travel behaviours of residents, and how obtained funds would be used (Taylor, 2010[89]). Measures will need to balance effectiveness in mode shift and time of travel with potential negative equity impacts. In lower-income areas with low public transport availability, measures can force lower‑income citizens to not use private vehicles, but also decrease access to opportunities (Di Ciommo and Lucas, 2014[90]). Schemes that determine pricing tiers based on income levels could be a more equitable solution. However, this may limit the effectiveness of reducing car use in areas where these users make up the majority (TransForm, 2019[91]).
Policy should focus on providing alternatives to cars and not reducing access for disadvantaged groups. In regions with poor public transport, private vehicles can be the main, or even the only, means for accessing essential opportunities. This disproportionately affects lower-income groups, who are forced to maintain a private vehicle, although the high costs limit their budget for other essentials, such as housing or health care (Mattioli, 2017[92]). The only way to change this reality is to combine disincentives for private vehicle use with measures that improve access to opportunities by sustainable modes. Simply putting an additional price on car use without offering alternative transport options is likely to run into opposition, as middle- and low-income groups see their access reduced. In contrast, higher-income groups enjoy congestion-free roads and shorter travel time. Good public transport and shared mobility alternatives as a complement to pricing measures, on the other hand, could distribute the benefits across income groups (Crozet and Mercier, 2018[93]).
Improving public transport is key to affordable, sustainable access for all. Tackling transport inequalities means improving access to opportunities for marginalised groups. Often, this requires extending high-quality public transport services towards peripheral areas of cities. A recent ITF study analysed differences in access to opportunities between urban centres and wider commuting zones for 121 European cities, for different modes. It found that access by public transport is lower in the wider commuting zone of European cities than in their urban centres. Yet, these are the areas with the largest populations of lower-income households. In the twelve worst-performing cities, less than 20% of people living in city peripheries have public transport services close by. Infrastructure investments could help provide faster and more reliable access to the city (ITF, 2019[94]).
Affordability of public transport is a core component of accessibility. Spatial proximity to public transport is useless unless users have the means to use the services. In Bogota, Colombia, access to opportunities can decrease by up to 54% when considering transport fares and budgets of lower-income households (Peralta Quiros and Rodríguez Hernández, 2016[95]). Many governments provide subsidies to users to prevent barriers for lower-income groups (Li, 2019[96]). Targeted subsidies based on income, household status, and other socio-economic criteria often provide the best balance between the system's affordability and financial sustainability. Granting general subsidies based on age, for example, are not always an indicator of financial need. However, subsidies for students, for example, can have other benefits, such as helping to establish more sustainable transport behaviour from a young age.
Improvements in technology, such as smart cards and data management tools, improve targeting vulnerable users. In Colombia’s capital, Bogota, local authorities started granting fare subsidies to public transport users based on data derived from the System for Selecting Beneficiaries of Social Spending (SISBEN). The SISBEN is a stratification instrument already in use for water, electricity and health care subsidies. Through this particular scheme, SISBEN beneficiaries in Bogota increased their monthly trips by more than 50% compared to non-beneficiary users (Peralta Quiros and Rodríguez Hernández, 2016[95]). The subsidy has also increased access to opportunities for citizens living on urban peripheries and thus contributed to reducing spatial inequalities (Guzman and Oviedo, 2018[97]).
Service quality improvements help to improve access. Increases in capacity, reliability and service hours would make public transport more convenient and attractive for all users, especially those who rely solely on the system. Beyond these general improvements, tailored measures can enhance levels of public transport use and satisfaction for specific groups whose needs are generally ignored or less considered by universal policies (van Lierop and El-Geneidy, 2016[98]). For instance, measures specifically targeting safety and safety perceptions would be crucial for increasing patronage among women (Shibata, 2020[99]; Badiora, Wojuade and Adeyemi, 2020[100]; Chant and Mcllwaine, 2016[101]).
Integrated shared mobility could boost sustainable mobility for all. To harness the environmental and social benefits from shared mobility, services should be integrated with existing public transport in terms of infrastructure, service schedules, ticketing and fares. Mobility as a Service (MaaS) applications could facilitate integration. Currently, however, no examples exist where MaaS best practices have been implemented. The highest societal benefits will come from having a regulatory framework that aligns policies on pricing, land use and infrastructure design, as well as for allocating concessions and overseeing activities (ITF, 2018[102]). Particular attention needs to be paid to the role of MaaS in improving the mobility of disadvantaged groups, and how services could be designed to specifically respond to those needs (Pangbourne et al., 2020[103]).
Shared mobility can provide last-mile solutions that enhance accessibility in lower-density urban and suburban areas
Shared mobility can better connect the outskirts of cities. Vanpooling services could be the most cost‑efficient way to link peripheral areas to major public transport stations. ITF simulations found that in Lyon, France, a system with integrated van-based ridesharing could double the area with good access to employment opportunities. Accessibility gains are most noticeable in the periphery of the city (ITF, 2020[25]). Forms of shared micromobility could enhance access in denser urban areas and, to a certain extent, lower-density suburban areas by providing last-mile solutions. In Chicago and Philadelphia, well‑planned bikesharing services enhance access to employment opportunities for lower-income communities to a higher degree than for other income groups (Qian and Niemeier, 2019[104]).
Shared mobility and micromobility solutions can only be equitable if lower-income groups can afford to use them. In San Francisco, dockless bikesharing services provide better access for lower‑income neighbourhoods than dock-based services due to a larger service area and frequent repositioning practices (Qian, Jaller and Niemeier, 2020[105]). City authorities will need to ensure interventions do not overlook these areas. For instance, in Denver, the Department of Public Works requires car-share companies to have infrastructure in “opportunity areas”, i.e. where at least 30% of the population lives in poverty (Kodransky and Lewenstein, 2014[106])
Lack of access to the internet, smartphones and online payment services can limit access to shared mobility. Mobile phone penetration is around 90% in both developing and developed countries (Deloitte, 2019[107]). Yet individual characteristics such as gender, employment, literacy or age can negatively affect people’s access to smartphones (ITU and UNESCO, 2019[108]). Other barriers exist for mobile payments. In the United States, 17 million people are unbanked, equalling one in twelve households (Kodransky and Lewenstein, 2014[106]). Shared mobility services will need to take these inequalities into account to leave no citizen behind (Cohen and Shirazi, 2017[109]).
Affordability of shared mobility services is a concern for operators, as it needs to be for authorities. In most countries, it is private initiatives that have created new forms of app-based shared mobility. These services require high initial capital investments, and their digital payment systems have high transaction costs. Because of this, many business models target higher-income segments for these new services, especially in developing countries. Extending the benefits of shared mobility in these conditions towards lower‑income groups can be challenging for private operators, despite their environmental and equity benefits. In Mexico City, Jetty, a ridesharing startup, tried to offer its services to lower-income groups, reaching beyond their usual mid-to-high income market. They sought to decrease prices to bring them closer to MXN 5 (USD 0.23), the average cost of a bus ride in the city. One of the difficulties of implementation was the high cost of electronic payment commissions (Flores, 2020[110]). When individual transactions are very small, the commission eats into profits quite substantially. Developing new business models that address these difficulties by adapting to user income characteristics and needs is part of the solution (Wiprächtiger et al., 2019[111]). Given their potential accessibility and environmental benefits, increasing collaboration with public authorities for the expansion of these services towards lower income segments could be beneficial.
New regulatory frameworks could boost the affordability of shared mobility services for users. Shared mobility services could enhance access to opportunities in underserved areas where traditional transport offer has limited reach. In such cases, this raises the question of whether certain services, such as vansharing, could benefit from public transport-exclusive subsidies (ITF, 2019[112]). This would require brokering agreements with private operators, and in many cases, broadening the legal definition of which services can receive subsidies. Shared mobility services in many countries are not yet regulated or fall into grey legal areas. Relevant authorities will need to work together with shared mobility operators to develop new frameworks and regulations if they are to be part of a multi-modal, affordable and sustainable transport offer. These relationships will be essential during recovery from the Covid-19 pandemic.
Paratransit services provide valuable connectivity to lower-income groups in peripheral areas, especially in developing countries (IDB and ITF, 2020[113]). They also pose regulatory challenges. Paratransit services operate under different frameworks from those for official public transport systems. Some operate outside any regulatory supervision, some under unclear rules agreed formally or informally with the authorities (Salazar Ferro, 2015[23]). Integrating informal paratransit into regulated shared mobility can reap some of the largest decarbonisation reductions from shared mobility, according to ITF modelling results. Examples show that such a process brings other benefits, such as increased service quality standards, improved road safety and air quality (Bocarejo and Urrego, 2020[82]). It can also make mobility more affordable if tariff integration and subsidies are part of the formalisation process (Salazar Ferro, 2015[23]; Bocarejo and Urrego, 2020[114]). Without this, travel costs may go up (Bocarejo and Urrego, 2020[82]). Tensions may arise when moving from cash to digital fare systems: digital payment systems charge high commissions; also drivers may perceive payments to be delayed and feel they are less in control (Flores, 2020[110]).
Urban densification in pursuit of shorter travel distances must not extend to the point of overcrowding. Whether land-use policies and transit-oriented development will create more healthy, sustainable and equitable neighbourhoods depends mainly on two factors: the population density and the liveability and affordability of housing units in these neighbourhoods. Density and diverse land-uses mean short distances and the potential for less carbon emission from mobility. It can also make public transport more efficient. When density turns into overcrowding, however, the result can be detrimental to health and the quality of urban life in general. The Covid-19 pandemic was associated with a rapid spread of the Coronavirus around overcrowded lower-income neighbourhoods. This is partly linked to lower quality living conditions, making it harder for people to take precautionary measures. High rent prices also contribute to high concentrations of people in smaller spaces. Affordable and decent quality housing is a vital antidote to overcrowding.
Unbridled transit-oriented development can make housing less affordable. Proximity to good public transport can raise rents and land value in the neighbourhoods where investments occur. Gentrification may displace less well-off citizens to parts of the city with poorer service and less access. Investments in public transport might not serve the residents of an area targeted by transit-oriented development unless displacements are prevented. It is vital to support existing residents by ensuring rent-controlled and mixed-income housing in these developments. Working with local residents during the planning process will help.
Less road space for cars makes cities safer and fairer. Much of urban space is devoted to cars. Prioritising cars on city streets unfairly favours drivers and limits other traffic participants in utilising street space for their own travel needs. The users of more sustainable modes are more likely to be young people or seniors, women, earn lower incomes and come from ethnic minorities. There is also a significant opportunity cost linked to the excessive allocation of road space for cars instead of urban amenities and housing developments that benefit a greater portion of society. This is particularly true in cities where urban land and affordable housing is increasingly scarce.
Allocating road space to sustainable mobility has significant social benefits, particularly by increasing road safety. The Reshape and Reshape+ scenarios integrate measures that seek to enlarge road space allocated for sustainable mobility to increase the mode share of these modes. These include lengthening of priority lanes for public transport and extensions and widening of pedestrian roads and cycling lanes. Studies show that driving cars and motorcycles in urban areas is associated with, respectively, a three and eleven times higher fatality risk than riding a bicycle (ITF, 2020[115]).
Active mobility users will continue sharing road space with heavy vehicles, even with mode shift. Almost 40% of the world’s population will be either children below the age of 15 or elderly citizens over 65 years of age by 2050. Active mobility or micromobility offers these and other groups independence and an affordable travel option. Guaranteeing safe trips for them will not least depend on the availability of safe, protected infrastructure for pedestrians, cyclists and users of micromobility. Lowering speed limits will also be essential for increasing safety in urban areas (Box 3.3).
Box 3.3. Best practice for urban road safety
Road safety has become a priority in cities that aim to become more liveable. Reducing the risks of urban traffic not only saves lives, it makes people feel safer and enables a shift towards walking and cycling. Such sustainable forms of transport reduce pollution, congestion and public health issues. Safety is an essential part of sustainable urban mobility plans.
One should learn from individual cities that have achieved large reductions in road casualties. In Best Practice for Urban Road Safety, the ITF (2020[116]) provides examples of relevant policies. They include developing reliable traffic injury data, enforcing speed limits, implementing safer street design, and predicting and preventing road crashes.
London, one of the cities showcased in the report, aims to eliminate fatal and serious traffic injuries by 2041. Reaching this goal is facilitated by the Mayor’s Transport Strategy which includes the reallocation of street space towards people walking and cycling, a policy which results in lower car use. This policy thus reduces greenhouse gas emissions. It also reduces local air pollution and tackles an obesity epidemic, two issues that affect deprived communities the most. By reducing car use, the strategy gives priority to the most efficient uses of public space – walking, cycling and public transport – thereby enabling the city to envisage growth without gridlock. Giving priority to more affordable means of transport also makes for a more inclusive city. Last, reducing car use makes the streets safer, in turn enabling a further shift towards active travel, closing a virtuous circle and accelerating change.
Another city featured in the report is Fortaleza, one of the very few cities which have cut by half the number of road deaths in the last decade. The Brazilian city expanded its cycling and bus priority networks, invested in traffic calming, redesigned pedestrian crossings and lowered speed limits on arterials. Such measures address road danger and reduce car dependence at the same time.
Gender shapes travel patterns; it should also shape transport planning. Gender heavily influences the way people travel. The types of jobs undertaken by women in the workforce are less likely to involve typical commutes. Women are overrepresented in the service and care industries, for instance, and also assume more roles within the household than men. Their trip patterns are thus typically more complicated, chaining together multiple trip purposes and destinations. Women tend to travel shorter distances, perform more inter-modal trips, combine several modes in one journey, and travel at off-peak hours. They also tend to use active mobility, generally walking (Miralles-Guasch, Melo and Marquet, 2015[117]). Thus, women tend to value public transport services’ reliability higher than men. This highlights the importance of transport service resilience from a gender perspective (Ng and Acker, 2018[118]; ITF, 2019[119]). The same is true for safety. Women also tend to face higher risks in public spaces than men, despite having higher walking shares. This is especially true in developing countries, making active mobility less safe for women than men (Chant and Mcllwaine, 2016[101]).
A gender-based approach to transport policies can contribute to adapting public spaces and infrastructure to serve the mobility patterns and needs of women. Mode share inequalities can be higher depending on the mode, income segment and area of the world (Gauvin et al., 2020[120]). In Latin American cities, at most, 30% of cycling trips are done by women, while in some European urban areas female users have a higher cycling mode share than men (Montoya-Robledo et al., 2020[121]). Even in urban areas with a higher female cycling share, there can be difficulties for women to use active mobility infrastructure because they have been developed without considering the needs of female users. One potential barrier, for example, could be the lack of cycling infrastructure allowing to carry a child along with the main rider (Montoya-Robledo et al., 2020[121]).
The higher the decarbonisation policy ambition, the higher the resilience of the system
Increasing the resilience of transport systems to external impacts is a growing requirement in cities around the world. Resilience is the ability for a transport system to function despite shocks where one mode may be more affected than others. External shocks can be linked to natural disasters and extreme weather phenomena that might make it impossible for vehicles to export. In 2018, in a study of more than 500 cities around the world, more than half indicated that transport systems are some of the most vulnerable public services to climate change in the short and medium term (Ahmed and Dey, 2020[4]). Shocks can also include unexpected events, such as global pandemics, where shared forms of transport may not be ideal. Disruptions in fuel distribution or energy production can further affect a component of transport systems, thereby requiring that systems develop ways to be resilient to these possibilities.
Mode availability is a useful proxy to quantify the resilience of urban transport networks. The ITF Urban Passenger Transport Model calculates how likely it is for travellers to use another mode when one mode is disrupted in a given urban area. The model takes into account mode shares for each city and gives an indicator between 0 and 1. In an urban area with a resilience level of one, all modes in the city have the same share or are used to the same extent. A resilience level of zero indicates that a single mode is responsible for all transport activity, therefore if disrupted, the entire system fails to function. This methodology provides a simplified metric for measuring transport resilience across time that is comparable for various world regions. It adds to other measures and methodologies to quantify levels of resilience (Ahmed and Dey, 2020[4]; Jaroszweski, Hooper and Chapman, 2014[122]; Arup, 2018[123]; Temmer and Venema, 2017[124]). These can include looking at the similarity between components of the transport system, the efficiency and dependency between modes in one system, the capacity of the system to recover from shocks, and the level of co‑ordination between stakeholders (Ahmed and Dey, 2020[4]).
Highly ambitious decarbonisation policies promote a variety of modes and improve resilience of the transport ecosystem
Higher decarbonisation ambition increases the resilience of transport systems by promoting a greater variety of modal choice. For most world regions, mode resilience is the highest under Reshape+ policies. This is particularly the case in developed countries. As Figure 3.13 shows, by 2050 resilience improvements in Reshape are the highest in the United States and Canada, EEA and Turkey, and OECD Pacific. These are the world regions where, under a Recover scenario, urban passenger transport activity is concentrated in private vehicle use. Highly ambitious decarbonisation policies bring about diversification of mode choice and improve resilience in these markets. This is a positive development, which could go in hand with other more direct measures to increase infrastructure and service resilience.
Even when promoting sustainable modes, resilience could be higher when the transport system depends on a variety of modes, rather than just a few. As reflected in Figure 3.13, in developing countries improvements in resilience are limited, and in some cases such as Asia and SSA, mode resilience even decreases under the Reshape scenario. In these two regions, under the Reshape scenario transport activity is more concentrated in forms of shared mobility than in other regions. This is particularly the case due to integration of paratransit services in the shared mobility offer. From a decarbonisation perspective, this could be positive. Nonetheless, from a resilience point of view, these results highlight the importance of modal diversity for having a resilient system that can respond and adapt to external shocks.
Figure 3.13. Resilience of urban transport systems by world region in 2050

Note: Figure depicts ITF modelled estimates. Resilience of transport systems describe its ability to withstand shocks. Mode availability is a useful proxy to quantify the resilience of a transport system. The indicator depicted is calculated based mode shares in each city and is between 0 and 1. A value of 1 means that all modes are available and used equally, while a value of 0 means that is single mode is relied on for all travel in the city. A disruption to one mode would have a lower impact in more resilient cities than one that depended fully on it to serve all transport needs. EEA: European Economic Area. LAC: Latin America and the Caribbean. MENA: Middle East and North Africa. OECD Pacific: Australia, Japan, New Zealand, South Korea. SSA: Sub-Saharan Africa. Transition economies: Former Soviet Union and non-EU South-Eastern Europe.
Policy recommendations
The findings of this Transport Outlook should be seen as a call to action: simply following current commitments on a Recover trajectory will not be enough. Urban transport shows great promise to significantly reduce its carbon footprint. With the right policy tools, its emissions could be cut by almost 80%. Yet, this will require increased ambition in cities’ climate action plans. National governments can empower cities to do that, by providing the funding and policy levers to respond to the decarbonisation challenge. To this end and for effective implementation of measures, good metropolitan-wide transport governance will be essential (ITF, 2018[125]). The Covid-19 pandemic is a double-edged sword for decarbonising passenger transport in cities. The following recommendations can support authorities in reshaping their urban transport systems in a way that cuts down emissions equitably once and for all during recovery from the pandemic.
Empower cities to decarbonise urban mobility and enhance accessibility to improve well‑being
National governments need to make sure that local authorities have the right tools and capacities for increasing the ambition of their measures for decarbonising and increasing the resilience of their transport sector. They can empower local authorities by providing additional funding for inclusive and sustainable transport policies. National governments can also ensure that city authorities can legally implement measures for their wider urban area. At the local level, city authorities should take complementary measures that align with, or exceed, national targets set as part of the revision of Nationally Determined Contributions (NDCs) under the Paris Agreement. Cities need to shift their mobility policies from maximising individual mobility to increasing access to opportunities for all people to meet their needs. This shift is the prerequisite to ensure that decarbonisation policies will also deliver lasting gains for social and economic well‑being.
Prioritise funding for sustainable urban transport over investment in city roads
Cities must fund the future they want for themselves. Sustainable, inclusive, liveable cities will invest a larger share of their budget into improving public transport and active mobility rather than build more infrastructure for private cars. They will also support other shared mobility options where these provide efficient alternatives to private vehicle use. Increased and consistent funding structures for sustainable transport will make sure that cities emerge from the Covid-19 pandemic with the tools to build a more sustainable and equitable system. Lack of funds for public transport and shared mobility could put sustainability at risk and dramatically reduce mobility options for citizens with no access to cars. Over‑reliance on passenger fares can hurt public transport services, especially during disruptions like the pandemic. Funds can come from road pricing and fuel taxes, but also from land-value capture mechanisms. Potential gentrification issues from land-value capture will require attention.
Improve the quality of public transport to create more inclusive and reliable services
Better public transport will attract more users. More public transport users mean more sustainable urban mobility. An expanded route network and more frequent services would improve access to the opportunities cities offer. A focus on reliability, safety and security will raise the attractiveness of public transport for users, as will integrated ticketing and service schedules, easily accessible stations and clean vehicles. This will also play a role in gaining back users’ trust in the systems, partially lost during the Covid‑19 pandemic in many cities around the world. Good quality public transport also makes urban mobility more equitable– if authorities ensure at the same time that fares can remain affordable.
Pursue integrated land-use and transport planning for sustainable, neighbourhood-based urban development
The rise in teleworking has created the spectre of increased urban sprawl. The ability to work remotely makes commuting less of an issue, which could induce citizens to move further away from downtown office districts. Managed well, this could be an opportunity for pursuing development approaches that put neighbourhoods and public transport corridors at their heart.
Integrating transport, and land use and planning will be vital for managing urban growth sustainably. Mixed-use areas, densification and transit-oriented development shorten residents’ travel distances to essentials, making it more attractive to walk or cycle for local trips and use public transport for longer journeys.
At a micro level, integrated transport and land-use planning should ensure an allocation of urban space that serves all citizens and reconsider, for example, the societal benefits of providing public space for parking private cars. Cities have the opportunity to permanent the temporary reallocation of street space for walking and cycling made during the crisis. Seizing this opportunity could fast-track plans to expand infrastructure for safe, simple, affordable mobility.
New development patterns will also be an opportunity for making public transport services less commuter‑centric and more equitable. Neighbourhood-based developments would allow transport services to adapt to the needs of user groups with shorter, though more complex mobility patterns than those of commuters travelling to cities’ central business districts. This includes women, the elderly and children.
Create incentives for greening urban vehicle fleets
At least one-third of urban travel will still be made by private vehicles in 2050. Reducing emissions from these car trips requires technology improvements that increase fuel efficiency. Making these new fuel technologies affordable will be essential for decarbonising passenger activities, especially in areas where inhabitants do not have options other than using private vehicles. Vehicle improvements will also be important for public transport bus fleets in developing nations. Governments should design Covid-19 recovery packages that fund research and development of these new technologies, while simultaneously encouraging their uptake in private, shared and public vehicle fleets by providing more charging infrastructure and financial purchase incentives.
Nurture transport innovation and collaborate with providers of new urban mobility services to maximise benefits and minimise costs
Well-managed shared mobility solutions can complement and expand the reach of public transport, offering substantial benefits such as reduced transport emissions and improved access to opportunities. Where, on the contrary, shared mobility competes against public transport, it could affect sustainability negatively.
Authorities and operators must work together to ensure affordable services, especially in areas where public transport service is insufficient. Emerging shared mobility services might be considered for subsidies usually limited to public transport, for certain areas or user groups, where shared mobility offers last-mile solutions. Shared mobility can also provide cost-effective solutions in low-density areas or at off-peak times. Combined service offers with public transport can be co‑ordinated through a Mobility-as-a-Service (MaaS) platform.
Combine transport decarbonisation and resilience measures now to meet future demand in sustainable ways and withstand disruptions
Ambitious decarbonisation policies for urban mobility can increase the resilience of cities’ transport systems against disruptions. Climate mitigation policies will reduce overdependence on private cars and create a multimodal network. Multimodal systems are more agile at adapting to future changes in travel demand and unexpected disruptions like extreme weather events or pandemics. Beyond modal diversity, authorities need to consider the capacity of the transport system to adapt and recover its functions after external events. The resilience of operations and infrastructure should also be considered.
References
[4] Ahmed, S. and K. Dey (2020), “Resilience modeling concepts in transportation systems: a comprehensive review based on mode, and modeling techniques”, Journal of Infrastructure Preservation and Resilience, Vol. 1/1, http://dx.doi.org/10.1186/s43065-020-00008-9.
[80] Amato, F. et al. (2014), Urban air quality: The challenge of traffic non-exhaust emissions, Elsevier, http://dx.doi.org/10.1016/j.jhazmat.2014.04.053.
[123] Arup (2018), Cities: Qualitative Resilience Profile, https://www.cityresilienceindex.org/#/city-profiles.
[100] Badiora, A., C. Wojuade and A. Adeyemi (2020), “Personal safety and improvements concerns in public places”, Journal of Place Management and Development, Vol. 13/3, pp. 319-346, http://dx.doi.org/10.1108/jpmd-03-2019-0013.
[84] Baker, J. and G. Gadgil (2017), East Asia and Pacific Cities: Expanding Opportunities for the Urban Poor, World Bank, Washington, DC, http://dx.doi.org/10.1596/978-1-4648-1093-0.
[57] Banister, D. (2019), Transport for all, Routledge, http://dx.doi.org/10.1080/01441647.2019.1582905.
[9] Banister, D. (2018), Inequality in Transport, Alexandrine Press, https://books.google.ch/books?id=NOKHuwEACAAJ.
[78] Barnes, J., T. Chatterton and J. Longhurst (2019), “Emissions vs exposure: Increasing injustice from road traffic-related air pollution in the United Kingdom”, Transportation Research Part D: Transport and Environment, Vol. 73/June, pp. 56-66, http://dx.doi.org/10.1016/j.trd.2019.05.012.
[45] BBC (2020), Coronavirus: Boom time for bikes as virus changes lifestyles - BBC News, https://www.bbc.com/news/business-52564351 (accessed on 7 October 2020).
[38] BBC (2020), Coronavirus: Wuhan shuts public transport over outbreak - BBC News, https://www.bbc.com/news/world-asia-china-51215348 (accessed on 7 October 2020).
[63] Bloomberg News (2020), The Car Is Staging a Comeback, Spurring Oil’s Recovery, https://www-bloomberg-com.cdn.ampproject.org/c/s/www.bloomberg.com/amp/news/articles/2020-05-10/the-car-is-staging-a-comeback-spurring-oil-s-recovery (accessed on 8 October 2020).
[31] Bloom, N. (2020), How working from home works out, Stanford Institute for Economic Policy Research, https://siepr.stanford.edu/research/publications/how-working-home-works-out (accessed on 9 October 2020).
[82] Bocarejo, J. and L. Urrego (2020), “The impacts of formalization and integration of public transport in social equity : The case of Bogota”, Research in Transportation Business & Management June, p. 100560, http://dx.doi.org/10.1016/j.rtbm.2020.100560.
[114] Bocarejo, J. and L. Urrego (2020), “The impacts of formalization and integration of public transport in social equity: The case of Bogota”, Research in Transportation Business & Management, p. 100560, http://dx.doi.org/10.1016/j.rtbm.2020.100560.
[68] Buckle, S. et al. (2020), Draft discussion paper: Addressing the COVID and climate crises: potential economic recovery pathways and their implications for climate change mitigation, NDCs and broader socio-economic goals.
[3] C40 (2020), Climate Action Planning Resource Centre, https://resourcecentre.c40.org/.
[65] Calvino, F. and M. Virgillito (2018), “The innovation-employment nexus: A critical survey of theory and empirics”, Journal of Economic Surveys, Vol. 32/1, pp. 83-117, http://dx.doi.org/10.1111/joes.12190.
[51] CBC (2020), TransLink says it’s losing $75M a month and faces ’really unpleasant options’ without emergency funding | CBC News, https://www.cbc.ca/news/canada/british-columbia/translink-seeking-emergeny-funding-coronavirus-pandemic-1.5531302 (accessed on 9 October 2020).
[6] CDP (2020), Cities at risk: dealing with the pressures of climate change, https://www.cdp.net/en/research/global-reports/cities-at-risk.
[101] Chant, S. and C. Mcllwaine (2016), Cities, Slums and Gender in the Global South: Towards a feminised urban future (Regions and Cities, Routledge.
[85] Chen, Y. et al. (2017), Unequal commutes: Job accessibility and employment in Accra, https://www.theigc.org/wp-content/uploads/2017/08/Chen-et-al-2017-Final-report.pdf.
[58] Chu, T. (2020), Covid-19 Is Not The ‘Death Of The City’ - It’s The Rise Of The Neighborhood Center, Forbes, https://www.forbes.com/sites/tiffanychu/2020/10/01/covid-19-is-not-the-death-of-the-cityits-the-rise-of-the-neighborhood-center/#70556ab32491 (accessed on 15 October 2020).
[29] Citymapper (2020), Citymapper Mobility Index, https://citymapper.com/cmi (accessed on 7 October 2020).
[109] Cohen, S. and S. Shirazi (2017), Can We Advance Social Equity with Shared, Autonomous and Electric Vehicles?, ITS UC Davis Institute or Transportation Studies.
[93] Crozet, Y. and A. Mercier (2018), “Urban Toll: Rethinking Acceptability Through Accessibility”, International Transport Forum Discussion Papers, No. 2018/16, OECD Publishing, Paris, https://dx.doi.org/10.1787/af22477a-en.
[37] de la Garzia, A. (2020), COVID-19 Has Been ’Apocalyptic’ for Public Transit | Time, https://time.com/5869375/public-transit-coronavirus-covid/ (accessed on 7 October 2020).
[61] Deloitte (2020), Mobility after coronavirus – from public to private, https://www2.deloitte.com/ch/en/pages/public-sector/articles/mobilitaet-nach-der-corona-krise.html (accessed on 8 October 2020).
[107] Deloitte (2019), Global mobile consumer trends.
[90] Di Ciommo, F. and K. Lucas (2014), “Evaluating the equity effects of road-pricing in the European urban context – The Madrid Metropolitan Area”, Applied Geography, Vol. 54, pp. 74-82, http://dx.doi.org/10.1016/j.apgeog.2014.07.015.
[49] Dingel, J. and B. Neiman (2020), How Many Jobs Can be Done at Home?, Becker Friedman Institute, Chicago, https://github.com/jdingel/DingelNeiman-workathome. (accessed on 9 October 2020).
[36] Dormer, D. (2020), Calgary Transit reducing frequency of buses and trains in response to COVID-19 | CTV News, https://calgary.ctvnews.ca/calgary-transit-reducing-frequency-of-buses-and-trains-in-response-to-covid-19-1.4877748 (accessed on 7 October 2020).
[66] Dowd, J. (2017), Aggregate Economic Return on Investment in the U.S. DOE Office of Energy Efficiency and Renewable Energy, US Department of Energy, https://energy.gov/sites/prod/files/2015/05/f22/evaluating_realized_rd_mpacts_9-22-14.pdf (accessed on 9 October 2020).
[110] Flores, O. (2020), ITF Interview to Onésimo Flores (CEO) on impacts of Covid-19 to Jetty.
[22] Fulton, L. et al. (2017), Three Revolutions in Urban Transportation, UC Davis, Davis.
[120] Gauvin, L. et al. (2020), “Gender gaps in urban mobility”, Humanities and Social Sciences Communications, Vol. 7/1, http://dx.doi.org/10.1057/s41599-020-0500-x.
[87] Geurs, K. and B. van Wee (2004), “Accessibility evaluation of land-use and transport strategies: review and research directions”, Journal of Transport Geography, Vol. 12/2, pp. 127-140, http://dx.doi.org/10.1016/j.jtrangeo.2003.10.005.
[21] GIZ (2019), Sustainable Urban Transport: Avoid-Shift-Improve (A-S-I), https://www.transformative-mobility.org/assets/publications/ASI_TUMI_SUTP_iNUA_No-9_April-2019.pdf.
[2] GIZ (2017), Transport in Nationally Determined Contributions (NDCs): Lessons learnt from case studies of rapidly motorising countries, https://www.international-climate-initiative.com/fileadmin/Dokumente/2017/171115_Publikation_EN_TransportInNDCs.pdf.
[44] Goetsch, H. and T. Quiros (2020), COVID-19 creates new momentum for cycling and walking. We can’t let it go to waste!, World Bank Blogs, https://blogs.worldbank.org/transport/covid-19-creates-new-momentum-cycling-and-walking-we-cant-let-it-go-waste (accessed on 7 October 2020).
[69] Goetz, M. (2020), Policy Brief Electric Vehicle Charging Considerations for Shared, Automated Fleets Introduction and Background, UC Davis Institute of Transportation Studies.
[32] Google LLC (2020), COVID-19 Community Mobility Reports, https://www.google.com/covid19/mobility/ (accessed on 7 October 2020).
[17] Gössling, S. et al. (2016), “Urban Space Distribution and Sustainable Transport”, Transport Reviews, Vol. 36/5, pp. 659-679, http://dx.doi.org/10.1080/01441647.2016.1147101.
[16] Gota, S. et al. (2019), Decarbonising transport to achieve Paris Agreement targets, http://dx.doi.org/10.1007/s12053-018-9671-3.
[11] Gough, I. (2011), Climate Change, Double Injustice and Social Policy A Case Study of the United Kingdom.
[50] Guyot, K. and I. Sawhill (2020), Telecommuting will likely continue long after the pandemic, Brookings, https://www.brookings.edu/blog/up-front/2020/04/06/telecommuting-will-likely-continue-long-after-the-pandemic/ (accessed on 9 October 2020).
[97] Guzman, L. and D. Oviedo (2018), “Accessibility, affordability and equity: Assessing ‘pro-poor’ public transport subsidies in Bogotá”, Transport Policy, Vol. 68, pp. 37-51, http://dx.doi.org/10.1016/j.tranpol.2018.04.012.
[46] Hook, A. et al. (2020), “A systematic review of the energy and climate impacts of teleworking”, Environmental Research Letters, Vol. 15, p. 93003, http://dx.doi.org/10.1088/1748-9326/ab8a84.
[43] Ibold, S. et al. (2020), The COVID-19 outbreak and implications to sustainable urban mobility – some observations | Transformative Urban Mobility Initiative (TUMI), TUMI, https://www.transformative-mobility.org/news/the-covid-19-outbreak-and-implications-to-public-transport-some-observations (accessed on 6 October 2020).
[73] ICCT (2019), Transportation Roadmap, https://www.theicct.org/transportation-roadmap (accessed on 13 March 2019).
[113] IDB and ITF (2020), Measuring accessibility in Latin American cities: the case of Bogota, Mexico City and Santiago.
[64] IEA (2020), Clean energy innovation in the Covid-19 crisis – Analysis - IEA, https://www.iea.org/articles/clean-energy-innovation-in-the-covid-19-crisis (accessed on 9 October 2020).
[13] IEA (2020), IEA Mobility Model, https://www.iea.org/areas-of-work/programmes-and-partnerships/the-iea-mobility-model.
[48] IEA (2020), Working from home can save energy and reduce emissions. But how much? – Analysis - IEA, https://www.iea.org/commentaries/working-from-home-can-save-energy-and-reduce-emissions-but-how-much (accessed on 9 October 2020).
[15] IEA (2019), Growing preference for SUVs challenges emissions reductions in passenger car market – Analysis, https://www.iea.org/commentaries/growing-preference-for-suvs-challenges-emissions-reductions-in-passenger-car-market (accessed on 28 October 2020).
[40] IGC (2020), Impact of COVID-19 on public transport, https://www.theigc.org/blog/impact-of-covid-19-on-public-transport/ (accessed on 29 October 2020).
[62] Ipsos (2020), IMPACT OF CORONAVIRUS TO NEW CAR PURCHASE IN CHINA.
[18] ITF (2021), Avoiding Car Dependency, OECD Publishing, Paris.
[116] ITF (2020), Best Practice for Urban Road Safety Case Studies, https://www.itf-oecd.org/sites/default/files/docs/best-practice-urban-road-safety.pdf.
[56] ITF (2020), Good to Go? Assessing the Environmental Performance of New Mobility Corporate Partnership Board Report, OECD Publishing, Paris, https://doi.org/10.1787/f5cd236b-en. (accessed on 15 October 2020).
[115] ITF (2020), Safe Micromobility, https://www.itf-oecd.org/sites/default/files/docs/safe-micromobility_1.pdf.
[25] ITF (2020), “Shared Mobility Simulations for Lyon”, International Transport Forum Policy Papers, No. 74, OECD Publishing, Paris, https://dx.doi.org/10.1787/031951c3-en.
[94] ITF (2019), Benchmarking Accessibility in Cities: Measuring the impact of proximity and transport performance.
[86] ITF (2019), Improving Transport Planning and Investment Through the Use of Accessibility Indicators, https://www.itf-oecd.org/sites/default/files/docs/improving-transport-planning-investment-accessibility-indicators.pdf.
[28] ITF (2019), ITF Transport Outlook 2019, OECD Publishing, Paris, https://dx.doi.org/10.1787/transp_outlook-en-2019-en.
[112] ITF (2019), Regulating App-Based Mobility Services: Summary and Conclusions.
[119] ITF (2019), Transport Connectivity A Gender Perspective, OECD Publishing, Paris, http://www.itf-oecd.org (accessed on 26 January 2021).
[27] ITF (2019), “Transport Innovations from the Global South: case studies, insights, recommendations”, https://www.itf-oecd.org/sites/default/files/docs/transport-innovations-global-south.pdf.
[125] ITF (2018), Policy Directions for Establishing a Metropolitan Transport Authority for Korea’s Capital Region Ca, OECD Publishing.
[102] ITF (2018), Shared Mobility Simulations for Dublin Case-Specific Policy Analysis, OECD, Paris, http://www.itf-oecd.org (accessed on 2 October 2020).
[19] ITF (2018), The Shared-Use City: Managing the Curb, OECD Publishing, Paris, http://www.itf-oecd.org.
[26] ITF (2016), “Shared mobility: innovation for liveable cities”, International Transport Forum Policy Papers Nº 21, http://dx.doi.org/10.1787/24108871.
[1] ITF/OECD (2018), Transport CO2 and the Paris Climate Agreement Reviewing the Impact of Nationally Determined Contributions, https://www.itf-oecd.org/transport-co2-paris-climate-agreement-ndcs.
[108] ITU and UNESCO (2019), The State of Broadband: Broadband as a Foundation for Sustainable Development.
[122] Jaroszweski, D., E. Hooper and L. Chapman (2014), “The impact of climate change on urban transport resilience in a changing world”, Progress in Physical Geography: Earth and Environment, Vol. 38/4, pp. 448-463, http://dx.doi.org/10.1177/0309133314538741.
[106] Kodransky, M. and G. Lewenstein (2014), Connecting Low-Income People to Opportunity with Shared Mobility, nstitute for Transportation & Development Policy (ITDP), https://itdpdotorg.wpengine.com/wp-content/uploads/2014/10/Shared-Mobility_Full-Report.pdf.
[96] Li, M. (2019), Measuring Public Transport Fare Affordability, https://thredbo-conference-series.org/downloads/thredbo16/Fare-Affordability-Benchmarking-Study.pdf.
[88] Litman, T. (2020), “Evaluating Transportation Equity Guidance For Incorporating Distributional Impacts in Transportation Planning”, World Transport Policy & Practice, Vol. 8/2, pp. 50-65, https://www.vtpi.org/equity.pdf.
[92] Mattioli, G. (2017), “‘Forced Car Ownership’ in the UK and Germany: Socio-Spatial Patterns and Potential Economic Stress Impacts”, Social Inclusion, Vol. 5/4, pp. 147-160, http://dx.doi.org/10.17645/si.v5i4.1081.
[41] McArthur, J. and E. Smeds (2020), Coronavirus showed the way cities fund public transport is broken – here’s how it needs to change, https://theconversation.com/coronavirus-showed-the-way-cities-fund-public-transport-is-broken-heres-how-it-needs-to-change-145136 (accessed on 7 October 2020).
[52] Medda, F. (2012), “Land value capture finance for transport accessibility: A review”, Journal of Transport Geography, Vol. 25, pp. 154-161, http://dx.doi.org/10.1016/j.jtrangeo.2012.07.013.
[117] Miralles-Guasch, C., M. Melo and O. Marquet (2015), “A gender analysis of everyday mobility in urban and rural territories: from challenges to sustainability”, Gender, Place & Culture, Vol. 23/3, pp. 398-417, http://dx.doi.org/10.1080/0966369x.2015.1013448.
[121] Montoya-Robledo, V. et al. (2020), “Gender stereotypes affecting active mobility of care in Bogotá”, Transportation Research Part D: Transport and Environment, Vol. 86, p. 102470, http://dx.doi.org/10.1016/j.trd.2020.102470.
[59] Moreno, C. (2020), Proximité urbaine et l’amour des lieux, http://www.moreno-web.net/proximite-urbaine-et-lamour-des-lieux-chrono-urbanisme-chronotopie-topophilie-par-carlos-moreno/ (accessed on 15 October 2020).
[83] Moreno-Monroy, A. (2016), “Access to public transport and labor informality”, IZA World of Labor, http://dx.doi.org/10.15185/izawol.274.
[118] Ng, W. and A. Acker (2018), Understanding Urban Travel Behaviour by Gender for Efficient and Equitable Transport Policies, OECD, Paris, http://www.itf-oecd.org (accessed on 26 January 2021).
[8] OECD (2019), “Delivering accessible and sustainable mobility”, in Accelerating Climate Action: Refocusing Policies through a Well-being Lens, OECD Publishing, Paris, https://dx.doi.org/10.1787/7dfbd08d-en.
[71] OECD/European Commission (2020), Cities in the World: A New Perspective on Urbanisation, OECD Urban Studies, OECD Publishing, Paris, https://dx.doi.org/10.1787/d0efcbda-en.
[7] OECD/ITF (2019), Benchmarking Accessibility in Cities: Measuring the Impact of Proximity and Transport Performance, https://www.itf-oecd.org/sites/default/files/docs/accessibility-proximity-transport-performance_2.pdf.
[14] OICA (2020), Motorization Rate 2015 - Worldwide, http://www.oica.net/world-vehicles-in-use-all-vehicles-2/.
[103] Pangbourne, K. et al. (2020), “Questioning mobility as a service: Unanticipated implications for society and governance”, Transportation Research Part A: Policy and Practice, Vol. 131, pp. 35-49, http://dx.doi.org/10.1016/j.tra.2019.09.033.
[79] Panko, J. et al. (2019), “Evaluation of tire wear contribution to PM2.5 in urban environments”, Atmosphere, Vol. 10/2, pp. 1-14, http://dx.doi.org/10.3390/ATMOS10020099.
[60] Paris en Commun (2020), Le programme - Anne Hidalgo 2020, https://annehidalgo2020.com/le-programme/ (accessed on 19 October 2020).
[95] Peralta Quiros, T. and C. Rodríguez Hernández (2016), To measure the real impact of transport services, affordability needs to be part of the equation, https://blogs.worldbank.org/transport/measure-real-impact-transport-services-affordability-needs-be-part-equation.
[20] Pimentel, C. and F. Alvelos (2018), Integrated urban freight logistics combining passenger and freight flows - Mathematical model proposal, Elsevier B.V., http://dx.doi.org/10.1016/j.trpro.2018.09.010.
[35] Puentes, R. (2020), COVID’s Differing Impact on Transit Ridership – The Eno Center for Transportation, https://www.enotrans.org/article/covids-differing-impact-on-transit-ridership/ (accessed on 7 October 2020).
[67] PWC Strategy (2020), The Impact of COVID-19 on the European Automotive Market Learnings from past crises, future market outlook and recommended actions for OEMs.
[105] Qian, X., M. Jaller and D. Niemeier (2020), “Enhancing equitable service level: Which can address better, dockless or dock-based Bikeshare systems?”, Journal of Transport Geography, Vol. 86, p. 102784, http://dx.doi.org/10.1016/j.jtrangeo.2020.102784.
[104] Qian, X. and D. Niemeier (2019), “High impact prioritization of bikeshare program investment to improve disadvantaged communities’ access to jobs and essential services”, Journal of Transport Geography, Vol. 76, pp. 52-70, http://dx.doi.org/10.1016/j.jtrangeo.2019.02.008.
[77] Reichmuth, D. (2019), Inequitable Exposure to Air Pollution from Vehicles in California, Union of Concerned Scientists, Cambridge, Massachusetts, https://www.ucsusa.org/sites/default/files/attach/2019/02/cv-air-pollution-CA-web.pdf (accessed on 12 October 2020).
[74] Rodrigue, J., C. Comtois and B. Slack (2009), The geography of transport systems [electronic resource], https://transportgeography.org/?page_id=9422 (accessed on 20 January 2019).
[23] Salazar Ferro, P. (2015), THE CHALLENGE OF FINDING A ROLE FOR PARATRANSIT SERVICES IN THE GLOBAL SOUTH, https://www.codatu.org/wp-content/uploads/Pablo-Salazar-Ferro.pdf.
[54] Sclar, E., M. Lönnroth and C. Wolmar (2016), Improving Urban Access: New Approaches to Funding Transport Investment, Routledge, https://books.google.ch/books?id=eX6VAQAACAAJ.
[99] Shibata, S. (2020), “Are women-only cars (WOC) a solution to groping? A survey among college students in Tokyo/Kanagawa, Japan”, International Journal of Comparative and Applied Criminal Justice, Vol. 44/4, pp. 293-305, http://dx.doi.org/10.1080/01924036.2020.1719533.
[76] Slovic, A. et al. (2016), “How Can Urban Policies Improve Air Quality and Help Mitigate Global Climate Change: a Systematic Mapping Review”, Journal of Urban Health, Vol. 93/1, pp. 73-95, http://dx.doi.org/10.1007/s11524-015-0007-8.
[81] Soret, A., M. Guevara and J. Baldasano (2014), “The potential impacts of electric vehicles on air quality in the urban areas of Barcelona and Madrid (Spain)”, Atmospheric Environment, Vol. 99/2, pp. 51-63, http://dx.doi.org/10.1016/j.atmosenv.2014.09.048.
[30] Sostero, M. et al. (2020), “Teleworkability and the COVID-19 crisis: a new digital divide?”, Eurofound.
[55] Steer (2020), The Covid-19 Funding Gap: The Case for Continuing Support for Urban Public Transport, http://www.steergroup.com (accessed on 9 October 2020).
[10] Sustainable Development Commission (2011), Fairness in a Car-dependent Society 2-Fairness in Transport-Finding an alternative to car dependency-Sustainable Development Commission.
[89] Taylor, B. (2010), How Fair is Road Pricing? Evaluating Equity in Transportation Pricing and Finance, Bipartisan Policy Center.
[124] Temmer, J. and H. Venema (2017), Building a Climate-Resilient City: Transportation infrastructure, International Institute for Sustainable Development, https://www.iisd.org/system/files/publications/pcc-brief-climate-resilient-city-transportation-infrastructure.pdf.
[24] The World Bank (2019), GREEN YOUR BUS RIDE Clean Buses in Latin America Summary report, http://documents1.worldbank.org/curated/en/410331548180859451/pdf/133929-WP-PUBLIC-P164403-Summary-Report-Green-Your-Bus-Ride.pdf.
[34] TomTom (2020), Tracking the impact of COVID-19 through traffic, https://www.tomtom.com/covid-19/ (accessed on 7 October 2020).
[91] TransForm (2019), PRICING ROADS, ADVANCING EQUITY.
[39] TransitCenter (2020), Transit Is Essential: 2.8 Million U.S. Essential Workers Ride Transit to Their Jobs - TransitCenter, https://transitcenter.org/2-8-million-u-s-essential-workers-ride-transit-to-their-jobs/ (accessed on 7 October 2020).
[53] Transport for London (2017), Land value capture - Final report.
[42] UITP (2020), Resuming public transport services post-lockdown | UITP ||, https://www.uitp.org/publications/covid-19-pandemic-resuming-public-transport-services-post-lockdown/ (accessed on 7 October 2020).
[12] UN (2018), World Urbanization Prospects: The 2018 Revision, https://population.un.org/wup/ (accessed on 29 April 2019).
[70] United Nations, D. (2019), World Urbanization Prospects The 2018 Revision, United Nations, New York.
[98] van Lierop, D. and A. El-Geneidy (2016), “Enjoying loyalty: The relationship between service quality, customer satisfaction, and behavioral intentions in public transit”, Research in Transportation Economics, Vol. 59, pp. 50-59, http://dx.doi.org/10.1016/j.retrec.2016.04.001.
[33] Venter, Z. et al. (2020), “COVID-19 lockdowns cause global air pollution declines”, Proceedings of the National Academy of Sciences of the United States of America, Vol. 117/32, pp. 18984-18990, http://dx.doi.org/10.1073/pnas.2006853117.
[75] WHO (2018), Ambient (outdoor) air pollution, https://www.who.int/news-room/fact-sheets/detail/ambient-(outdoor)-air-quality-and-health (accessed on 12 October 2020).
[111] Wiprächtiger, D. et al. (2019), “Access-based business model innovation in frontier markets: Case study of shared mobility in Timor-Leste”, Technological Forecasting and Social Change, Vol. 143, pp. 224-238, http://dx.doi.org/10.1016/j.techfore.2019.02.004.
[72] WorldPop (2020), WorldPop datasets, https://www.worldpop.org/ (accessed on 3 November 2020).
[5] Zhou, Y., J. Wang and H. Yang (2019), “Resilience of Transportation Systems: Concepts and Comprehensive Review”, IEEE Transactions on Intelligent Transportation Systems, Vol. 20/12, pp. 4262-4276, http://dx.doi.org/10.1109/tits.2018.2883766.
[47] Zhu, P. et al. (2018), “Metropolitan size and the impacts of telecommuting on personal travel”, Transportation, Vol. 45/2, pp. 385-414, http://dx.doi.org/10.1007/s11116-017-9846-3.