The fragile Arctic environment is particularly vulnerable to some pollutants that contribute to atmospheric warming, such as black carbon and ground-level ozone. While an important share of air pollutants reach the Arctic from outside the region, emissions from the Arctic Council countries have the greatest impact. This chapter introduces the motivations for policy action on air pollution in Arctic Council countries, highlighting the environmental, health and economic benefits. Particular attention is given to the role of short-lived climate pollutants, and specifically black carbon, whose reduction plays an important role in mitigating climate change. The chapter then provides an overview of the sectoral sources of air pollution in Arctic Council countries.
The Economic Benefits of Air Quality Improvements in Arctic Council Countries
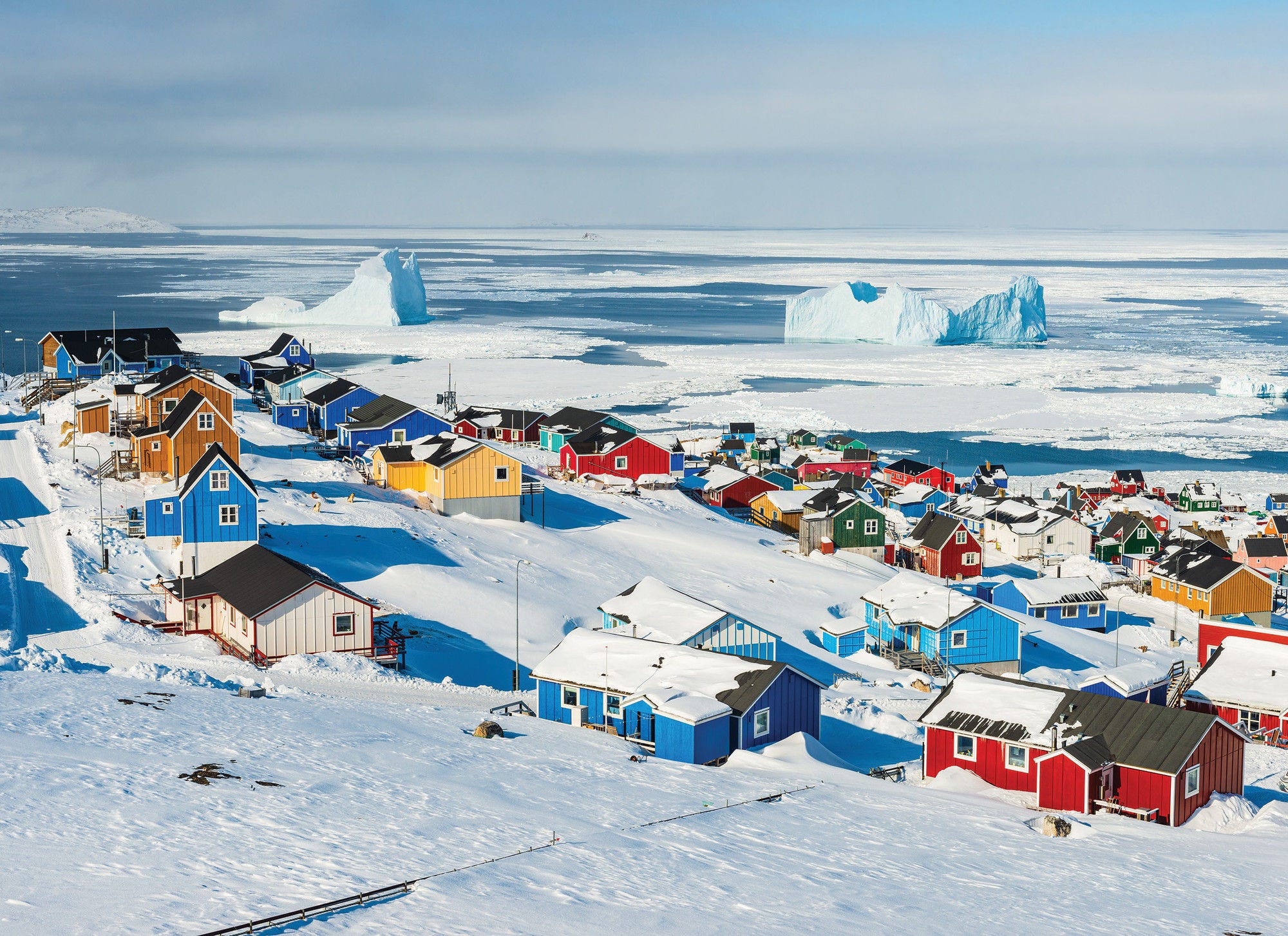
1. Air pollution in Arctic Council countries
Abstract
1.1. Introduction
Air pollution is one of the most serious environmental challenges at the global level, with adverse effects on human health, well-being and the environment. In 2017, outdoor air pollution alone was responsible for approximately 3.5 million deaths (GBD, 2018[1]); in the absence of stricter policies, air pollution-related mortality is projected to reach a global total of 6 to 9 million by 2060 (OECD, 2016[2]). Air pollution also has significant impacts on ecosystems and climate change, as some air pollutants – most notably black carbon and ground-level ozone– are major contributors to atmospheric warming. Such impacts are especially detrimental for fragile environments, such as the Arctic.
Owing to the low population density and limited economic activity characterising the Arctic region, local emissions of air pollutants in the Arctic are limited. An important share of air pollutants reaching the Arctic originates at lower latitudes, highlighting the need to reduce emissions well beyond the Arctic region. Most notably, emissions occurring in Arctic Council (AC) countries have greater impact in the Arctic region, due to their proximity (AMAP, 2015[3]). For this reason, Canada, Denmark, Finland, Iceland, Norway, the Russian Federation (hereafter Russia), Sweden and the United States have affirmed their support to achieve a collective 25-33% reduction of black carbon emissions by 2025 compared to 2013 levels (Arctic Council, 2019[4]).
Alongside concerns about black carbon emissions, Arctic Council countries have put in place policies to reduce emissions of a wide range of air pollutants. Besides delivering environmental and health benefits, improved air quality can have positive effects on the economy, increasing labour productivity and crop yields while lowering health expenditures (OECD, 2016[2]). Recent literature has focused on the economic benefits of improved air quality and on the additional co-benefits of climate policies (Amann et al., 2020[5]; Harmsen et al., 2020[6]; Markandya et al., 2018[7]; Amann et al., 2017[8]; Vandyck et al., 2018[9]). For example, Vandyck et al. (2018[9]) demonstrate that the air quality co-benefits of climate policy alone counterbalance the costs of meeting the Nationally Determined Contributions that countries presented under the Paris Agreement. Recent evidence from the empirical literature also highlights the substantial economic benefits of reducing air pollution (Dechezleprêtre, Rivers and Stadler, 2019[10]).
This report contributes to the existing literature by quantifying the economic consequences of implementing policies that directly target air pollution in Arctic Council countries. In particular, the report compares the outcomes of five scenarios:
A baseline scenario that assumes the continuation of air pollution policies and legislation already in place.
A scenario in which Arctic Council countries implement additional policies to reduce air pollution.
A scenario in which these policies are also implemented in the 13 Observer countries to the Arctic Council.1
A scenario that assumes the global implementation of these policies.
An integrated policy scenario, in which these air pollution policies are implemented alongside ambitious climate and energy transition policies.2
While this analysis focuses primarily on the benefits of policy action in Arctic Council countries by comparing current legislation with additional policy action in these countries, the additional scenarios consider two important aspects that can influence the success of air pollution policies: transboundary air pollution, and integrated policy action across different environmental domains. In all policy scenarios, air pollutant emissions are reduced through policy-induced adoption of the best available techniques (BATs). Such policies target a wide range of air pollutants, aiming to improve air quality. Specifically, this report considers emissions of black carbon (BC), organic carbon (OC), sulphur dioxide (SO2), nitrogen oxides (NOx), non-methane volatile organic compounds (NMVOCs), nitrous oxide (N2O), carbon monoxide (CO), and ammonia (NH3).3 These pollutants are particularly relevant as they drive the concentrations of fine particulate matter (PM2.5) and ground-level ozone (O3).
Building on the report The Economic Consequences of Outdoor Air Pollution (OECD, 2016[2]), this report adopts a modelling approach that links economic activity to projected emissions, pollutant concentrations, the biophysical impacts of outdoor air pollution, and their feedback effects on the economy. The main modelling tool used in the analysis is the OECD ENV-Linkages model (Chateau, Dellink and Lanzi, 2014[11]), which can quantify the economic consequences of air pollution to 2050. This economic analysis is supported by results from the Greenhouse Gas and Air Pollution Interactions and Synergies (GAINS) model developed by the International Institute for Applied Systems Analysis (IIASA),4 which provides projections of air pollutant emissions, as well as the costs of adopting BATs to reduce air pollution (Amann et al., 2011[12]; Höglund-Isaksson et al., 2020[13]; Winiwarter et al., 2018[14]; Klimont et al., 2017[15]). Finally, the TM5-FASST model developed by the Joint Research Centre of the European Commission links emission projections with human exposure to PM2.5 and ground-level ozone pollution (Van Dingenen et al., 2018[16]).
The economic consequences of air pollution policies are quantified in this report by considering two complementary aspects: (1) macroeconomic effects; and (2) welfare improvements. The macroeconomic effects result from the market impacts of air pollution, which affect specific sectors resulting in changes in economic growth and gross domestic product (GDP). Welfare improvements account for the impacts of air pollution on well-being and, in this report, they result from changes in the incidence of air pollution-related mortality and illness. The macroeconomic effects are calculated in ENV-Linkages considering both the benefits and associated costs of reducing emissions and improving air quality. Drawing on previous OECD analysis (OECD, 2016[2]), the benefits are calculated estimating the improvements in labour productivity and agricultural productivity and the decrease in health expenditures that would result from improved air quality. The emission reduction costs are included in ENV-Linkages as additional sector-specific costs for firms and households, based on the costs the BATs from the GAINS model. Meanwhile, welfare improvements from air pollution policies are quantified using valuation techniques that attribute a monetary value to lives lost and to the pain and suffering associated with illness.
This report is structured as follows. The rest of Chapter 1 highlights the main motivations for policy action to reduce air pollution in Arctic Council countries and provides an overview of the sources, distribution and impacts of air pollution in these countries. Chapter 2 outlines the methodology used for the modelling analysis and describes the policy scenarios studied in the report. Chapter 3 presents the emission and concentration projections as well as the health benefits from policy action on air pollution. Chapter 4 outlines the economic consequences of the policy scenarios, including both macroeconomic and welfare effects. Chapter 5 illustrates possible additional benefits from wider geographical adoption of air pollution policies and from integrated air pollution, climate and energy transition policies. Finally, Chapter 6 discusses the overall benefits of air pollution policies, also consdiering the additional benefits of policy action that could not be included in the modelling analysis, and concludes with a call for action on air pollution.
1.2. The focus of Arctic Council countries on air pollution
Air pollution is one of the most serious environmental challenges of our times, due to its severe effects on human health and the environment. The impacts of air pollution – and in particular of short-lived climate pollutants (SLCPs) – are particularly detrimental for the fragile Arctic environment due to the region’s exceptional sensitivity to local and global temperature changes (EPA, 2012[17]). SLCPs are air pollutants that also have a warming impact on the climate. They include black carbon (Box 1.1), methane, ground-level ozone, and hydrofluorocarbons.5
While local emissions contribute significantly to air pollution in the Arctic region, most pollutants affecting the Arctic are transported to the region from lower latitudes by the wind (AMAP, 2015[3]). As a consequence, emission reductions occurring in the whole territory of the eight Arctic Council countries can largely help to preserve air quality in the Arctic. Emission reductions in other regions – and particularly in the 13 Observer countries to the Arctic Council (Table 1.1) – can contribute further to reducing pollutant concentrations in the Arctic.
Table 1.1. Arctic Council countries and Observers
Arctic Council countries |
Arctic Council Observers |
|
---|---|---|
Canada United States Russian Federation Denmark Finland Iceland Norway Sweden |
France Germany Italy Japan Netherlands People’s Republic of China (hereafter, China) Poland |
India Korea Singapore Spain Switzerland United Kingdom |
Source: (Arctic Council, 2021[18]).
In recent years, Arctic Council countries have increased their engagement for addressing air pollution. Owing to its effect on the local and global climate (Box 1.1), black carbon has become a major concern for these countries, which have set an aspirational collective target to reduce their aggregate BC emissions from between 25 to 33% by 2025 compared to 2013 levels (Arctic Council, 2019[4]).6
Besides helping to preserve the Arctic environment, improved air quality in the region would also have economic and health benefits throughout the whole territory of the eight Arctic Council countries. These benefits would be particularly significant in densely populated areas characterised by high levels of economic activity, as well as in agricultural areas. Indeed, lower pollution levels lead to improved health and improved agricultural productivity (Van Dingenen et al., 2009[19]; Holland, 2014[20]; OECD, 2016[2]).
The health impacts of outdoor air pollution in Arctic Council countries are large. The Global Burden of Disease (GBD) project estimates that in 2017, over 420 000 people died from air pollution in the region, accounting for almost 9% of all deaths in Arctic Council countries that year (GBD, 2018[21]). In addition, human exposure to high concentrations of PM2.5 increases the risk of cardiovascular diseases (e.g. cardiac arrhythmia, stroke, and coronary and ischaemic heart disease), respiratory problems (e.g. asthma, bronchitis, and pulmonary dysfunctions), lung cancer, respiratory infections, diabetes and kidney failure. Furthermore, exposure to PM2.5 is associated with adverse birth and nervous system outcomes, reduced cognitive functions and accelerated biological aging. Exposure to ground-level ozone is also responsible for respiratory diseases, leading to significant levels of mortality and morbidity. Lower concentrations of fine particulate matter and ground-level ozone would reduce the incidence of air pollution-related illnesses and deaths (GBD, 2018[21]).
Box 1.1. Black carbon and climate change in the Arctic
Black carbon is a primary component of fine particulate matter and can have serious impacts on human health and the environment. Although the eight Arctic Council countries are responsible for 8% of black carbon emissions globally, they are responsible for 30% of BC’s warming effect in the Arctic (AMAP, 2015[3]).
Due to its dark colour, BC is the most light-absorbing component of particulate matter and, as such, it is a major contributor to climate change. BC is a short-lived climate pollutant with a strong atmospheric warming potential. It is the second-largest anthropogenic contributor to global warming after carbon dioxide (CO2) (Yang et al., 2019[22]; Bond et al., 2013[23]; Shrestha, Traina and Swanston, 2010[24]; Moffet and Prather, 2009[25]; AMAP, 2019[26]). Despite its short lifetime in the air, the radiative forcing of one gram of black carbon is comparable to that of a ton of carbon dioxide (CCAC and WHO, 2015[27]).
Besides sparking a warming effect when suspended in the atmosphere, black carbon further interferes with the Arctic climate when it is deposited on snow and ice cover, darkening the surface and thus decreasing the albedo, i.e. the ability to reflect solar radiation (AMAP, 2015[3]). The Arctic region is particularly vulnerable to the effects of BC deposition, due to the presence of large snow and ice-covered surfaces. The dark coat accelerates ice and snow melting while warming the atmosphere (AMAP, 2015[3]). In recent decades, BC deposition alone has accounted for 0.5 to 1°C of warming in the Arctic (Bice et al., 2009[28]). A recent study of Greenlandic snow cover suggests that snow albedo might decrease by over 10% by the end of the century (Tedesco et al., 2016[29]).
Nonetheless, black carbon is co-emitted and can interact with other pollutants, some of which – such as sulphur dioxide (SO2) – can have a counterbalancing cooling effect (AMAP, 2015[3]; Smith et al., 2020[30]). In some instances, black carbon itself can lead to a slight cooling effect, as BC particles facilitate cloud condensation, thus enhancing cloud reflectivity. Since BC emissions are always accompanied by cooling effects, climate modelling studies have shown that the climate mitigation potential of BC is relatively small and highly uncertain (Kühn et al., 2020[31]).
Despite these uncertainties, the possible impacts of black carbon on the Arctic climate represent a major threat in and beyond the Arctic region. Arctic warming accelerates sea level rise and interferes with the water cycle, increasing the risk of floods and droughts and affecting water availability in other regions of the world. For this reason, and given the multifaceted effects of black carbon pollution, policies targeting BC have the advantage of addressing the challenge of climate change and air pollution simultaneously.
Besides affecting human health, air pollution also has adverse effects on plant development and health. Ground-level ozone is absorbed by leaves, where it induces cell death and reduces physiological functions. Thus, high concentrations of ground-level ozone lead to slower growth, reduced flowering and lower seed production, hindering crop yield and quality (Latha et al., 2017[32]).
As a consequence, the biophysical impacts of outdoor air pollution entail substantial economic costs (OECD, 2016[2]; OECD, 2014[33]; Roy and Braathen, 2017[34]), which result from (1) losses in labour productivity; (2) additional health expenditures; (3) lower agricultural productivity; and (4) the welfare costs of air pollution-related mortality and suffering as a result of illness.
1.3. Anthropogenic sources of air pollution in Arctic Council countries
In the Arctic Council region, the largest contributors to the emission of black carbon and organic carbon – both components of primary PM2.5 – are the energy and industrial sectors, together with residential wood burning, and transport.7 While Arctic Council countries have set an aspirational emission reduction target for black carbon, substantial health benefits can only be achieved if other pollutants are also reduced. Specifically, reducing emissions of total primary PM2.5 and of other air pollutants that contribute to the formation of secondary PM2.5 is necessary to reduce PM2.5 pollution, which is the main driver of the health impacts of air pollution (Box 1.2). Abating emissions of gases which form secondary PM2.5 (precursor gases), such as sulphur dioxide, nitrogen oxides, ammonia, and non-methane volatile organic compounds, could further contribute to reducing the concentration of fine particles in the atmosphere.
Box 1.2. What are primary and secondary pollutants?
Pollutants can be classified into primary and secondary pollutants depending on how they are generated. Primary pollutants, such as carbon monoxide and sulphur dioxide, are the direct by-product of human activities. Secondary pollutants, such as ground-level ozone, are formed when primary (or precursor) pollutants react with other elements in the atmosphere. Some pollutants, such as NOX and NMVOCs, are precursor gases of both ground-level ozone and PM2.5.
PM2.5 is both a primary and secondary pollutant. Therefore, both primary and secondary PM2.5 are taken into account in air pollution measurements. Primary PM2.5 is emitted directly into the atmosphere by activities such as the combustion of fossil fuels and road transport. Particles composing primary PM2.5 include organic carbon and black carbon. Secondary PM2.5 is generated when PM2.5 precursors such as sulphur dioxide, nitrogen oxides, volatile organic compounds and ammonia react with other elements in the atmosphere. This process can take place far away from the original emission source (Hodan and Barnard, 2004[35]).
Ground-level ozone is a secondary pollutant, as it is not directly emitted into the atmosphere. Ground-level ozone is an example of photochemical oxidants, i.e. it is formed from the interaction between sunlight and precursor gases – mainly volatile organic compounds, nitrogen oxides, carbon monoxide and methane (CH4) (Unger et al., 2006[36]).
Meteorological and climatic conditions, such as atmospheric temperature, play a role in the chemical interactions occurring in the atmosphere (Lam et al., 2011[37]). For example, a rise in global temperatures could result in higher concentrations of ground-level ozone and secondary PM2.5. Other climatic factors, such as the frequency of precipitation and the presence of sunlight, can also influence the formation of secondary pollutants. For instance, the higher levels of sunlight in the summer months facilitate the formation of ground-level ozone.
Transport is an important source of air pollution in Arctic Council countries. It contributes significantly to the formation of PM2.5 and ground-level ozone. According to estimates of IIASA’s GAINS model, in 2015 transport accounts for around 70% of CO emissions, half8 of NOX and BC emissions and for one-quarter of OC and NMVOCs emissions in the region (Figure 1.1).
The industry and energy sectors are other key sources of pollution in Arctic Council countries. Together, energy production and consumption and the industrial sector are responsible for nearly all SO2 emissions and one-third of NMVOCs and NOX emissions in the region. These sectors are also responsible for a significant share of BC (18%) and OC (6%) emissions (Figure 1.1). Flaring9 alone represents 7% of total anthropogenic NMVOCs and 14% of black carbon emissions.
The residential sector is a key contributor to the formation of primary and secondary PM2.5 and ground-level ozone. In particular, the combustion of solid and liquid fuels for domestic heating is a major source of organic carbon (40%) and black carbon (over 20%), while also contributing to NMVOCs (10%), NOX (5%) and SO2 (3%) emissions in the region (Figure 1.1).
Finally, the agricultural sector is a major contributor of NH3 (92%), and it also has an important role in the emission of NMVOCs (27%) and OC (20%) (Figure 1.1). Agricultural fires,10 which are often used to dispose of crop residues, are responsible for nearly all the OC, NMVOCs and BC emissions from the sector and for 70% of primary PM2.5 emissions in the region.
Figure 1.1. Emissions of air pollutants in Arctic Council countries
Sectoral shares in 2015

Note: “Residential” emissions are due to wood and fuel burning. “Other” includes emissions from the waste sector (except agricultural waste, which is included in “Agriculture”). In this figure, PM2.5 refers to primary emissions only.
Source: IIASA’s GAINS model.
Although ground-level ozone is not directly emitted to the atmosphere, human activities contribute to the emission of its precursor gases, which include methane, NMVOCs, NOX, and CO. In Arctic Council countries, the transport, industry and power generation sectors emit large shares of these pollutants (Figure 1.1). Most notably, emissions of methane and CO, which are particularly relevant to ground-level ozone formation, are largely driven by industry and energy-related emissions and by transport emissions.
Other emission sources in the Arctic Council region – such as shipping and gas and oil flaring – are only included as part of aggregate emissions of Arctic Council countries, as their projections are characterised by high levels of uncertainty that did not allow emissions to be distinguished by country. Shipping activities in the Arctic are projected to keep growing, potentially quadrupling by 2050 (AMAP, 2015[3]) as reduced ice cover will open new shipping routes and opportunities across the region’s seas. However, navigating in Arctic waters implies significant costs, which might reduce the net economic gains of shorter transit routes (ITF, 2019[38]), thus making the sector’s development in the region rather uncertain. Emissions from flaring activities are also subject to uncertainty due to the variation in gas and oil composition and in flaring operating practices (Huang and Fu, 2016[39]; Conrad and Johnson, 2017[40]; Conrad and Johnson, 2017[40]; Klimont et al., 2017[15]; Stohl et al., 2013[41]).11 Flaring inventories and projections are particularly uncertain for key emitters, such as Russia, due to the lack of detailed activity data for a wide array of sources, and of a country-specific emission factor12 (Böttcher et al., forthcoming[42]).
Finally, emissions from wildfires – which are also highly uncertain – are not included in the emission projections presented in this report, although they are accounted for in the calculations of pollutant concentrations (Section 3.2). Wildfires are an important and increasing source of several pollutants (van Marle et al., 2017[43]) that also contribute to global warming, including black carbon. In Europe and North America, the frequency of wildfires has increased in recent years, and wildfire locations have changed too, moving towards more northern latitudes. Climate change is estimated to have a considerable impact on this trend. Indeed, increasing temperatures and changes in precipitation patterns leading to arid conditions and lower soil moisture facilitate the development and spread of forest fires (Arctic Council, 2019[4]).
References
[12] Amann, M. et al. (2011), “Cost-effective control of air quality and greenhouse gases in Europe: Modeling and policy applications”, Environmental Modelling & Software, Vol. 26/12, pp. 1489-1501, http://dx.doi.org/10.1016/j.envsoft.2011.07.012.
[8] Amann, M. et al. (2017), Costs, Benefits and Economic Impacts of the EU Clean Air Strategy and their Implications on Innovation and Competitiveness, International Institute for Applied Systems Analysis (IIASA).
[5] Amann, M. et al. (2020), “Reducing global air pollution: the scope for further policy interventions”, Philosophical Transactions of the Royal Society A: Mathematical, Physical and Engineering Sciences, Vol. 378/2183, p. 20190331, http://dx.doi.org/10.1098/rsta.2019.0331.
[45] Amann, M., Z. Klimont and F. Wagner (2013), “Regional and global emissions of air pollutants: recent trends and future scenarios”, Annual Review of Environment and Resources, Vol. 38/1, pp. 31-55, http://dx.doi.org/10.1146/annurev-environ-052912-173303.
[26] AMAP (2019), Review of Reporting Systems for National Black Carbon Emissions Inventories, AMAP, Tromsø, https://www.amap.no/documents/doc/eua-bca-technical-report-2/1780 (accessed on 16 December 2019).
[3] AMAP (2015), Summary for Policy-makers: Arctic Climate Issues 2015, AMAP, Tromsø, https://www.amap.no/documents/doc/summary-for-policy-makers-arctic-climate-issues-2015/1196 (accessed on 4 December 2020).
[18] Arctic Council (2021), Arctic Council - Observers, https://arctic-council.org/en/about/observers/ (accessed on 10 March 2021).
[4] Arctic Council (2019), Expert Group on Black Carbon and Methane - Summary of Progress and Reccomendations, https://oaarchive.arctic-council.org/handle/11374/2411 (accessed on 9 March 2021).
[28] Bice, K. et al. (2009), Black Carbon: A Review and Policy Recommendations, Princeton University, https://www.princeton.edu/~mauzeral/WWS591e/Princeton.WWS591E.Black.Carbon.report.2009.pdf.
[23] Bond, T. et al. (2013), “Bounding the Role of Black Carbon in the Climate System: A Scientific Assessment”, Journal of Geophysical Research Atmospheres, Vol. 118/11, pp. 5380-5552, http://dx.doi.org/10.1002/jgrd.50171.
[42] Böttcher, K. et al. (forthcoming), “Black carbon emissions from flaring in Russia in the period 2012-2017”.
[27] CCAC and WHO (2015), Reducing Global Health Risks Through Mitigation of Short-lived Climate Pollutants: Scoping report for policymakers, World Health Organization, Geneva, https://www.who.int/phe/publications/climate-reducing-health-risks/en/.
[11] Chateau, J., R. Dellink and E. Lanzi (2014), “An Overview of the OECD ENV-Linkages Model: Version 3”, OECD Environment Working Papers, No. 65, OECD Publishing, Paris, https://dx.doi.org/10.1787/5jz2qck2b2vd-en.
[40] Conrad, B. and M. Johnson (2017), “Field Measurements of Black Carbon Yields from Gas Flaring”, Environmental Science & Technology, Vol. 51/3, pp. 1893-1900, http://dx.doi.org/10.1021/acs.est.6b03690.
[10] Dechezleprêtre, A., N. Rivers and B. Stadler (2019), “The economic cost of air pollution: Evidence from Europe”, OECD Economics Department Working Papers, No. 1584, OECD Publishing, Paris, https://dx.doi.org/10.1787/56119490-en.
[17] EPA (2012), Report to Congress on Black Carbon, United States Environmental Protection Agency, https://www3.epa.gov/airquality/blackcarbon/2012report/fullreport.pdf.
[1] GBD (2018), Global Burden of Disease Study 2017 (GBD 2017) Burden by Risk 1990-2017, Global Burden of Disease Collaborative Network., Seattle, United States: Institute for Health Metrics and Evaluation (IHME), http://ghdx.healthdata.org/record/ihme-data/gbd-2017-burden-risk-1990-2017 (accessed on 25 March 2021).
[21] GBD (2018), “Global Burden of Disease Study 2017: All cause Mortality and Life Expectancy 1950-2017, Global Burden of Disease Collaborative Network.”, Seattle, United States: Institute for Health Metrics and Evaluation (IHME).
[6] Harmsen, M. et al. (2020), “Co-benefits of black carbon mitigation for climate and air quality”, Climatic Change, http://dx.doi.org/10.1007/s10584-020-02800-8.
[35] Hodan, W. and W. Barnard (2004), “Evaluating the Contribution of PM2.5 Precursor Gases and Re-entrained Road Emissions to Mobile Source PM2.5 Particulate Matter Emissions Prepared by MACTEC Under Contract to the Federal Highway Administration”, MACTEC Federal Programs, Research Triangle Park, NC..
[13] Höglund-Isaksson, L. et al. (2020), “Technical potentials and costs for reducing global anthropogenic methane emissions in the 2050 timeframe –results from the GAINS model”, Environmental Research Communications, Vol. 2/2, p. 025004, http://dx.doi.org/10.1088/2515-7620/ab7457.
[20] Holland, M. (2014), Cost-benefit Analysis of Final Policy Scenarios for the EU Clean Air Package, http://ec.europa.eu/environment/air/pdf/TSAP%20CBA.pdf (accessed on 10 January 2018).
[39] Huang, K. and J. Fu (2016), “A Global Gas Flaring Black Carbon Emission Rate Dataset from 1994 to 2012”, Scientific Data, http://dx.doi.org/10.1038/sdata.2016.104.
[46] IEA (2018), World Energy Outlook 2018, International Energy Agency, Paris, https://dx.doi.org/10.1787/weo-2018-en.
[38] ITF (2019), ITF Transport Outlook 2019, OECD Publishing, Paris, https://dx.doi.org/10.1787/transp_outlook-en-2019-en.
[15] Klimont, Z. et al. (2017), “Global Anthropogenic Emissions of Particulate Matter Including Black Carbon”, Atmospheric Chemistry and Physics, Vol. 17/14, pp. 8681-8723, http://dx.doi.org/10.5194/acp-17-8681-2017.
[31] Kühn, T. et al. (2020), “Effects of black carbon mitigation on Arctic climate”, Atmospheric Chemistry and Physics, Vol. 20/9, pp. 5527-5546, http://dx.doi.org/10.5194/acp-20-5527-2020.
[37] Lam, Y. et al. (2011), “Impacts of future climate change and effects of biogenic emissions on surface ozone and particulate matter concentrations in the United States”, Atmospheric Chemistry and Physics, Vol. 11/10, pp. 4789-4806, http://dx.doi.org/10.5194/acp-11-4789-2011.
[32] Latha, R. et al. (2017), “Absorbing Aerosols, Possible Implication to Crop Yield - A Comparison between IGB Stations”, Aerosol and Air Quality Research, Vol. 17/3, pp. 693-705, http://dx.doi.org/10.4209/aaqr.2016.02.0054.
[7] Markandya, A. et al. (2018), “Health Co-benefits from Air Pollution and Mitigation Costs of the Paris Agreement: A Modelling Study”, Lancet Planet Health, Vol. 2, pp. 126-33, https://doi.org/10.1016/S2542-5196(18)30029-9.
[25] Moffet, R. and K. Prather (2009), “In-situ Measurements of the Mixing State and Optical Properties of Soot with Implications for Radiative Forcing Estimates”, Proceedings of the National Academy of Sciences of the United States of America, Vol. 106/29, pp. 11872-11877, http://dx.doi.org/10.1073/pnas.0900040106.
[2] OECD (2016), The Economic Consequences of Outdoor Air Pollution, OECD Publishing, Paris, https://dx.doi.org/10.1787/9789264257474-en.
[33] OECD (2014), The Cost of Air Pollution: Health Impacts of Road Transport, OECD Publishing, Paris, https://dx.doi.org/10.1787/9789264210448-en.
[44] Rao, S. et al. (2016), “A multi-model assessment of the co-benefits of climate mitigation for global air quality”, Environmental Research Letters, Vol. 11/12, p. 124013, http://dx.doi.org/10.1088/1748-9326/11/12/124013.
[34] Roy, R. and N. Braathen (2017), “The Rising Cost of Ambient Air Pollution Thus Far in the 21st Century: Results from the BRIICS and the OECD Countries”, OECD Environment Working Papers, No. 124, OECD Publishing, Paris, https://doi.org/10.1787/19970900 (accessed on 26 April 2019).
[24] Shrestha, G., S. Traina and C. Swanston (2010), “Black Carbon’s Properties and Role in the Environment: A Comprehensive Review”, Sustainability, Vol. 2, pp. 294-320, http://dx.doi.org/10.3390/su2010294.
[30] Smith, S. et al. (2020), “Impact of methane and black carbon mitigation on forcing and temperature: a multi-model scenario analysis”, Climatic Change, http://dx.doi.org/10.1007/s10584-020-02794-3.
[41] Stohl, A. et al. (2013), “Black carbon in the Arctic: the underestimated role of gas flaring and residential combustion emissions”, Atmospheric Chemistry and Physics, Vol. 13/17, pp. 8833-8855, http://dx.doi.org/10.5194/acp-13-8833-2013.
[29] Tedesco, M. et al. (2016), “The darkening of the Greenland ice sheet: trends, drivers, and projections (1981–2100)”, The Cryosphere, Vol. 10/2, pp. 477-496, http://dx.doi.org/10.5194/tc-10-477-2016.
[36] Unger, N. et al. (2006), “Cross influences of ozone and sulfate precursor emissions changes on air quality and climate”, Proceedings of the National Academy of Sciences of the United States of America, Vol. 103/12, pp. 4377-4380, http://dx.doi.org/10.1073/pnas.0508769103.
[16] Van Dingenen, R. et al. (2018), “TM5-FASST: A Global Atmospheric Source–receptor Model for Rapid Impact Analysis of Emission Changes on Air Quality and Short-lived Climate Pollutants”, Atmospheric Chemistry and Physics, Vol. 18, pp. 16173-16211, https://doi.org/10.5194/acp-18-16173-2018.
[19] Van Dingenen, R. et al. (2009), “The global impact of ozone on agricultural crop yields under current and future air quality legislation”, Atmospheric Environment, Vol. 43/3, pp. 604-618, http://dx.doi.org/10.1016/j.atmosenv.2008.10.033.
[43] van Marle, M. et al. (2017), “Historic global biomass burning emissions for CMIP6 (BB4CMIP) based on merging satellite observations with proxies and fire models (1750–2015)”, Geoscientific Model Development, Vol. 10/9, pp. 3329-3357, http://dx.doi.org/10.5194/gmd-10-3329-2017.
[9] Vandyck, T. et al. (2018), “Air quality co-benefits for human health and agriculture counterbalance costs to meet Paris Agreement pledges”, Nature Communications, Vol. 9/1, http://dx.doi.org/10.1038/s41467-018-06885-9.
[14] Winiwarter, W. et al. (2018), “Technical opportunities to reduce global anthropogenic emissions of nitrous oxide”, Environmental Research Letters, Vol. 13/1, p. 014011, http://dx.doi.org/10.1088/1748-9326/aa9ec9.
[22] Yang, Y. et al. (2019), “Variability, Timescales, and Nonlinearity in Climate Responses to Black Carbon Emissions”, Atmospheric Chemistry and Physics, Vol. 19/4, pp. 2405-2420, http://dx.doi.org/10.5194/acp-19-2405-2019.
Notes
← 1. The 13 non-Arctic countries approved as Observers to the Arctic Council are France, Germany, Italy, Japan, the Netherlands, the People's Republic of China (hereafter China), Poland, India, Korea, Singapore, Spain, Switzerland, and the United Kingdom.
← 2. The climate and energy transition policies considered in this report are those included in the Sustainable Development Scenario (SDS) presented by the International Energy Agency (IEA) (IEA, 2018[46]).
← 3. While greenhouse gases are not directly targeted by the air pollution policies considered, this report also looks at methane (CH4) and carbon dioxide (CO2) emissions. This report does not include the long-range transport of mercury and persistent organic pollutants (POPs).
← 4. All scenarios based on inputs from the GAINS model were developed with the support of the European Union-funded Action on Black Carbon in the Arctic.
← 5. Chapter 6 in this report also discusses the specific impacts of SLCP emissions in more detail.
← 6. For simplicity, the report will refer to policy action to reduce black carbon as the collective target.
← 7. Regional estimates of pollutant emissions by sector are obtained using the GAINS model developed by IIASA (Rao et al., 2016[44]; Amann, Klimont and Wagner, 2013[45]; Amann et al., 2011[12]). All emission figures in this Chapter refer to 2015.
← 8. All the emission rates presented in this chapter refer to Arctic Council countries’ totals, unless otherwise specified.
← 9. Gas flaring is a combustion process that burns associated, unwanted or excess gases and liquids released during many industrial processes, such as oil and gas extraction or refineries.
← 10. Other types of fires, such as forest and grassland fires, represent major sources of air pollution but are not linked to the agricultural sector.
← 11. Gas and oil composition can vary substantially across different oil and gas fields, and is also severely affected by environmental conditions such as temperature and atmospheric pressure.
← 12. Country-specific emission factors allow to activity levels to be linked to emission levels at the national scale, rather than relying on regional or global averages.