This chapter takes stock of the vulnerabilities of medicine and medical device supply chains, making a case for the importance of enhancing their resilience. First, it provides insight into the complexity and variability of the organisation of these global supply chains and presents trade statistics. It then explores the growing issue of medical product shortages, which pre‑dated the COVID‑19 pandemic, including potential causes of disruption. Finally, the chapter discusses additional strain that is placed on supply chains in times of severe crises.
Securing Medical Supply Chains in a Post-Pandemic World

1. Vulnerabilities of medical supply chains
Abstract
Key findings
Medical supply chains are complex and have become increasingly internationalised over time. Supply chains, in the context of this report, refer to the flows of goods and services needed from production to distribution, and ultimately to final consumption (or use) of a medical product – medicine or medical device – by patients, health professionals or healthcare institutions.
The production of medicines involves complex transnational supply chains, generally beginning with raw materials that are transformed into active pharmaceutical ingredients (API) at primary manufacturing sites, with secondary sites producing the finished pharmaceutical products. Global trade in pharmaceuticals has increased 10‑fold over the past 30 years, and now accounts for about 4% of total trade by value. About half the movements of goods (by value) concerned intermediate inputs (such as APIs) in 2022. The share of traded intermediate inputs in the value of final pharmaceutical products peaked at 25% in 2015 and has been declining since.
Medical device supply chains are even more varied and, and in some cases more complex, than those of medicines, with some bearing a closer resemblance to supply chains for non-medical products such as clothing or electronics. Medical devices span a huge range of products, and their supply chains are highly product-dependent, with manufacturing reliant on a large number of suppliers of individual components that may be specific to individual devices or commonly used by non-medical manufacturers. Over the past 30 years, global trade in medical devices has increased 7‑fold in value, to reach a total amount of USD 700 billion in 2022, with one‑third in intermediate goods, one‑third in final products and about one‑third in capital goods (durable equipment).
Medicine shortages were already widespread prior to the COVID‑19 pandemic. Although shortage definitions and notification rules vary widely across countries and regions, multiple studies have reported steady increases in the prevalence of shortages in various contexts.
These studies show that shortages predominantly affect older, off-patent medicines. However, one study looking at 20 countries in the European Economic Area (EEA), found that these medicines do not necessarily have a higher probability of being in shortage. The most commonly affected medicine types varied across countries and periods. Central nervous system, cardiovascular and anti‑infectives medicines were among the most commonly affected classes, with injectables more likely to be in shortage than oral dosage forms.
Manufacturing and quality issues are by far the most frequently reported causes of medicines shortages (50‑60%). “Commercial reasons” are also often cited (25% in one study of 20 EEA countries). Market dynamics have been identified as an important root cause in the United States, where competitive pricing pressures on off-patent multi-source products can be very intense. For other countries, empirical evidence on root causes of shortages is lacking.
The contribution of the nature of distribution chains in local or national shortages has not been established empirically.
Some medicinal products face specific challenges arising from unique features of their supply chains. These include vaccines (which are subject to exceptionally rigorous requirements, notably with regard to quality controls and testing), plasma-derived medicines (dependent on plasma collection) and radio-pharmaceuticals (whose production costs are subsidised by manufacturing countries).
Prior to the pandemic, shortages of medical devices received less attention than shortages of medicines, likely due in part to differences in notification requirements. Experts and industry representatives have nevertheless identified several risks to the future supply of medical devices. These pertain to long-awaited reforms in medical device and in-vitro-diagnostic regulation in the European Union; competition with other larger industrial sectors for the acquisition of raw material and electronic components used as intermediate inputs; possible changes in the regulation of certain chemical substances; and, more recently, significant inflation in the costs of inputs. Data on the occurrence and evidence of the causes of shortages of medical devices and in-vitro diagnostics are, however, very scant.
The resilience of medical product supply chains in the face of severe crises has been tested on several occasions. For example, large surges in demand occurred with the H1N1 and COVID‑19 pandemics, and in the latter, these were coupled with significant disruptions in manufacturing and trade restrictions, together exacerbating the pre‑existing issues. Despite being severely stressed during these periods and facing several shortages, medical product supply chains demonstrated considerable resilience.
Chapter 1 takes stock of the vulnerabilities and particularities of medical product supply chains. Supply chains, in the context of this report, refer to the flows of goods and services needed from production to distribution, and ultimately to final consumption (or use) of a medicine or medical device – by patients, health professionals or healthcare institutions (Section 1.1). A supply chain failure is said to occur when supply is unable to meet demand for a product marketed in a given country. While shortages of medical goods were increasing in frequency prior to the pandemic of COVID‑19 (Section 1.2), the crisis demonstrated the critical importance of securing supply chains of medical goods to address future severe health crises (Section 1.3).
1.1. Understanding the complexity of medical product supply chains
Medical product supply chains are complex and often fragmented, with many different stakeholders involved globally. The term “medical products” itself encompasses a wide variety of items and substances used in healthcare, including pharmaceuticals (i.e. medicines), vaccines, medical devices (i.e. products or equipment intended for a medical purpose), biological products, blood and tissue products, diagnostic tools and tests, personal protective equipment (PPE), and medical consumables (e.g. disposable items such as blood collection tubes, syringes) etc. Medicines may also be used in combination with medical devices, further adding to the complexity. It is important to note that regulatory oversight, manufacturing processes, and safety standards for these products can vary widely depending on their type, intended purpose, and the level of risk they pose, as well as by jurisdiction. For example, while regulatory authorities authorise medicinal products (including medicines, vaccines, plasma products, etc.) by assessing their safety, efficacy, and quality they may have different and distinct regulatory responsibilities for medical devices.1 The sections below describe some of the specifics of supply chains of medicines (Section 1.1.1) and medical devices (Section 1.1.2) for human use.
1.1.1. Pharmaceutical supply chains are complex and internationalised
Figure 1.1 offers a basic schematic of pharmaceutical supply chains, as described by Chapman, Dedet and Lopert (2022[1]). The production of medicines involves complex transnational supply chains, with the organisation often driven by cost containment practices and the structures of production processes. They can involve multiple stakeholders across different facilities and countries. For example, small-molecule (non-biological)2 medicine production starts with raw materials transformed into active pharmaceutical ingredients (APIs) at primary (often specialised) sites, while secondary sites turn these APIs into finished pharmaceutical products (FPPs). Marketing authorisation holders (MAHs), i.e. the companies or legal entities with authorisation to market the products, often also use contract manufacturers. Medicines are then supplied by manufacturers to distributors (i.e. wholesalers) and to retail dispensing points, with hospitals in some places bypassing wholesalers and being supplied directly by MAHs. Disruptions in manufacturing and production processes may have a global impact on the availability of medicines, while problems in distribution processes are more likely to have localised effects (e.g. local or national).
Figure 1.1. The complexity of pharmaceutical supply chains
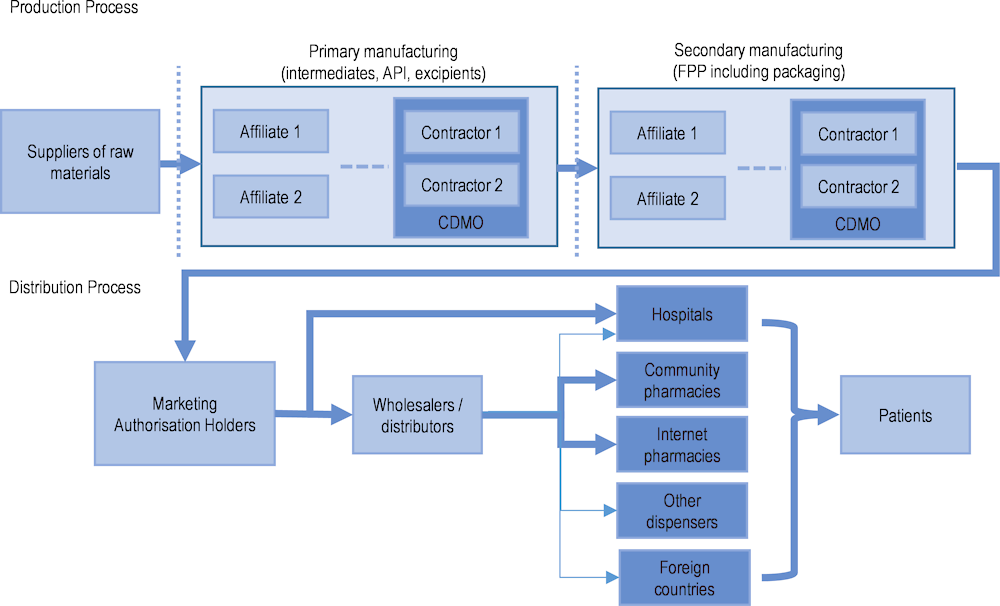
Note: Dotted vertical lines represent the possibility of an international border. API: active pharmaceutical ingredient; FPP: finished pharmaceutical product; CDMO: contract development and manufacturing organisation.
Source: Adapted from Chapman, S., G. Dedet and R. Lopert (2022[1]), “Shortages of medicines in OECD countries”, https://doi.org/10.1787/b5d9e15d-en.
Trade in pharmaceutical products has increased, especially for intermediate products
Since 1995, there has been a remarkable increase in trade in pharmaceutical products, with a notable surge in the second half of the 2010s, and a further acceleration following the COVID‑19 pandemic (Figure 1.2, Panel A). This can be attributed to several factors, including advancements in pharmaceutical research and development, and increased global demand for healthcare products. It is also explained by the expansion of global supply chains, as highlighted by the increasing share of trade in intermediate inputs (such as APIs) in Figure 1.2. In addition, the share of pharmaceutical products in total world trade by value has been steadily increasing (Figure 1.2, Panel B). This trend is indicative of the growing importance of the pharmaceutical industry in the global economy.
Higher values for trade in pharmaceutical products during the pandemic are partially explained by higher prices. However, they also illustrate how trade in pharmaceuticals outpaced the overall growth in merchandise trade in recent years, as well as the role played by trade to address pandemic-related disruptions and shortages. While there is some granularity in trade data,3 it remains challenging to analyse trade flows for specific products (such as face masks during COVID‑19) and trade statistics do not allow for a full analysis of supply chains when not coupled with input-output data.
Figure 1.2. World trade in pharmaceutical products (1995‑2022), by value
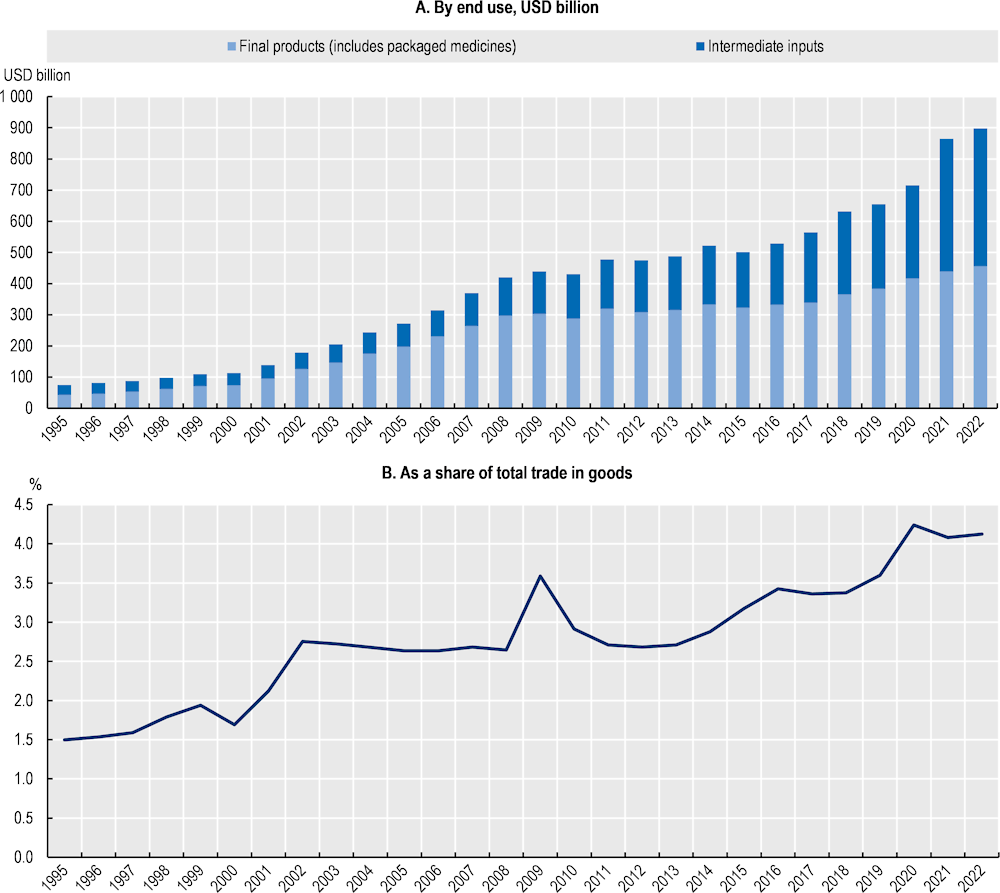
Note: Pharmaceutical products as defined by the WTO Pharma Agreement (HS Chapter 30, and headings 2936, 2937, 2939, 2941). Data prior to 2017 are based on older versions of the HS classification and may not accurately reflect the list of products.
Source: BACI (CEPII) (2023[2]), BACI Trade data, www.cepii.fr/CEPII/en/bdd_modele/bdd_modele_item.asp?id=37; and Drevinskas, E., E. Shing and T. Verbeet (2023[3]), Trade in medical goods stabilises after peaking during pandemic, www.wto.org/english/blogs_e/data_blog_e/blog_dta_23may23_e.htm.
Germany, Switzerland and the United States are the top exporters of pharmaceutical products by value, reflecting their strong positions in high-value, research-intensive pharmaceutical activities. In contrast, the People’s Republic of China (hereafter “China”) and India are among the top 3 exporters by volume, signifying their roles in the mass production of APIs and off-patent medicines (Figure 1.3). Germany stands out as a top exporter and importer in both volume and value, suggesting that the country plays a role upstream in pharmaceutical supply chains, while also being the largest consumer market in the European Union (EU). The United States stands as the top importer of pharmaceutical products in both volume and value, driven by its large consumer market and high per capita healthcare spending. Belgium and Switzerland have small domestic markets but are both major exporters and importers of pharmaceutical products (by value). This is another illustration of how pharmaceutical supply chains have become global with specialised economies acting as key hubs for the transformation and distribution of pharmaceutical products.
Figure 1.3. Top exporters and importers of pharmaceutical products (2021), by value and volume
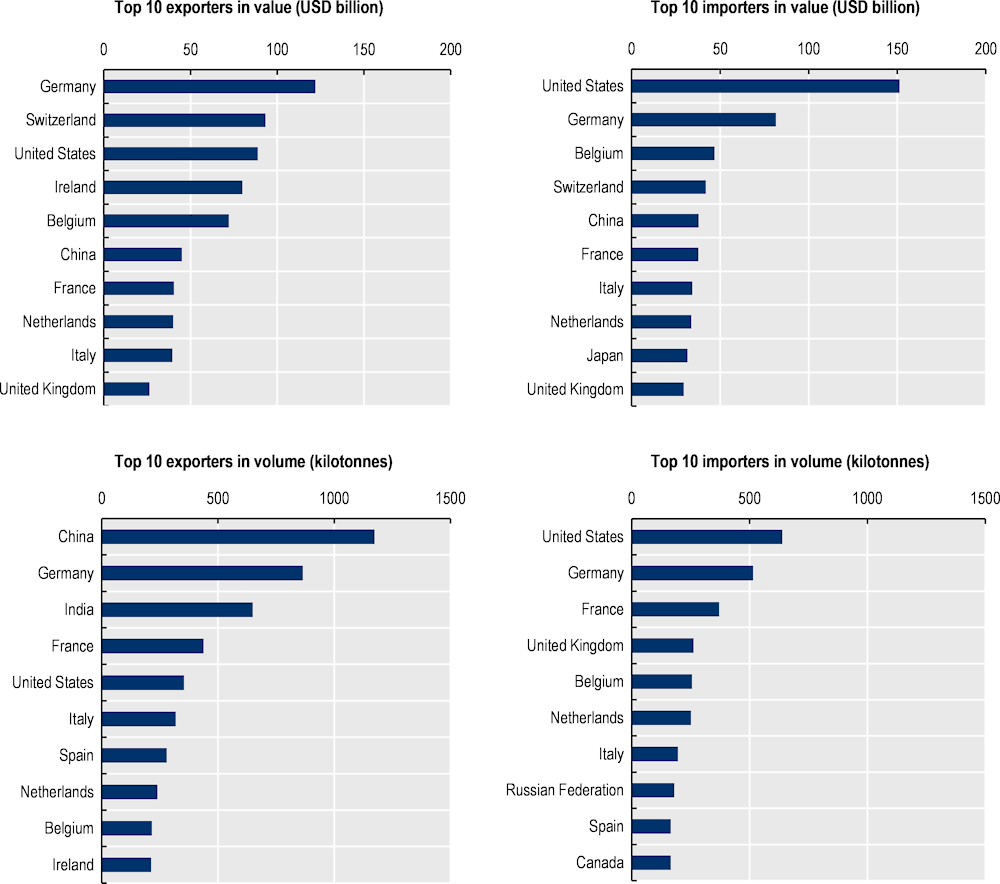
Note: Pharmaceutical products as defined by the WTO Pharma Agreement (HS Chapter 30, and headings 2936, 2937, 2939, 2941). Data prior to 2017 are based on older versions of the HS classification and may not accurately reflect the list of products.
Source: BACI (CEPII) (2023[2]), BACI Trade data, www.cepii.fr/CEPII/en/bdd_modele/bdd_modele_item.asp?id=37; and Drevinskas, E., E. Shing and T. Verbeet (2023[3]), Trade in medical goods stabilises after peaking during pandemic, www.wto.org/english/blogs_e/data_blog_e/blog_dta_23may23_e.htm.
Sourcing of pharmaceutical products has become increasingly internationalised
When using input-output data (such as the OECD Inter-Country Input-Output tables), the internationalisation of pharmaceutical supply chains becomes even clearer (Figure 1.4). These data lack any granularity beyond the specification of the pharmaceutical industry (identified through ISIC code 21 corresponding to “manufacture of basic pharmaceutical products and pharmaceutical preparations”). However, the data indicate that when looking at the world output of pharmaceutical products (i.e. all intermediate and final products produced by firms belonging to the pharmaceutical industry), the share of output corresponding to all the intermediate inputs traded upstream in their supply chains (from any country and industry) steadily increased between 1995 and 2015. In 1995, there were only 12 cents of trade intermediate inputs for each 1 dollar of output in the pharmaceutical industry; in 2015, there were more than 25 cents of trade in intermediate inputs (in constant prices).
Figure 1.4. Import intensity of pharmaceutical production (1995‑2020)
As a share of world gross output of pharmaceutical products (%), in value
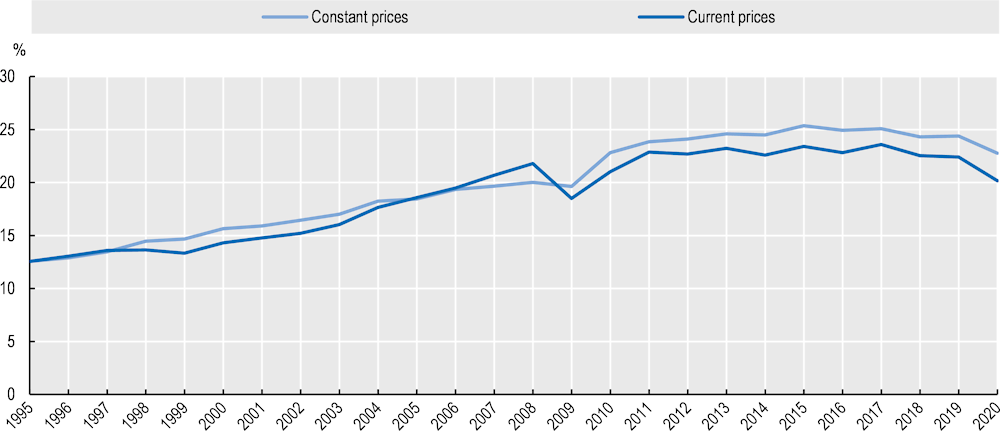
Note: The import intensity of production indicates for each dollar of final output in the pharmaceutical industry the share of value corresponding to all trade in intermediate inputs upstream in the value chain. Data for the world are estimated via an average weighted by final demand in each country and masks substantial heterogeneity across countries and products. Data in previous year’s prices.
Source: OECD (2023[4]), OECD Inter-Country Input-Output Database, http://oe.cd/icio.
From Figure 1.4 it can be seen that 2015 was the “peak year” in the globalisation of the pharmaceutical industry, with a trend towards more domestic supply chains in 2017‑20 (i.e. a lower share of traded intermediate inputs in final output). While this trend began prior to the pandemic, 2020 should be regarded as an exceptional year in which the import intensity of production was lower due to disruptions in international trade. Trade data post 2020 indicate an important surge in trade of pharmaceutical products (Figure 1.2) that may be associated with more foreign inputs trade and higher import intensity of production. It remains to be seen whether the pandemic has also triggered a restructuring of pharmaceutical supply chains with a re‑shoring of inputs manufacturing. Such a trend would take time to materialise into actual shifts in trade flows and a change in the input-output structure. Importantly, Figure 1.4 shows average figures that belie significant heterogeneity across buyers, and suppliers, as well as across products.
Some country and region-specific results are shown in Figure 1.5, highlighting not only a shift in the overall use of foreign inputs but also changes in the geographical distribution of suppliers. In the European Union, Japan and the United States, pharmaceutical products have been produced with a smaller share of domestic value‑added4 over the years (and there has been no decline in the import intensity of production for these economies). China is the only country in this sample for which pharmaceutical supply chains became more domestic between 2011 and 2019.
While as suppliers of inputs, China and India have benefited from the internationalisation of EU, Japanese and US supply chains, more foreign value added is actually coming from other OECD economies. Most of the increased foreign value‑added in US and Japanese pharmaceutical supply chains is the result of growth in sourcing in the EU, and in Switzerland, while EU supply chains rely more on Switzerland and North American suppliers (i.e. Canada, Mexico and the United States). There is an increase in the value added coming from China in EU, Swiss an US supply chains, but relatively small. While these results are for all pharmaceutical products, they are not inconsistent with more product-specific assessments identifying a high level of sourcing from India and China (but concentrated in certain off-patent medicines and specific APIs). In addition, data on the origin of value‑added in final consumption do not reflect the magnitude of countries’ contributions to final consumption in terms of quantities.
Figure 1.5. Origin of value added in final consumption of pharmaceutical products (1995, 2011 and 2019)
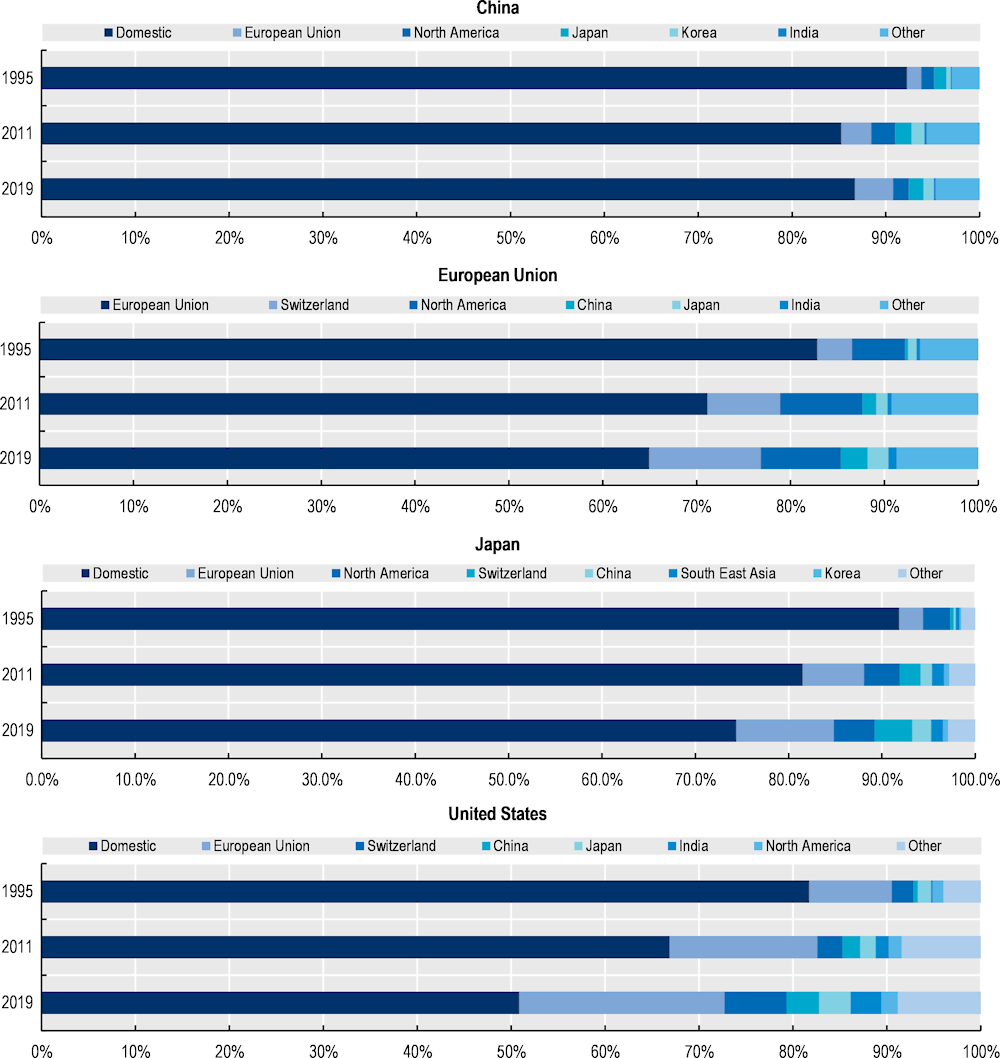
Note: Based on a decomposition of final demand for products of the pharmaceutical industry (ISIC code 21 – manufacture of basic pharmaceutical products and pharmaceutical preparations) identifying the country of origin of value added.
Source: OECD (2023[4]), OECD Inter-Country Input-Output Database, http://oe.cd/icio.
Distribution of medicines takes variable forms across countries
Post production, the distribution of medicines follows a range of different pathways, depending on the country, the type of medicine and the final intended use (administration in inpatient care, dispensing in retail pharmacies, etc.). Medicines dispensed by retail pharmacies to patients are generally supplied by wholesalers, subject to different regional or national regulations and obligations. Adapting to market conditions, wholesalers adopt a variety of organisational structures. In the United States, there are ~33 500 wholesale distributors and third-party logistics providers (FDA, 2023[5]). Across Europe, the number of wholesalers and warehouses vary significantly. For example, in 2021, Germany counted 9 full-line wholesalers5 with 106 warehouses, while Poland had 122 full-liner wholesalers with 190 warehouses. In Germany, 2 413 authorisations had been issued for wholesalers (including those who only distribute a sub-set of medicines) compared with 421 in Poland (GIRP, 2022[6]).6 In some cases, however, generic manufacturers may choose to bypass wholesalers and gain direct access to pharmacy shelves by offering financial or in-kind discounts or complementary services. The effect of this high variability in wholesale activity on reliability of supply is unknown.
In the EU, medicines dispensed or administered in hospitals are mainly purchased directly from manufacturers. In 2021, manufacturers delivered 7% of their products directly to retail pharmacies and 35% to hospitals, with the remainder 58% delivered to wholesalers for distribution to retail pharmacies (52%) and hospitals (6%) (GIRP, 2022[6]).7
Flows and practices in the distribution chains may lead to local shortages. Within the European Economic Area (EEA), so-called “parallel-trade” is often cited as a possible cause of national shortages. This type of trade consists of purchasing medicines in a country where (often regulated) prices are low, and reselling them in a country with higher prices, without the consent of the manufacturer. The practice is consistent with the principle of free movement of goods within the EEA and enables savings in recipient countries. According to the association of companies engaged in parallel trade, Affordable Medicines Europe, parallel trade imports accounted for 2.8% of the total EU pharmaceutical market in 2020. At that time, Germany, the United Kingdom and the Netherlands were the 3 top importers, with respectively 51%, 14% and 10% of EU parallel imports by value. Denmark, the Netherlands and Sweden had the highest shares of parallel imports in their national markets, with 25%, 10% and 10% respectively. According to members’ responses to a survey by the association, 50‑60% of imports in these countries originated from high-income countries. France and Germany were the largest exporters in terms of global sales (Aguiar and Ernest, 2021[7]).
Individual pharmaceutical product supply chains are unique: Some examples
While each pharmaceutical product supply chain is unique, some general insights can be gained from examining the supply chains of selected product categories. Chapter 11 of Ready for the Next Crisis? (OECD, 2023[8]) includes several detailed case studies:
Propofol (an intravenous anaesthetic) has a complex manufacturing process, requiring the API to be part of a stable emulsion (i.e. a mixture of two non-miscible substances such as oil and water). Propofol’s API (2,6-diisopropylphenol) has a relatively diversified supply in India, Italy, Switzerland and the United States, but the overall number of suppliers remains fewer than 10. Secondary manufacturing of propofol is a controlled and sterile process that involves creation of the emulsion and preparation of the final product. It is generally outsourced to either a subsidiary of the brand manufacturer or an independent contract manufacturer. Testing and packaging generally take place in locations separate from manufacturing. There are several manufacturers of propofol, but only a limited number are authorised to sell in each market.
Low-molecular-weight heparins (LMWH – a class of anticoagulants, with a case study based on the example of enoxaparin) are biologicals2 derived from unfractionated heparin, most of which originates from porcine (pig) intestines. China plays a crucial role as a producer, supplying 60% of the crude heparin utilised in the United States for the production of heparin sodium in 2010 (US Congress, 2018[9]). After purification in a laboratory, heparin extracts are transformed into heparin sodium, with capacity in China, Singapore and the United States. Subsequently, full length heparin is converted into smaller LMWH fragments through depolymerisation, a process requiring sophisticated techniques to ensure product stability and quality. This step is conducted by the brand manufacturer or a specialised contract manufacturing firm. Exports of heparin increased significantly during the COVID‑19 pandemic.
Macrolide antibiotics (a class of antibiotics, using the specific example of azithromycin) require specialised production processes that are currently highly concentrated. Fermentation to produce the intermediate ingredient, erythromycin, takes place in several geographical locations in China. This technique requires clean water, a favourable environment, and adequate infrastructure. Primary manufacturing of the azithromycin API from erythromycin requires several intermediate (chemical) steps that can be split across countries and companies, although interviewees suggested that this step generally occurs in Asia. The formulation stage is more geographically diverse. However, few companies market azithromycin.
The OECD has also analysed the supply chains of plasma-derived medicines and vaccines, both of which are classified as biologics.2 Box 1.1 and Box 1.2 outline some of the supply chain characteristics of these products.
Box 1.1. Plasma-derived medicinal product supply chains at a glance
Plasma-derived medicinal products (PDMPs) are essential for preventing and treating a range of conditions that include immune deficiencies, autoimmune and inflammatory conditions, and bleeding disorders, and they are also indicated in certain infectious diseases and critical situations such as septic shock and severe burns (Strengers, 2023[10]; Strengers, 2017[11]; Brand et al., 2021[12]; Schmidt and Refaai, 2022[13]). They are classified as biologicals, manufactured from human blood plasma through a process called fractionation. This technique involves separating, purifying, and concentrating different types of proteins found in blood plasma into therapeutic doses (see Figure 1.6). A range of PDMPs serve critical functions in healthcare, including in particular albumin (the major plasma protein responsible for regulating blood volume), coagulation factors (essential for blood clotting, used to treat genetic bleeding disorders and surgical bleeding) and immunoglobulins (essential for defence against infectious agents and the regulation of the immune system). Currently around 20 different therapeutic proteins can be purified from plasma, and PDMPs are licensed for the treatment of many diseases and disorders (Schmidt and Refaai, 2022[13]). In most jurisdictions, PDMPs are regulated as prescription medicines, subject to particularly rigorous regulation, testing, and controls. Their importance is underscored by the inclusion of several PDMPs in the World Health Organization’s Model List of Essential Medicines (WHO, 2023[14]).
The PDMP manufacturing process starts with the collection of blood plasma from healthy donors, which is a resource of human origin (PPTA, 2022[15]). Plasma can be obtained either through whole blood donation (recovered plasma) or directly by apheresis (plasmapheresis or sourced plasma). Most PDMPs are primarily sourced from plasmapheresis, a method by which whole blood is collected and centrifuged, plasma separated, and red blood cells returned to the donor (Strengers, 2023[10]). Compared to whole blood donation, which only takes 10‑20min, plasmapheresis is a longer and more laborious process, taking around 60‑90 min, but is more efficient as it generates two to three times more plasma per donation.
Figure 1.6. Schematic of plasma manufacturing process and supply chain
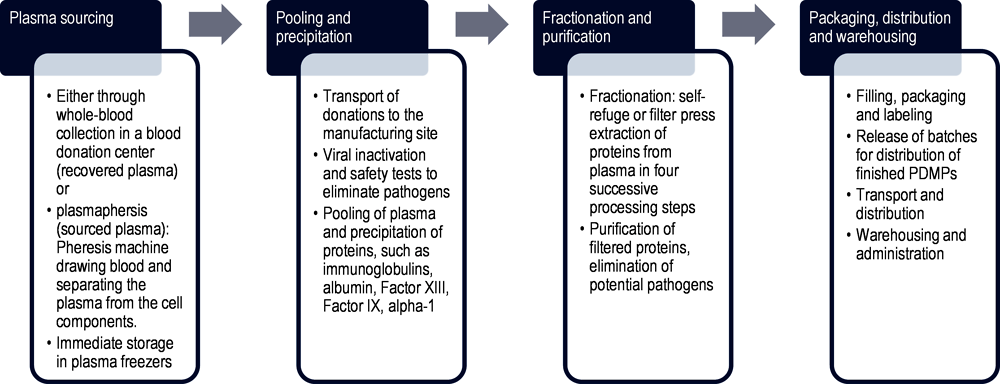
Note: The stages depicted in this schematic are intended to provide a general overview of plasma production and are not comprehensive.
Source: Authors’ elaboration based on Kluszczynski, T., S. Rohr and R. Ernst (2020[16]), Key economic and value considerations for plasma-derived medicinal products (PDMPs) in Europe.
As the sourced plasma must conform to the highest safety standards, it is subject to viral removal processes, pathogen elimination, and multiple inactivation steps. In a second step, multiple donations are pooled in large manufacturing vessels. As depicted in Figure 1.6, proteins are first precipitated from the plasma. Each plasma contains valuable proteins that are extracted, such as immunoglobulins, albumin, Factor VIII, Factor IX, alpha‑1 antitrypsin, and many more (Kluszczynski, Rohr and Ernst, 2020[16]). The harvest is obtained either through self-refuge of the plasma while the proteins are in motion or through filter press extraction. This process is also described as fractionation as it separates the plasma into four successive processing steps, so-called fractions, where different protein types are obtained for the final product (Strengers, 2023[10]). In the case of immunoglobulins, the plasma pool has to be large and geographically diverse, containing at least a thousand donations that in some jurisdictions may be sourced from all over the world (Kluszczynski, Rohr and Ernst, 2020[16]). Geographic diversity is important to ensure that the final product contains a wide spectrum of antibodies to fight against various pathogens. The filtered proteins are then purified, and potential pathogens eliminated before they are filled, packed and batches of finished PDMPs released for distribution.
The demand for PDMPs, such as intravenous immunoglobulins (IVIg), which are polyvalent and without an alternative or recombinant version, is growing at a rate of 6‑8% per year globally, likely due to expanded access to medical care, the development of new products and advanced diagnostics (Schmidt and Refaai, 2022[13]; Strengers, 2023[10])1. Immunoglobulins are essential in the body’s immune defence against foreign agents such as viruses and bacteria. Furthermore, the increasing number of patients with immunodeficiencies caused by oncology treatments and the growth in off-label use of IVIg are contributing to the increasing demand (correspondence with experts, 2023; (Schmidt and Refaai, 2022[13])).
Despite decades of effective therapeutic use, these treatments still face serious patient access challenges due to an uneven plasma donation landscape across countries, lengthy manufacturing processes taking up to 7‑12 months under strict safety procedures, and complex regulatory frameworks that impede the collection and manufacturing of plasma (Kluszczynski, Rohr and Ernst, 2020[16]). The key challenges appear upstream in the value chain, beginning with the collection of the raw material, i.e. plasma, which can only be sourced from eligible and healthy human donors.
1. Latest research demonstrates that consumption in Europe alone is projected to increase by one‑third from 50.5 tonnes in 2017 to 67.5 tonnes in 2025 (Marketing Research Bureau, 2023[17]). The plasma collected in this region meets 63% of the demand and the rest is mainly supplied by the United States (Kluszczynski, Rohr and Ernst, 2020[16]).
Source: Authors as cited and from consultations with experts in 2023.
Box 1.2. Vaccine supply chains at a glance
Vaccines are biological medicines intended to stimulate immunity to a particular infectious disease or pathogen and may be deployed as part of population-based immunisation strategies as well as in response to seasonal and emergency outbreaks. Although the precise stages and inputs needed for the production of a given vaccine vary depending on the technology platform (e.g. inactivated vaccine, live attenuated vaccine, viral vector-based, recombinant protein, messenger ribonucleic acids (mRNA) etc.), vaccine supply chains can be broadly described as in Figure 1.7.
Figure 1.7. Schematic of vaccine manufacturing process and supply chain
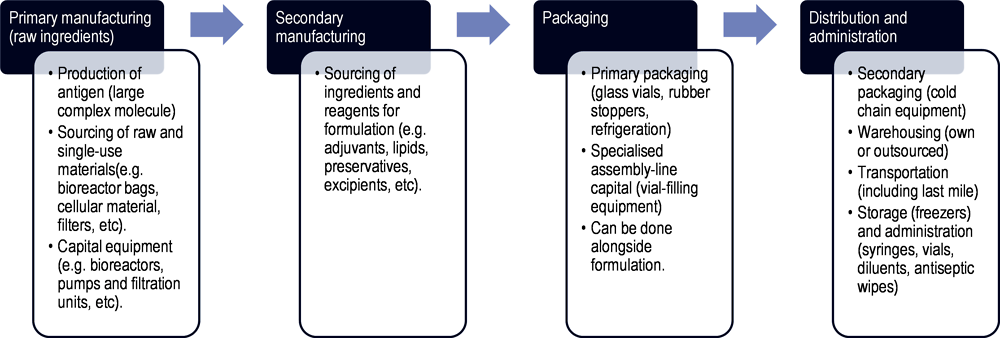
Note: The stages depicted in this schematic intend to provide a general overview of vaccine production and are not comprehensive.
Source: Author’s own elaboration based on OECD (2021[18]), Using trade to fight COVID‑19: Manufacturing and distributing vaccines, https://doi.org/10.1787/dc0d37fc-en; Bown, C. and T. Bollyky (2021[19]), “How COVID‑19 vaccine supply chains emerged in the midst of a pandemic”, https://doi.org/10.1111/TWEC.13183; OECD (2023[8]), Ready for the Next Crisis? Investing in Health System Resilience, https://doi.org/10.1787/1e53cf80-en.
Primary manufacturing consists of the initial production steps to create the vaccine’s active ingredient i.e. the antigen, which is responsible for inducing an immune response. The process and type of production facility needed for this vary according to the type of vaccine being produced. Typically, it includes culturing and propagating the target organism (e.g. virus or bacteria) in bioreactors, inactivating or attenuating the pathogen, and purifying the antigenic components – to create what is often known as “bulk antigen” or “bulk vaccine”.
Secondary manufacturing involves the formulation of the vaccine, by combining the vaccine’s active ingredient with all other components and mixing them uniformly in a single vessel. Here, stabilisers, adjuvants, and preservatives may be added. Additional ingredients in the production or packaging of a vaccine may require separate mini supply chains.
For packaging, vaccine formulations are transferred to a separate facility in order to “fill” (squirt doses into vials) and “finish” (cap the vials with stoppers and then label and package) the vaccine. This requires specialised assembly-line capital equipment, in addition to inputs such as glass vials and stoppers. In some cases, the formulation and fill and finish take place in the same facility. Stringent quality controls are taken at this stage.
Finally, vaccines doses must be transported at appropriate temperatures, and delivered while maintaining the cold chain. The cold chain is interconnected with refrigeration equipment; while most vaccines can be kept between 2°C and 8°C, some require temperatures as low as ‑20°C or ‑70°C.
The three main manufacturing stages described above can take place in different factory buildings, as well as across several countries. Each step also involves rigorous quality controls and testing, which represent up to 70% of the manufacturing time (Vaccines Europe, 2020[20]). On average, the entire manufacturing process is long and can take up to two years, with differences according to the technology platform.
According to the World Health Organization’s 2022 Global Vaccine Market Report, the vaccine supply base is highly concentrated geographically and at firm level (WHO, 2023[21]). In 2019, an estimated 76% of vaccine production took place in Europe, followed by North America (13%), Asia (8%) and the rest of the world (3%) (Vaccines Europe, 2019[22]). In 2021, excluding COVID‑19 vaccines, 10 manufacturers alone provided 71% of vaccine doses globally. When looking at individual vaccines, often only two or three suppliers provide more than 80% of supply (WHO, 2023[21]).
The geographical concentration of the manufacturing base underscores the role of trade. As of 2019, vaccines were imported by 209 economies and exported by 90 economies. Cao, Du and Xia (2023[23]) found that vaccine trade links remain highly concentrated within developed countries in Europe and the United States, a pattern largely in line with the WHO’s assessment (WHO, 2023[21]). In 2021, the EU was the largest exporter of vaccines, with Belgium as the top exporter by both value and volume, accounting for 16% of global volume exports, followed by the United States (14%) and China (12%)1. Export volume rankings differ from value rankings, revealing heterogeneity in unit prices across suppliers. In relative terms, imports are less concentrated in both value and volume, although the top 20 importers represent 52% of global import volumes (72% in value)1.
As mentioned above, production of vaccines relies on several ingredients. For example, the manufacture of pertussis-containing pentavalent vaccine requires approximately 160 different ingredients. Using 2017‑19 UN COMEXT trade data for 20 vaccine ingredients and items needed to distribute vaccines, Evenett et al. (2021[24]) found that the EU was a net importer of only three vaccine ingredients and distribution items. China and the United States were identified as key non-EU sources of vaccine inputs, followed by Switzerland and Japan (Evenett et al., 2021[24]).
1. OECD calculations using trade data from the BACI database, 2023.
Source: Authors as cited and from consultations with experts in 2023.
1.1.2. Medical device supply chains exhibit even more variability
Medical device supply chains exhibit even more variability, and in some cases more complexity, than pharmaceuticals, with some bearing a closer resemblance to supply chains for non-medical products such as clothing or electronics. According to the WHO (2023[25]), medical devices “include all the health technologies (except for vaccines and medicines) required for prevention, diagnosis, treatment, monitoring, and palliation”; countries also have their own definitions. Such broad definitions mean that medical devices cover a huge range of goods, from simple tongue depressors to complex ventilators with many associated parts (e.g. semi-conductors, other bespoke consumables). Medical device manufacturing is highly product-dependent, and a linear process flow is not adequate to capture their complexity. Like medicines, there may be multiple steps in several countries, however, some components of medical devices (e.g. chips) may be produced for both health and non-healthcare markets (OECD, 2023[8]). The perceived simplicity of a product may belie complexity in its supply chain.
Figure 1.8 depicts a basic schematic of a supply chain for a medical device, based on Chen et al.’s (2021[26]) analysis in a 2021 report prepared for the United States Government. Manufacturers source individual components or devices from the suppliers who produce them, who in turn source individual components or parts (raw materials) to make certain components from other suppliers, and so on. The number of suppliers can be vast, and suppliers also provide many of these same components to non-medical manufacturers (e.g. for electronics). However, there are instances in which components (e.g. a pressure regulator) are specific to a particular medical device (e.g. a ventilator), and there may be limited sources for these components. Once the manufacturer assembles the final product, it may require sterilisation (often by a contract steriliser, which is another type of supplier), before being ready to be sold to customers. Customers vary according to the type of device, but can include hospitals, physician practices, pharmacies (where patients may purchase the product directly), other medical supply stores, or even consumers in other non-health industries such as mining and construction. Hospitals typically purchase either from distributors (i.e. wholesalers) or directly from manufacturers. In some countries, group purchasing organisations (GPOs) may play a role in the movement of goods from manufacturers to hospitals and be involved in the purchasing process. Some medical devices also require maintenance and repair through various types of contracts; this involves additional components, services and providers.
Figure 1.8. The complexity of medical device supply chains
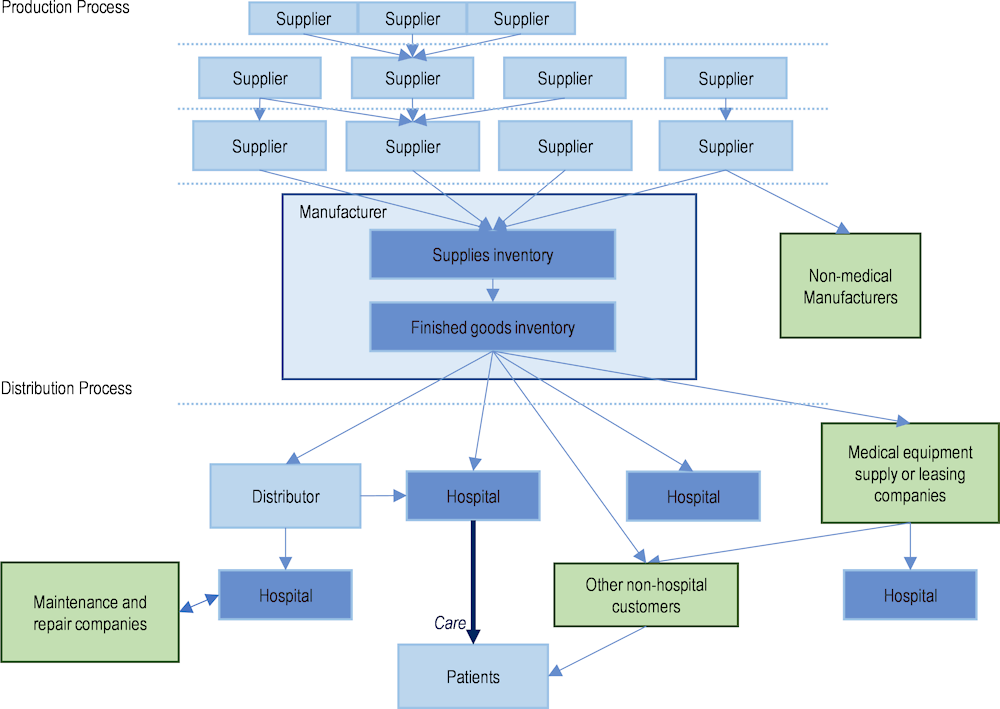
Note: Solid blue arrows depict flow of goods; dotted blue lines represent the possibility of an international border; green boxes depict examples of particularities to some medical device supply chains. This schematic is not intended to be exhaustive.
Source: Adapted from Chen, P. et al. (2021[26]), Medical Device Supply Chains: An Overview and Description of Challenges During the COVID‑19 Pandemic, https://aspe.hhs.gov/reports/medical-device-supply-chains.
Trade in medical devices has also been on the rise, with increasing diversity of leading exporters and importers
Trade statistics for medical devices (as defined by the WTO in its work on the identification of medical products in trade statistics) suggest trends similar to those of pharmaceutical products, with increasing trade flows and an internationalisation of supply chains (Figure 1.9). Medical devices also include capital goods, i.e. machines and devices that are used repeatedly to provide health services or used to manufacture other medical goods. Trade in medical equipment accounts for the largest share of trade in medical devices by value (34% in 2022) followed by personal protective equipment (30%) and other medical supplies (25%), with the smallest share observed for orthopaedic and other assistive equipment (11%) (Figure 1.10).
Figure 1.9. World trade in medical devices (1995‑2022), by value
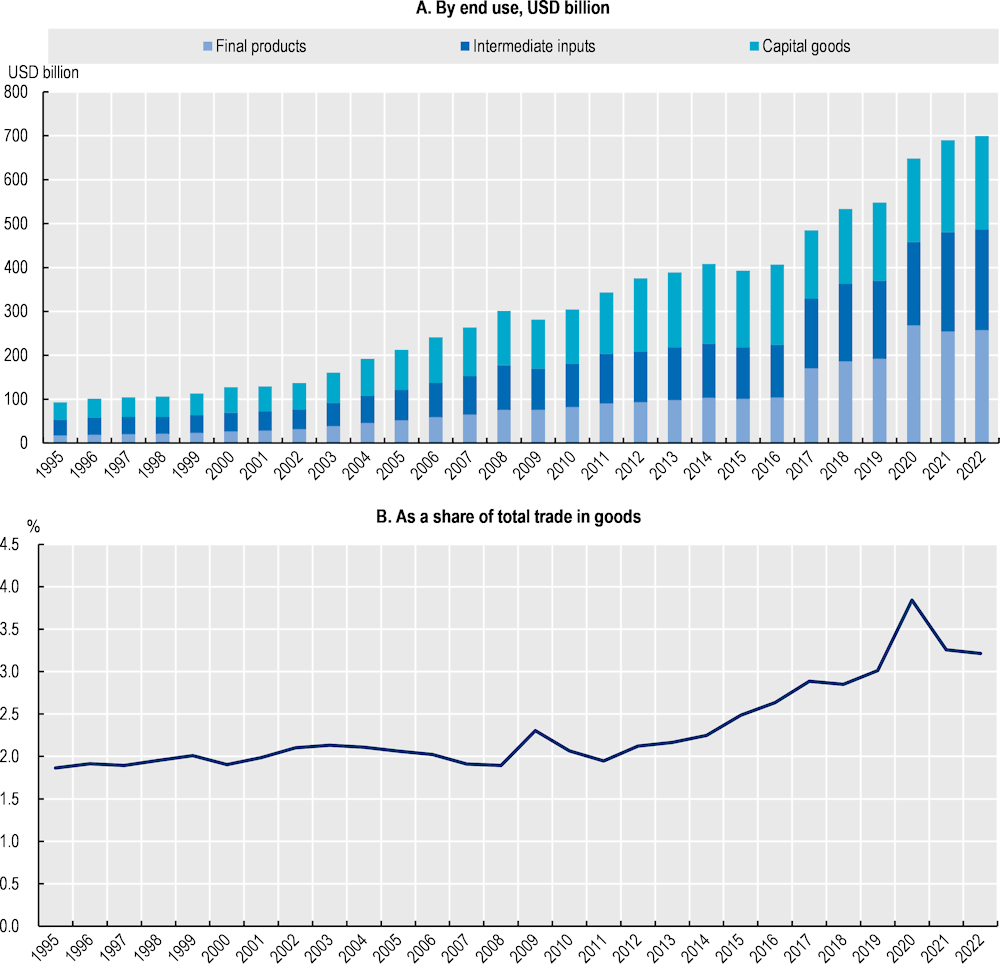
Note: Based on WTO list of medical equipment and machines (majority of products in HS90), orthopaedic devices, personal protective equipment and other medical supplies. Data prior to 2017 are based on older versions of the HS classification and may not accurately reflect the list of products.
Source: BACI (CEPII) (2023[2]), BACI Trade data, www.cepii.fr/CEPII/en/bdd_modele/bdd_modele_item.asp?id=37; and Drevinskas, E., E. Shing and T. Verbeet (2023[3]), Trade in medical goods stabilises after peaking during pandemic, www.wto.org/english/blogs_e/data_blog_e/blog_dta_23may23_e.htm.
Figure 1.10. Composition of world trade in medical devices (2022), by value
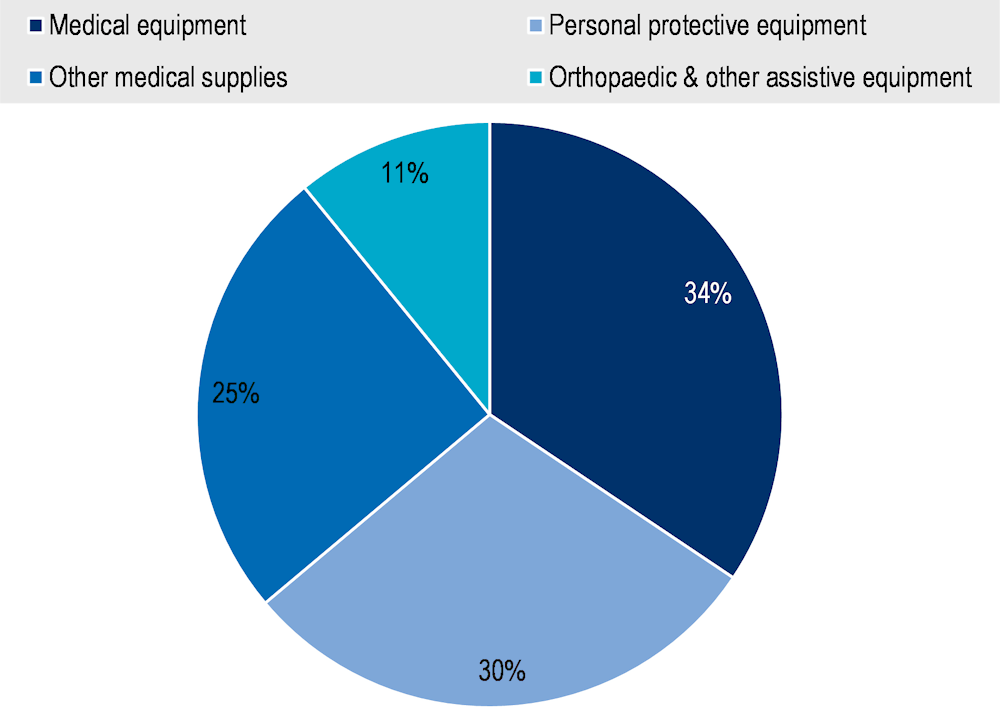
Note: Medical equipment = Medical equipment and machines (majority of products in HS Chapter 90) including magnetic resonance imaging apparatus, X-ray tubes and operating tables; Orthopaedic and other assistive equipment = items such as wheelchairs, spectacles, hearing aids and artificial teeth; Personal protective equipment = Equipment and single‑use items, such as gloves and face masks (excluding protective garments, as HS classifications largely overlap with products for non-medical use); other medical supplies = Hospital and laboratory inputs and consumables, such as syringes.
Source: Drevinskas, E., E. Shing and T. Verbeet (2023[3]), Trade in medical goods stabilises after peaking during pandemic, www.wto.org/english/blogs_e/data_blog_e/blog_dta_23may23_e.htm.
The heterogeneity of medical devices traded and the variability of their supply chains lead to an even more geographically diverse array of leading exporters and importers than for medicines (Figure 1.11). The United States is the leading importer of all types of medical devices by value due to its large market and high level of health spending. With the exception of personal protective equipment, the United States is also the top exporter of medical devices. However, some Asian economies are specialised in the production of medical devices. China is the leading exporter of personal protective equipment and among the top three exporters in other categories of medical devices. Singapore is also among the leading exporters of medical and orthopaedic equipment.
Figure 1.11. Top exporters and importers of medical devices (2022), USD billion
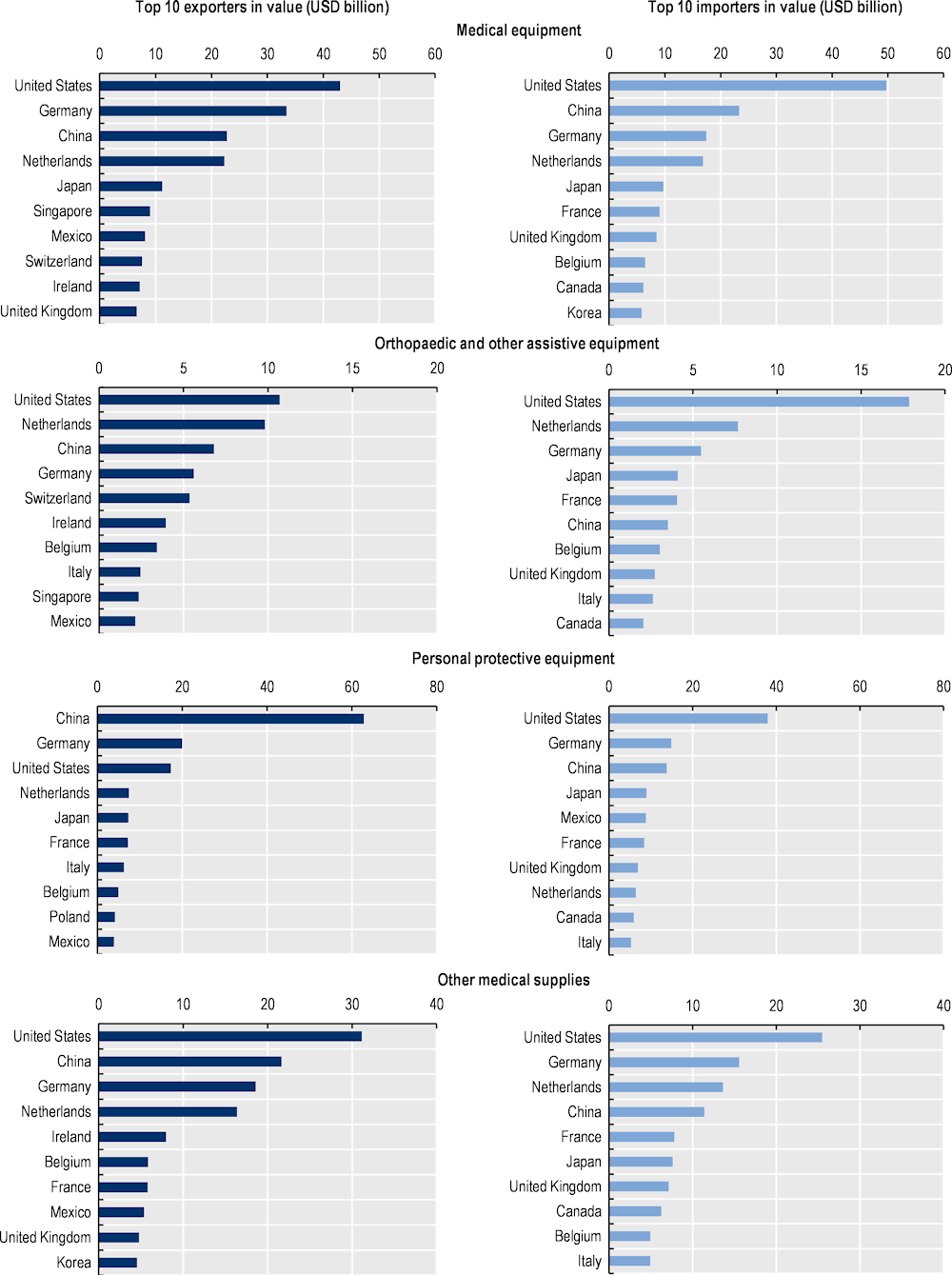
Note: Medical equipment = Medical equipment and machines (majority of products in HS Chapter 90) including magnetic resonance imaging apparatus, X-ray tubes and operating tables; Orthopaedic and other assistive equipment = items such as wheelchairs, spectacles, hearing aids and artificial teeth; Personal protective equipment = Equipment and single‑use items, such as gloves and face masks (excluding protective garments, as HS classifications largely overlap with products for non-medical use); other medical supplies = Hospital and laboratory inputs and consumables, such as syringes.
Source: Drevinskas, E., E. Shing and T. Verbeet (2023[3]), Trade in medical goods stabilises after peaking during pandemic, www.wto.org/english/blogs_e/data_blog_e/blog_dta_23may23_e.htm.
Individual medical device supply chains are unique: Some examples
As already mentioned, the term “medical devices” encompasses a broad range of products and product types, each with a unique supply chain. To illustrate these differences, some examples are described below:
Ventilators are a form of durable equipment, although their use involves various disposable items (Chen et al., 2021[26]). They consist of durable machinery responsible for air pressurisation, valves to manage pressure regulation, and electronics that monitor and control the delivery. Plastic tubes connecting the patient to the ventilator are disposable components. Ventilator supply chains involve medical equipment companies, maintenance repair companies, and repair service contractors. While supply chains of the disposable components are fairly simple, ventilators can consist of more than 1 500 parts, involving many different suppliers. Some individual components may be common to other types of devices manufactured by companies in both the health and non-health sectors, while others may be specific to a particular application or care setting. While consumables, such as disposable ventilator circuits, are often sold through distributors, ventilators are sold directly to hospitals or healthcare organisations or leased through medical equipment companies (Chen et al., 2021[26]).
Facemasks, a form of disposable personal protective equipment (PPE), are generally made from nonwoven fabric made of synthetic fibres (primarily polypropylene, a polymer derived from oil) that are melted (or “melt-blown”) to create a filtration system that can trap small particles (Chen et al., 2021[26]; OECD, 2020[27]). They are an example of a device supply chain that includes both non-medical manufacturers and non-hospital end users. Facemask production is a relatively complex process with different types of inputs and assembly of various parts, requiring specialised machinery. They generally consist of three layers of different materials, in addition to nose strips made from metal, and ties or loops that need to be manufactured separately. Masks then need to be sterilised prior to testing and packaging. While the manufacture of polypropylene non-woven fabric is widespread, as the input is used by non-medical manufacturers (e.g. as crop cover, for air filters, diapers, personal care products etc.), the melt-blown process is concentrated among a limited number of companies. The primary constraint in facemask production has been linked to a shortage of propylene non-woven fabric, the key input. Before the COVID‑19 pandemic, China was the main producer of masks, accounting for around half of global production (OECD, 2020[27]). The sourcing of facemasks has since become diversified with additional suppliers emerging in other countries (OECD, 2022[28]).
Testing supplies and equipment are the components required to conduct clinical laboratory testing for disease diagnosis, screening, and surveillance. Required components depend on the specific type of test, each with its own manufacturing processes. As an example, COVID‑19 tests (including polymerase chain reaction – PCR – and antigen tests) are composed of various components, nearly all of which can be used for other types of tests. The supplies and equipment include nasal swabs, blood collection kits, chemical laboratory reagents, transport media (i.e. packaging that aids transfer to laboratories without contamination), testing machinery, and simple plastic consumables such as micropipettes, among others (Chen et al., 2021[26]; OECD, 2020[27]). Consumable testing components such as the pipettes, swabs, reagents etc., have similar supply chains to that shown in Figure 1.8, and are typically sold through distributors. However, testing machinery, such as PCR machines, have more complex supply chains that involve laboratory equipment leasing companies, third party maintenance companies, and service maintenance contracts (Chen et al., 2021[26]).
To inform this report, the OECD also analysed the supply chain of continuous positive airway pressure (CPAP) devices more closely, described in Box 1.3.
Box 1.3. Continuous positive airway pressure (CPAP) device supply chain at a glance
Continuous Positive Airway Pressure (CPAP) ventilation is an important therapeutic modality in respiratory medicine. CPAP machines provide non-invasive positive pressure ventilation to patients through a tight-fitting nasal mask, face (oro-nasal) mask or helmet to improve oxygenation and reduce the work of breathing (see Figure 1.12 for the device setup). They are generally used to treat obstructive sleep apnoea, although they can also be used as respiratory support in certain medical conditions including COVID‑19. While CPAP devices assist in keeping airways open via a constant flow of air, they differ from the traditional invasive mechanical “ventilators” in critical care that can take over the entire breathing process to provide respiratory support to intubated patients who cannot breathe on their own. Many ventilators can support multiple methods (or modes) of ventilation1, including CPAP; however, this is part of the broader functionality of the ventilator itself, and is not a standalone CPAP device.
Figure 1.12. Block diagram of the CPAP device setup, showing the different components
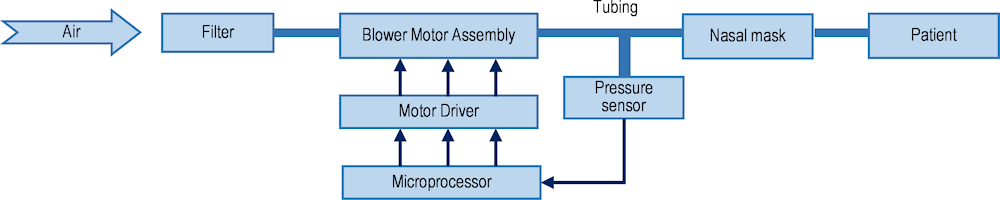
Source: Reproduced from Chen, Z., Z. Hu and H. Dai (2012[29]), Control system design for a Continuous Positive Airway Pressure ventilator, https://doi.org/10.1186/1475-925x-11-5 (CC BY 2.0).
While Bilevel Positive Airway Pressure (BiPAP) offers dual pressure levels catering to inhalation and exhalation, and invasive ventilators cater to more acute patients, CPAP remains a critical component in this spectrum, ideal for conditions such as COVID‑19. CPAP devices were included in the WHO priority list of medical devices in the COVID‑19 response (WHO, 2020[30]). Their fundamental role in enhancing oxygenation, coupled with the potential ability to avert the need for invasive intubation and ventilation, underscores their value, especially when planning for future respiratory health crises.
CPAP devices have long and complex global supply chains. The most important components of a CPAP device are the electronic board and the blower. The electronic board or printed circuit board (PCB) is responsible for controlling the functionality of the device and the communication chips necessary for running the board, from regulating airflow to adjusting pressure settings based on the user’s needs. The PCB’s design and manufacturing require precision engineering, often involving advanced electronic manufacturing services from different parts of the world. The communication chips, integral to the PCB, enable the board’s functionalities. These chips ensure that different parts of the CPAP machine communicate effectively with each other. They also allow for data logging and, in some modern devices, enable remote monitoring of patient usage and device functioning through wireless connectivity. The blower or turbine is another crucial component, responsible for generating a consistent and controlled flow of air. The quality and reliability of the blower are vital for the effectiveness of CPAP therapy, as it needs to maintain a steady air pressure regardless of external factors like voltage fluctuations or varying breathing patterns of the patient. Apart from these main components, there are various other elements often built into a CPAP device, depending on its functionality and purpose. These elements include different kinds of gas supply systems, oxygen blender, separate oxygen and air flowmeters, tubing systems, humidifier, bacterial and viral filter, a mask, as well as various other plastics.
1. Other modes of ventilation (i.e. methods of inspiratory support) supported by ventilators include Pressure Control, Volume Control, Pressure Regulated Volume Control, Pressure Support, and BiPAP (Bilevel Positive Airway Pressure) which is another form of non-invasive ventilation.
Source: Authors as cited and based on consultations with experts in 2023.
1.2. Growing issue of medical product shortages pre‑dated the COVID‑19 crisis
A shortage occurs when demand for an approved medical product exceeds its supply, making it inaccessible to patients in need. Shortages may be local, national, or global; they may last a few days, months or even years. Not every reported shortage will impact patients, but a shortage can become a public health issue if no appropriate alternative exists. Even when alternatives are available, shortages may incur costs to health systems, because of the time spent by health professionals to adapt treatments and source alternatives. Furthermore, shortages of different types of medical products (e.g. diagnostics) may interfere with the appropriate use of others (e.g. certain medicines). Country definitions of shortages vary widely (see Box 1.4 for an example).
Medical product shortages can have multiple causes. They may arise because of a sudden, unanticipated surge in demand, for example during exceptional outbreaks of seasonal infections. Most often, they occur due to disruptions in the supply chain although disruptions do not necessarily result in shortages if appropriately managed. Shortages may also be due to market exit from the manufacturer.
While COVID‑19 highlighted vulnerabilities in supply chains, shortages of medical products had become increasingly common in a number of countries prior to the pandemic. The following text summarises available information on shortages of different types of medical products and highlights some of the related causes. It does not address COVID‑19 specific shortages, which are discussed in Section 1.3 of this chapter.
Box 1.4. Medicine shortage definitions vary from country to country
Formal definitions of “medicine shortages” vary widely, and these have been discussed extensively elsewhere in the literature (e.g. (World Health Assembly, 2017[31]; WHO, 2017[32]; Acosta et al., 2019[33]; Troein et al., 2020[34]). In general, OECD countries consider a medicine shortage to exist when supply is insufficient to meet demand at national level, and may include both temporary and permanent discontinuations (i.e. withdrawal from the market). Some countries also include a minimum duration of supply disruption in their shortage definitions.
For the purposes of this report, a “medicine shortage” is referred to as “any supply disruption or sudden change in the supply-demand equilibrium of a marketed pharmaceutical product that leads to an actual or anticipated lack of stock on the shelf for patients”, as per the definition used by (Chapman, Dedet and Lopert, 2022[1]). These include both temporary and permanent supply discontinuations; the latter sometimes referred to as “availability issues”. This definition does not include situations of “non-availability” or “unavailability”, where a product has not been marketed in a particular jurisdiction.
As medicine shortage definitions vary widely from country to country, so do reporting methods and requirements and, as a consequence, so does the content of national shortage notification databases. For example, some notification databases capture temporary supply disruptions at pharmacy or wholesaler level, while others only include notifications from marketing authorisation holders of shortages resulting from upstream factors for those medicines deemed most critical to the country’s health system. This lack of harmonisation renders cross country comparisons of shortage notification data particularly challenging.
The “non-availability” of a medicine, which is not considered as a shortage in this report, may nevertheless be matter of significant concern for public health. For example, a number of countries report the absence of paediatric formulations for tuberculosis treatment in their domestic markets (WHO, 2023[35]). While products exist globally, they are not approved for sale in these countries and must be imported from others. In Europe, for example, the relatively low prevalence of tuberculosis means that some manufacturers consider the market too small to launch their products. Low prices and high regulatory standards discourage some companies from producing, registering, and supplying their products in these markets, preferring instead to focus on markets with higher disease burden and potentially greater returns (Chorba, 2023[36]; Edwards et al., 2023[37]).
1.2.1. Shortages of pharmaceuticals have gained increasing attention in recent years
Previous OECD work published in 2022 found that, in a sample of 14 OECD countries, the number of shortage notifications increased by 60% over the period 2017 to 2019 (Chapman, Dedet and Lopert, 2022[1]). Differing stakeholder perceptions and a lack of a standardised definition of a shortage, however, make their quantification challenging. Studies have examined medicine shortages at international, hospital, and community pharmacy levels. A 2020 analysis across 11 EU countries revealed that cardiovascular medicines were most severely affected by active shortages between January and August 2019, accounting for 27% of shortages, followed by nervous system medicines at 25% (Troein et al., 2020[34]). Shortages affected a diverse range of products and manufacturers in different countries (ibid.). Hospital pharmacists reported increasing problems with shortages, with antimicrobials consistently the most frequently affected, followed by oncology medicines and anaesthetic agents. Community pharmacies also faced shortages across all medicine classes, with cardiovascular medicines being the most severely affected. Overall, shortages primarily involved older, off-patent medicines, with injectables and generics featuring prominently (Chapman, Dedet and Lopert, 2022[1]).
These findings are generally consistent with a 2022 study analysing the situation in 20 EEA countries between 2008 and 2020 (Jongh et al., 2021[38]). Over the entire period and for the full sample, the medicine classes featuring most prominently in shortage notifications were central nervous system (22% of notified shortages), cardiovascular system (14%), general anti‑infectives (12%), alimentary tract and metabolism (10%) and antineoplastic and immunomodulating agents (7%). These shortages impacted both retail and hospital pharmacies. Nearly half (45%) of all reported shortages affected tablets, and around a quarter (23%) injectables or infusions. However, the latter had a higher probability of being in shortage (+32% vs. +26% for tablets). One‑third of medicines reported in shortage are listed in the World Health Organization Model List of Essential Medicines (WHO EML). Although 97% of medicines in shortage were off-patent and rather old products, statistical analysis shows that patent status and time since launch were not significantly associated with the probability of being in shortage. Around 76% of all shortages involved multisource products for which alternatives existed, while the product in shortage likely represented the only available version for the remaining 24%. The duration of shortages was highly variable from 1 day to 13.5 years, and the average duration across all notifications was 137 days. Two-third of all notifications were resolved within the first three months. Longer durations were reported for shortages arising from commercial reasons.8
The total number of shortages across the 20 EEA countries increased rapidly over the whole period, but this partly reflected an increase in the number of countries reporting shortages. The average number of notified shortages per country grew more modestly. The number of notified shortages in 2019 varied widely, from 13 in Greece to more than 6 500 in Portugal, partly reflecting differences in notification systems and their date of implementation (e.g. Greece had just implemented a notification requirement in 2019) (Jongh et al., 2021[38]). A more recent analysis of shortage notifications in eight EU countries (Belgium,9 Croatia, Finland, Germany, Norway, the Slovak Republic, Slovenia and Sweden) between January 2020 and November 2022 counted 17 250 temporary drug shortage notifications, with the highest numbers observed in Finland, Sweden and Norway. For the same period, 1 737 notifications for permanent drug product withdrawals were counted in Slovenia, the Slovak Republic and Belgium (Ravela, Airaksinen and Lyles, 2023[39]).
Recent statistics for North American countries show varying trends across countries (Figure 1.13). Although the quarterly number of active shortages in the United States had been steadily increasing from 2017 to 2019, it stabilised during the pandemic, decreased slightly in 2021 and began increasing again in 2022 (ASHP, 2023[40]).10 In Canada, the prevalence rate of shortages was increasing until spring 2020; it then declined during the pandemic, before seeing a slight increase in 2022 (Lau et al., 2022[41]). In Colombia, shortages increased steadily prior to May 2021 (Sabogal De La Pava and Tucker, 2022[42]).
Figure 1.13. Recent trends in national shortages of medicines in 3 countries
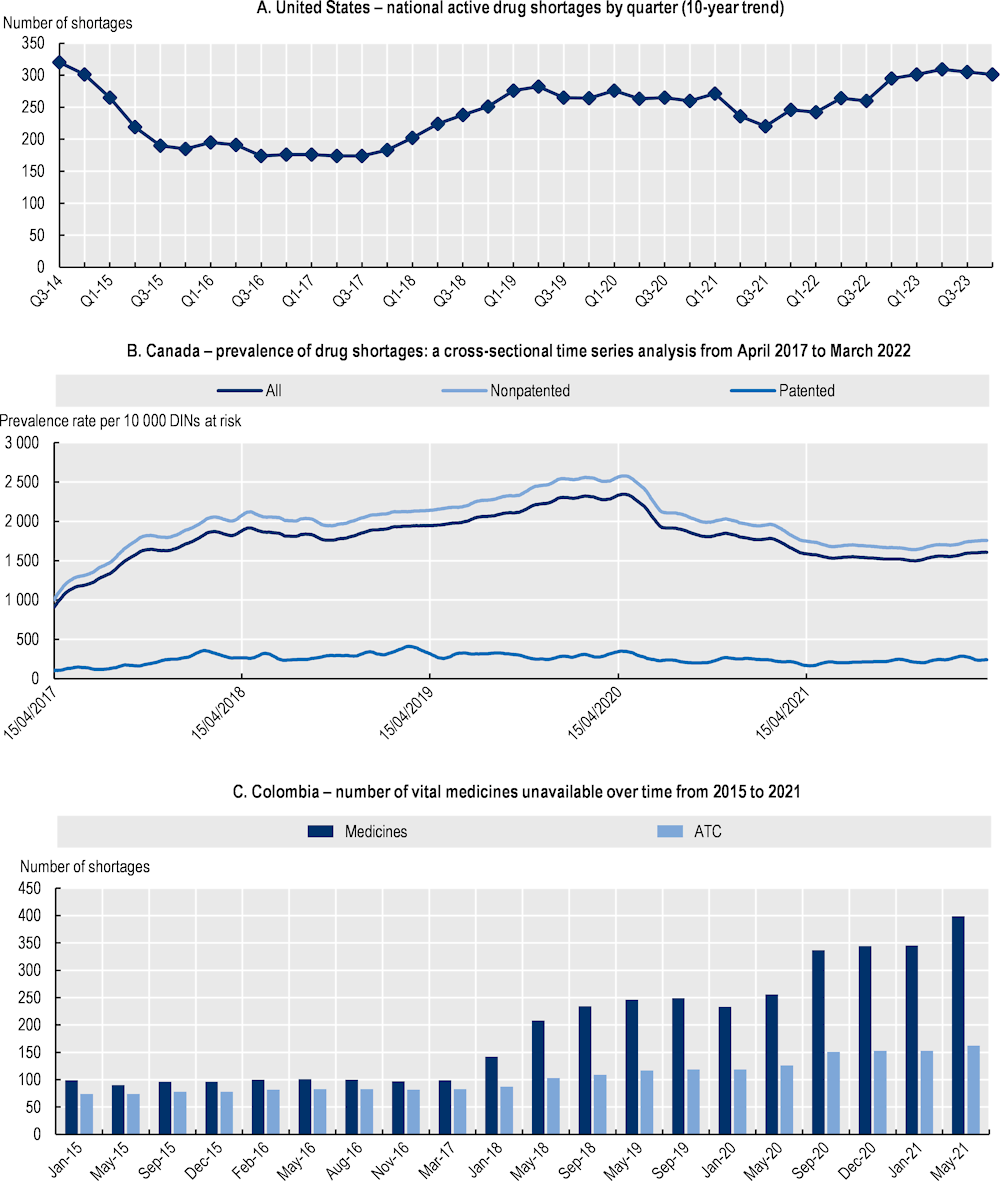
Notes: For the United States: Points indicate active shortages at the conclusion of each quarter. The underlying data are from the American Society of Health-System Pharmacists (ASHP) Drug Shortages website; ASHP commonly lists more shortages than US FDA as it includes shortages that do not meet criteria defined by US FDA (see end note 10). For Canada: DIN Drug Identification Number. For Colombia: ATC Anatomical Therapeutic Chemical classification.
Source: For the United States: Reproduced from ASHP (2023[40]), Drug shortages statistics, www.ashp.org/drug-shortages/shortage-resources/drug-shortages-statistics?loginreturnUrl=SSOCheckOnly; For Canada: Reproduced and adapted from Lau, B. et al. (2022[41]), “COVID‑19 and the prevalence of drug shortages in Canada: a cross-sectional time‑series analysis from April 2017 to April 2022”, https://doi.org/10.1503/cmaj.212070; For Colombia: Reproduced from Sabogal De La Pava, M. and E. Tucker (2022[42]), Drug shortages in low- and middle‑income countries: Colombia as a case study, https://doi.org/10.1186/s40545-022-00439-7 (CC BY 4.0).
Antimicrobials are among the most frequently reported shortages (Chapman, Dedet and Lopert, 2022[1]; Jongh et al., 2021[38]; Beraud, 2021[43]; EMA, 2023[44]). In particular, the 2022 winter season saw a spike in shortages of essential antibiotics in many countries (EMA, 2023[44]). As described in Section 1.1.1, previous OECD analyses highlighted the significant complexity of the manufacturing process for azithromycin (an example of a macrolide antibiotic), with a high concentration of API production in certain countries, albeit with manufacturing sites in different geographic locations (OECD, 2023[8]). Due to pressure on prices and low profitability of generic antibiotic production, there are few competitors at the different stages of the supply chain. A disruption at any stage can result in a shortage. Antimicrobial shortages are a worrying trend, particularly in the face of the wider threat of antimicrobial resistance (OECD, 2023[45]).
Root causes of medicines shortages are multifactorial, but difficult to identify
In many countries, shortage notifications made to regulatory agencies by manufacturers include information on their causes, often selected from a pre‑defined list of potential causes. These lists may differ across jurisdictions.
Shortages of medicines during routine circumstances tend to be attributed primarily to one of two main causes. Around 60% of manufacturer reported shortages are attributed to manufacturing and quality issues (FDA, 2019[46]; Benhabib et al., 2020[47]), such as production defects, input shortages, inventory management problems, temporary or permanent production suspensions due to technical problems or non-compliance with manufacturing standards, and site closures or relocations. The other reason often cited is market dynamics, where poor profitability and a lack of economic incentives make the production of older off-patent products in particular unattractive. Competitive public and private procurement processes often drive prices down to near the marginal cost of production, discouraging suppliers from maintaining surplus stock or investing in capacity and quality improvement. Finally, co‑ordination failures in transportation and delivery systems, including cyberattacks, can disrupt supply chains even when supply and demand are balanced (Chapman, Dedet and Lopert, 2022[1]; FDA, 2019[46]).
In the 2022 study on shortages in 20 EEA countries, available information on causes of nearly 7 000 shortages was reclassified into 7 categories (Jongh et al., 2021[38]). Between 2015 and 2020, 51% of shortages were due to quality and manufacturing issues, 25% to commercial reasons8, 9% to unexpected increases in demand, 8% to distribution issues, 4% to regulatory issues, 1% to unforeseen major events or natural disasters, and 1% to other issues. Over the period, however, the relative proportions of commercial and distribution issues varied inversely, suggesting a degree of overlap. Looking more closely at notifications in Portugal and Ireland, the study identified two major causes: changes in manufacturing site and increased demand in another country. Commercial reasons were further analysed, using stakeholder interviews: tendering practices, penalties for late delivery, and poor profitability were mentioned as influencing shortages in individual countries, but their respective contributions could not be estimated (ibid.).
More generally, empirical analyses of root causes are sparse. A 2021 systematic review identified only three studies (de Vries et al., 2021[48]). Among them, Yurokoglu, Liebman and Ridley (2017[49]) estimated the impact of a 2005 reduction in US Medicare reimbursement rates on shortages of injectable medicines, which had dramatically increased in the 2000s. Looking at a sample of 308 injectable medicines over 12 years, the authors estimated that a 50% cut in reimbursement rates had led to a reduction in manufacturers’ prices and increased the average duration of shortages by about 2 weeks (from an average duration of 59 weeks for the whole sample and the whole period). Since then, Frank, McGuire and Nason (2021[50]) presented empirical evidence on the link between generic prices, market entry/exit and shortages in the US market. Looking at markets for a large -albeit not representative‑ sample of 89 molecules-forms that lost patent protection between 2010 and 2013, the study showed very different patterns for oral and injectable markets, and for small and large markets in the 4 years following patent loss. For oral forms (66 molecule‑form “markets”), larger markets saw robust competition with multiple manufacturers and prices declining, while smaller markets attracted fewer manufacturers and saw prices increase. Shortages were reported in one‑third of these 66 “markets” and were more frequent in large markets (about 50%) than in smaller ones. Product recalls grew sharply in number over the period and affected larger markets (60% of recalls up to 2017) more than smaller ones. For injectable forms, markets are generally smaller, have fewer entrants and exhibit a significant degree of price volatility. Shortages were observed in 16 of 23 of these “molecule‑form markets” over the period, but were more frequent in smaller and medium sized markets (80%) than in larger ones (50%). Recall rates grew after 10 quarters to reach 65% (ibid.).
In a study of the US market, the IQVIA Institute examined market concentration (measured using the Herfindahl-Hirschman Index – HHI11) for medicines in shortage. Multi-source medicines in highly-concentrated markets (HHI 2 501‑9 999) accounted for 68% of shortages; single‑source medicines 27%, and multi-source medicines in moderately concentrated markets (HHI 1 500‑2 500) another 19% (IQVIA, 2023[51]). However, information on market concentration for medicines that are not in shortage is not presented in the report. The report also shows that the proportion of medicines in shortages increases when the price “per extended unit”12 decreases.
Only very limited information is available on (local) shortages due to misallocation in the distribution chain. A single study reports that in Italy, some cases of local shortages due to maldistribution were investigated and found to be the result from illegal behaviour by retail pharmacies (Di Giorgio et al., 2019[52]).
Example: Vaccines
Shortages of several key vaccines have occurred in recent years in OECD countries. However, published reports often group vaccines with antimicrobials, limiting insights about vaccine‑specific shortages. Nevertheless, there are some examples. In Europe, Filia et al. (2022[53]) found a total of 115 vaccine shortages/stock-outs reported in 19 of 21 European countries surveyed between 2016 and 2019, with a median stockout duration of 5 months (ranging from less than 1 month to 39 months). The most commonly affected vaccines were Dipetheria-Tetanus (DT)- and Td-containing combination vaccines,13 hepatitis B, hepatitis A, and Bacillus Calmette‑Guérin (BCG). Around 30% of shortage/stockout events for which information was available led to temporary changes in countries’ national immunisation programmes (e.g. alternative schedules, changes to the timing of doses or boosters, prioritisation of vulnerable groups) (ibid.). In the community pharmacy setting in Europe, 55% of responding countries reported vaccines to be in short supply in 2022, up from 44% in 2021, but well below the 88% reported in 2020 (PGEU, 2022[54]). The number of vaccine shortages reported by hospital pharmacists in the EU has been declining, with only 15% of survey participants reporting them as an issue in 2023 compared to 43% in 2018 (Miljković et al., 2019[55]; EAHP, 2023[56]). In the United States, a 2017 study found that there were 59 reported shortages of vaccine and immune globulin products in the period between 2001 and 2015, with half of these shortages involving paediatric vaccines (Ziesenitz et al., 2017[57]). They also found that the median number of new shortages reported annually to be 3, and a median shortage duration of 16.8 months (ibid.). In Australia, several “high” and “critical” impact shortages of different vaccines of variable duration were reported by manufacturers between 2014 and 2023, based on national medicine shortage data.14
The fluctuations in national vaccine shortages over time may reflect so called “global shortages or disruptions” of specific vaccines. For example, 2015 saw a shortage of pertussis-containing combination vaccines as a result of reduction in pertussis antigen production capacity (ECDC, 2016[58]). Fourteen EU Member States reported shortages of DT- containing vaccines in the period 2016‑19; which were mainly attributed to interruptions in production and supply (Jongh et al., 2021[38]; Filia et al., 2022[53]). Another example saw reported shortages of hepatitis A vaccine in several European countries (e.g. Austria, Denmark, Italy, Portugal, Spain and Sweden) as well as in the United States, linked to a spike in demand arising from an outbreak of hepatitis A, compounded by existing production issues (ECDC, 2017[59]; WHO, 2017[60]). Similarly, the BCG vaccine has been in shortage across multiple countries since 2012, also due to manufacturing quality problems and high demand (Filia et al., 2022[53]).
Causes of vaccine shortages are likely multifactorial. It is important to consider, however, that vaccines are different to other medicines, with lengthy production processes involving highly specialised facilities and equipment, and with additional quality controls and testing to ensure the safety and quality of these products that are administered to otherwise “healthy” populations. According to Filia et al. (2022[53]), the two most commonly reported causes of stockouts/shortages in 19 European countries surveyed between 2016 and 2019 were interruptions in production and/or supply due to quality issues or other reasons (n = 39; 33.9%) and global shortages (n = 35; 30.4%),15with higher-than-expected demand due to changes to vaccine schedules or targeted groups (7.0%), inaccurate forecasts (4.3%) or an outbreak/other reasons (4.3%) (Filia et al., 2022[53]). Other factors (13.9%) included delayed delivery, lack of suppliers, and issues at procurement level (e.g. delays, legislation, absence of reimbursement) (ibid.) In the United States, manufacturing problems were cited as the primary cause of vaccine shortages between 2001 and 2015 (50% of cases), followed by issues with supply and demand (7%) (Ziesenitz et al., 2017[57]). Reported reasons for shortages of vaccines in Australia between 2014 and 2023 included manufacturing issues (measles/mumps/rubella vaccine), seasonal stock depletion (influenza), and unexpected increases in consumer demand (e.g. rabies, hepatitis A, hepatitis B, and cholera vaccines), among others (TGA, 2023[61]).14
The challenges in vaccine supply chains stem from a range of issues linked to complexities of the manufacturing and quality control processes, regulatory factors, and uncertain demand. From the industry perspective, Vaccines Europe,16 an organisation representing 14 vaccine companies operating in Europe, identified several root causes of vaccine shortages in that region through consultations with experts from the four member companies with the largest portfolio of EU-marketed vaccines (GlaxoSmithKline, Merck Sharpe & Dohme, Pfizer, Sanofi Pasteur) (Pasté et al., 2022[62]). Industry experts highlighted the complexity of vaccine manufacturing, which involves intricate processes with necessary stringent quality controls, leading to lengthy production timelines that require contracts (with suppliers and health authorities) to be arranged well in advance. Complicating matters are unpredictable timelines due to independent lot releases by national control laboratories. Regulatory factors add further complexity, necessitating frequent post-approval changes to be submitted by manufacturers (e.g. due to improvements in facilities, equipment or processes; quality control; changes in suppliers etc), sometimes requiring submissions to over 100 regulatory agencies globally for a single change (ibid.). Nevertheless, these stringent requirements are necessary to ensure safety and effectiveness of vaccines, as well as compliance with good manufacturing practices.
Industry experts further highlighted that the diversity in vaccine presentations, and packaging and labelling requirements across countries results in the need to manufacture and distribute vaccines in smaller volumes, posing challenges for efficiency in production and inability to redistribute in the event of supply disruptions. Unpredictability in global demand, challenges in anticipating changes in vaccine recommendations, and difficulty in gaining accurate demand forecasts from health authorities were also cited by industry experts (e.g. due to development of national immunisation programmes, changes to existing guidelines, disease outbreaks etc). In addition, suboptimal vaccine budgets and procurement practices that do not take into account long lead times were mentioned (Pasté et al., 2022[62]). Other analyses cite similar vulnerabilities in vaccine supply chains, while also highlighting the added challenge of concentrated production with few global suppliers (Jongh et al., 2021[38]; WHO, 2023[21]).
Example: Radiopharmaceuticals
Since 2009, the High-level Group on the Security of Supply of Medical Radioisotopes (HLG-MR), established by the Nuclear Energy Agency, has been working on addressing shortages in the supply of certain radio‑isotopes. In the case of Technetium‑99m, used in 85% of nuclear medicine diagnostic scans performed worldwide – around 30 million patient examinations every year –, ageing production facilities and low prices have contributed to inadequate production capacity, making the supply of Tc‑99m unreliable. The current structure of the supply chain leaves some participants unable to increase the prices of their services to the levels needed to cover all fixed and variable costs of the required production capacity (OECD/NEA, 2019[63]).
Example: Plasma-derived medicinal products
In recent years, PDMP shortages have affected many regions across the world, particularly shortages of intravenous immunoglobulins (IVIg) for which there is no alternative broad-spectrum antibody. Following the onset of the COVID‑19 pandemic, blood product donations decreased due to social restrictions and health concerns and are only rebounding slowly (Covington, Voma and Stowell, 2022[64]). For example, one study reported that several OECD countries (the United Kingdom, France, Greece, Latvia, Lithuania and Portugal) have experienced shortages of intravenous and subcutaneous IVIg as a result of insufficient supply and market withdrawals over recent years (Strengers, 2023[10]). Even countries with significant sources of commercial plasma such as Germany, Czechia, Hungary and the United States have experienced shortages of IVIg. Another study focusing on the possible impact of COVID‑19 on plasma supply in the United States, reported a sharp drop in donations that is only rebounding slowly to pre‑pandemic levels (Covington, Voma and Stowell, 2022[64]).
The global supply of plasma is dominated by the collection of source plasma in the United States, which not only caters for the domestic market but is also exported (Strengers, 2023[10]). Pre‑pandemic data show that 67% of source plasma originated from the United States, whereas Asia-Pacific accounted for only 18%, and Europe 14% (Strengers, 2023[10]). In Europe, the majority of source plasma originates from four countries only. These are Austria, Czechia, Germany, Hungary. While on average, 14 litres are collected annually per 1 000 inhabitants in Europe, the United States collects roughly 113 litres per 1 000 inhabitants (Kluszczynski, Rohr and Ernst, 2020[16]).
Reasons for shortages are likely to be two‑fold: increasing numbers of patients eligible to be treated with plasma-derived therapies, and uncertainty in the supply of the raw material (i.e. plasma from human donors). The latter depends on eligibility requirements, the allowed frequency of donation, and different donation compensation policies in each jurisdiction. The COVID‑19 pandemic also impacted the volume of blood and plasma collected. Since it can take up to a year for plasma to be processed, the effect of a downturn in donations may remain unnoticed for a long period of time and will not be experienced as acutely as that of whole blood or red cells. In addition, disruptions in source plasma may not be readily perceived by transfusion services, which primarily focus on the collection of red cells and platelets (Covington, Voma and Stowell, 2022[64]).
PDMP manufacturing is challenging as it is affected by variations in the volume of donations, complex regulations, strict safety procedures to ensure purity and eliminate potential viruses and bacterial contamination, as well as lengthy manufacturing processes that can take 7‑12 months (Hess, 2010[65]). The most difficult challenge is in the collection of raw material, i.e. plasma, which can only be sourced from human donors. Finding potential donors is the first and most relevant hurdle to mitigate supply shortages. Beyond eligibility requirements, varying donation frequency and compensation schemes, donations are highly vulnerable to the effects of bad weather, health crises, geopolitical tensions, that can discourage even willing donors.
Demand for PDMPs is expected to increase at an annual rate of 6‑7% (PPTA, 2022[15]). Recent research suggests that consumption in Europe alone is projected to increase by one‑third, from 50.5 tonnes in 2017 to 67.5 tonnes in 2025 (Marketing Research Bureau, 2023[17]). The availability of plasma has become even more relevant than in recent years as research and diagnostics have evolved (Marketing Research Bureau, 2023[17]). According to data from the International Patient Organisation for Primary Immunodeficiencies (IPOPI), there are many patients who have not yet been diagnosed with diseases that require PDMP treatment (Strengers, 2023[10]).
1.2.2. Vulnerabilities in medical device supply chains have received less attention
Before the COVID‑19 pandemic, vulnerabilities in medical device supply chains received less attention than medicine shortages. This may be due in part to differences in notification requirements of potential shortages. In the United States, for example, notifications of potential shortages are required of medicine manufacturers at all times, but of medical device manufacturers only when there is a public health emergency.17 The FDA only reported five shortages of medical devices annually between 2010 and 2019, but this increased fourfold in the first half of 2020. Pre‑pandemic, 60% of shortages were reported to stem from regulatory and enforcement actions related to product quality and manufacturing. Other triggering events were natural disasters, discontinuations and economic factors (Beleche et al., 2022[66]).
In Europe, the 2023 version of the European Association of Hospital Pharmacists’ Shortage Survey (2023[56]) included data on medical devices for the first time. Although these data cannot be considered representative, they provide some insights into shortages of medical devices in hospitals. According to 61% (n=765) of hospital pharmacists, medical device shortages caused issues for patients, with more than a third reporting that the shortages occurred one to three times for the same device, with another third reporting that they experienced the issue more than 10 times for the same device. Pharmacists most commonly reported supply chain problems as the reason for shortages (53%, n=658), followed by a shortage or discontinuation of a component, part or accessory of the device (48%, n=603).
Shortages affecting in-vitro diagnostics (IVD) also occurred episodically (see Box 1.5).
Box 1.5. Shortages affecting in-vitro diagnostics
In 2021, the United Kingdom experienced a shortage of blood specimen collection tubes as a key supplier announced global supply chain issues (Rimmer, 2021[67]). According to the company, shortages were caused by the COVID‑19 pandemic. Manufacturers struggled to meet the high, changing, and unpredictable demand; this was aggravated by delivery delays due to global transportation issues, limited availability of, and access to raw materials, and delays due to UK border checks. In addition to this, the equipment used by the UK National Health Service (NHS) to run various diagnostic tests was set up to use tubes from a particular manufacturer. Using alternative collection tubes was possible but required prior validation of the substitutability to ensure test validity (Tsang, Absar and Gingrich, 2021[68]; Gosselin et al., 2021[69]).
A few months later, the US FDA (CDC, 2022[70]) and the Canadian Government (Government of Canada, 2022[71]) updated their respective device shortage lists to include all blood specimen collection tubes. Early in 2022, in the United States, this had a major impact on the availability of testing for sexually transmissible diseases, specifically syphilis and HIV (Raiken, 2022[72]). The company only managed to recover 97% of former supply levels by April 2023 (BD, 2023[73]).
In response to the shortages, UK NHS authorities published guidelines restricting the use of blood tests to avoid disruptions to urgent care. A clinical reference group, with advice from professional bodies covering a wide spectrum of clinical specialties, recommended actions for medical directors, nursing directors, general practitioners (GPs), pathology laboratories and all clinical staff including stock check, ordering and double tube practices; minimum re‑test intervals; and usage of Point of Care haemoglobin devices (NHS, 2021[74]; The Royal College of Pathologists, 2021[75]). Physicians were told to suspend non-essential blood tests (e.g. genomic tests for cancer diagnoses). Acute and mental health trusts were required to reduce their demand by a minimum of 25% during the shortage period. Guidelines included examples of clinically urgent testing, such as a test that could potentially avert a hospital admission or onward referral; or tests for patients with suspected sepsis or conditions with a risk of death or disability (NHS, 2021[76]). The guidance also warned against stockpiling of test tubes and urged GP practices to order supplies from NHS Supply Chain rather than trying to buy the equipment directly from manufacturers (Osborne, 2021[77]). Alternative products were being sought in co‑ordination with the Pathology Incident Director and NHS Supply Chain, and all laboratories that were switching to these supplies received samples for testing and validation (ibid.).
Similarly, the FDA recommended healthcare providers, laboratory directors, phlebotomists, and other personnel to consider conservation strategies to minimise blood collection tube use and maintain quality and safe patient care for those for whom testing was medically necessary (CDC, 2022[70]).
In England and Wales, a private supplier of IVD tests – offered an alternative supply for patients outside the NHS system (i.e. without public funding), for both home tests and tests performed in clinics. For home test samples, the company used microtainer test tubes, which utilise smaller blood samples obtained via finger prick (Better2Know, 2021[78]).
Causes of medical device shortages or risk of shortages
In published reports and interviews, stakeholders cite the following issues as likely to affect medical device supply in the future:
Competition with other industrial sectors for the acquisition of raw material and critical components has been mentioned both in case studies and stakeholder interviews. This is of particular concern where there are supply disruptions of key source materials that are not (easily) substitutable and are required for the production of critical or lifesaving devices.
New regulations on market access applicable in the European Union (see Box 1.6). Changes in the regulatory environment in the EU and the application of more stringent criteria and processes are expected to result in bottlenecks in the evaluation process, and in the market exit of small companies marketing older products, as well as a reduction in the range of devices manufactured by others. Several deadline extensions have provided more time for manufacturers and assessment bodies to prepare for the transition but the long term impacts of the regulatory reforms on the number of products and suppliers are difficult to predict.
Proposed changes in the regulation of chemical substances in manufacturing (Regulation on the registration, evaluation, authorisation, and restriction of chemicals – REACH). At the EU level, a proposal to restrict the use of around 10 000 per- and polyfluoroalkyl substances (PFASs) was submitted in January 2023. This proposal is intended to reduce the use of these chemical substances, which are very persistent in the environment and have negative effects on human health, and whose use is increasingly prevalent in manufactured goods (ECHA, 2023[79]). Some of these substances are used in medicines and medical devices. The European Chemical Agency, supported by specific scientific committees, produced a report addressing the risks of PFASs to the environment and human health and provided an assessment of the effectiveness, practicability, monitorability and socio‑economic impacts of restriction of the use of PFAS under the REACH Regulation (ECHA, 2022[80]). According to this report, the medical device sector is one of the most relevant sectors in terms of emissions of PFAS in the use phase (i.e. excluding waste). The report analysed several sub-categories of medical devices, such as implantable devices, tubes and catheters, and diagnostic laboratory testing, to determine whether alternative devices were available, as well as the consequences of restricting PFAS use (ibid., pp. 99‑102). For the three categories mentioned above, the potential for device substitution is low, and the unavailability of these devices would have a negative impact on human health. The reform proposal aims to exclude these devices from the restrictions on PFAS use. By contrast, use of PFAS in medical device packaging would be prohibited unless “vital for the functionality and safety” of the medical devices when no alternative method is available. The proposal envisages a derogation of the application of PFAS use restriction for a period of several years (to be determined), to account for the time to invest in R&D to find other solutions (ibid. pp. 127‑131).
Vertical integration in the laboratory sector, limiting the substitutability of components/reagents for IVDs. For example, during the pandemic, a specific type of blood specimen collection tubes went into shortage. Because some machines were calibrated specifically for the use of these tubes, there was no possibility of rapidly substituting other collection tubes without compromising the validity of tests (Rimmer, 2021[67]).
Experts and manufacturers mentioned a very high inflation in energy and transportation costs recently, as well as increased costs of raw materials, as an additional risk to supply, especially where price regulation prevents companies from passing on increased costs to consumers (interviews with stakeholders in 2023; (Snitem, 2022[81]).
Natural disasters were also mentioned as events potentially triggering supply shortages (Beleche et al., 2022[66]).
Approaches to averting and managing risks of medical device shortages are different to those of medicines, reflecting the number and extreme heterogeneity of medical devices, as well as differences in regulatory frameworks (including notification requirements). However, as for medicines, a key difficulty is in identifying when a lack of supply of a certain medical device creates a risk to health. Many devices have close substitutes; for example, medical devices approved by the US Food and Drug Administration (FDA) via the 510(K) process18 – claiming substantial equivalence to a legally marketed device – account for about 90% of FDA approvals (Medical Device Network, 2022[82]). In 2022, in France, 80% of new medical devices assessed by the health technology assessment body had no added value over existing comparators for the same therapeutic indication, which indicates that alternative therapeutics exist – even if they are not strictly equivalent (Haute Autorité de Santé, 2022[83]).
Box 1.6. The new EU regulation for medical devices
In 2021, the medical device market in Europe was valued at EUR 150 billion, and represented 27.3% of the global market. The overwhelming majority (95%) of the 34 000 companies acting in this sector in Europe are small and medium-size enterprises (SMEs) (MedTech Europe, 2016[84]). However, a small number of large players account for significant market share.
The EU is currently transitioning from its previous legislative framework for the regulation of medical devices and in-vitro diagnostics. Since 1993, this framework set regulatory requirements for authorisation of products in the EU market, as well as rules governing their quality, categorisation, and post-market surveillance, among other topics. Approved in 2017, the Medical Device Regulation (MDR, EU reg. no 2017/745) and In Vitro Diagnostic Medical Devices Regulation (IVDR, EU reg. no 2017/746) aim to strengthen the regulation, and address quality and safety concerns. A transition period, which benefited from several extensions, is planned to last until May 2028, when all current and new medical devices will have to be approved under the new regulations.
The EU is undergoing consequential changes in its framework for regulating medical devices and in-vitro diagnostics
In general, MDR and IVDR will require more stringent assessments to allow for product commercialisation and utilisation in the EU. New rules can be summarised as follows:
A wider range of products is defined as medical devices, and a larger proportion is classified as “high risk”. Some goods previously considered merely consumer products, such as aesthetic devices and software, are now recognised as medical devices, thus requiring more rigorous registration processes and quality oversight. In parallel, a much larger number of devices and IVDs are now categorised as high-risk, which entails more lengthy and demanding registration processes and post-market surveillance. For example, 80% of IVDs will have to undergo assessment by a Notified Body (NB), the vast majority of them for the first time (European Commission, 2021[85]).
The designation of national third-party assessment bodies for medical devices (i.e. Notified Bodies – NB) now involves a more rigorous certification process. Under EU legislation, the assessment of medical devices and IVDs for registration in the EU is conducted by notified bodies. These third-party private entities must be re‑certified under the new regulation and required to undergo several evaluation processes that involves national regulatory agencies and the European Medicines Agency (EMA).
Stronger requirements for post-market surveillance. All manufacturers will be required to present a quality management system (QMS), which describes a detailed plan to comply with MDR quality and safety requirements in accordance with the risk classification. Apart from class I devices (i.e. the lowest risk class), all medical devices are also required to present periodic safety update reports (PSURs).
The introduction of Unique Device Identifiers (UDI). Each new medical device will be required to have a UDI tag that allows manufacturers, users, and regulators to identify each device individually. The UDI will contain technical and regulatory information about the device and will allow regulators to track products for safety and quality monitoring. All this information will be made publicly available through a centrally managed European database on medical devices (Eudamed), currently being implemented progressively.
Given the more stringent assessment and quality assurance measures, manufacturers and other healthcare professionals have raised concerns about the operational difficulties and costs of adaptation to the new rules. Shatrov and Blankart (2022[86]) predict that “generic” versions of medical devices will be withdrawn from the market and that compliance with the MDR will be challenging for SMEs. The paediatric medical devices sector has been particularly vocal about the increased costs of bringing and maintaining products in the market and the associated risks for supply chains (European Academy of Paediatrics, 2023[87]).
Bottlenecks in Notified Bodies’ designations and increased workloads may stress the EU medical devices registration system
The transition process to the new requirements by NBs has faced some challenges and caused delays in the registration of devices. Of the 56 NBs that functioned under the previous directives, 39 have now been successfully re‑certified (European Commission, 2023[88]). However, the first certification of a NB under MDR took over two years to be completed.
The current pace of submissions and certifications delivered by notified bodies is still very low relative to demands for re‑certification of all existing medical devices, and submissions for new devices. By June 2023, 13 177 applications had been filed, but only 3 899 certifications had been issued. New registrations are also taking considerable time, with 71% of certificates taking longer than 13 months to be delivered by NBs (European Commission, 2023[89]).
In addition to increased workloads, more stringent and demanding assessment for medical device registration may also increase the time and resources NBs need to complete their assessments.
1.3. The case of health crises: Severe health crises, epidemics, and pandemics
An important finding from the risk management literature is that risk management approaches used for disruptions observed in “normal times” differ from those needed in extreme “black swan” events such as the COVID‑19 pandemic (Sodhi and Tang, 2021[90]). Using the example of health crises, Sodhi and Tang (2021[91]) distinguish significant outbreaks of seasonal influenza, occurring every two or three years, where demand for medical products and equipment may double, from epidemics and minor pandemics, that occur every 10 years, where demand increases three or four times, and severe (global) pandemics where demand can increase more than 10‑fold (as with COVID‑19).
Using examples of recent crises (e.g. H1N1, COVID‑19, international conflict), the following text summarises available information on shortages of different types of medical products and outlines some of the associated causes.
1.3.1. H1N1 (swine flu) pandemic
The H1N1 (swine flu) pandemic from 2009 and 2010 was a major health crisis that exposed vulnerabilities in the supply of medical products essential to containing the virus. In contrast with COVID‑19, the 2009 pandemic presented different challenges in managing resources to respond to the crisis. Manufacturing capacity was considerably less affected by public health measures intended to contain the spread of the H1N1 virus with, for example, lockdowns only rarely imposed in very specific cases. Because of the nature of the H1N1 virus and its similarities with seasonal influenza, an effective vaccine was made available five months after the WHO declared the health crisis a pandemic.
Nevertheless, a strong and rapid increase in demand for medical countermeasures presented challenges for manufacturers, while a lack of preparedness measures resulted in shortages of some essential medical products. Although masks were not required for the general population, studies found a substantial uptake in utilisation of facial protective equipment. A study by Murray et al. (2010[92]) found that mask and N95 respirator utilisation in three healthcare facilities in the Vancouver area (Canada) were double that of previous flu seasons. The United States Centers for Disease Control and Prevention (CDC) guidance from July 2010 recognised that multiple healthcare facilities had reported shortages of PPE, leading the agency to issue recommendations on how to reduce health workforce exposure in order to reduce utilisation of such products (CDC, 2010[93])
Concerns around the availability of essential pharmaceuticals – such as antivirals – and influenza vaccines led some OECD countries to stockpile and purchase large quantities of some products in advance. Despite anticipated planning for influenza pandemic scenarios, health authorities in the United States did not anticipate the possibility of a shortage of vaccines. Reduced capacity in vaccine manufacturing led to considerably slower immunisation of target groups during the first two months of the vaccination campaign, followed by declining public willingness to be vaccinated due to waning incidence of H1N1 and reduced media attention. This resulted in a surplus of unused vaccines. Only 90 million of the 162 million doses produced for the general public were finally used (Institute of Medicine (US) Forum on Medical and Public Health Preparedness for Catastrophic Events, 2010[94]).
On the other hand, advance purchases in high-income countries restricted access to vaccines for developing countries (Fidler, 2010[95]). Similarly, concerns about the availability of antiviral supplies – in particular, oseltamivir (Tamiflu®) – had led multiple countries to stockpile large quantities of the medicine. By 2007, France, Austria and Ireland possessed stockholdings of the antiviral sufficient for more than 40% of their populations, and 95 other governments had also guaranteed their own supplies (Elbe, Roemer-Mahler and Long, 2014[96]). EMA had prepared to extend the shelf life cycle of Tamiflu® capsules by two additional years if needed, but severe shortage situations were not reported in the EU (EMA, 2009[97]). In the United States, stockpiling efforts by state and national authorities helped avoid a national shortage of adult antivirals. However, the unexpectedly higher incidence of influenza infection in children resulted in reports of shortages of paediatric Tamiflu® capsules during the autumn of 2009 (US Government Accountability Office, 2011[98]).
1.3.2. COVID‑19 pandemic
The COVID‑19 pandemic is one of the most significant health crises in the last century, causing millions of deaths worldwide, and widespread disruptions to societies and economies. In the initial stages of the COVID‑19 pandemic, severe disruptions in supply and shortages of key medicines, testing reagents, and PPE occurred due both to spikes in demand and bottlenecks in supply. While these may be viewed as examples of supply chain failures, medical supply chains actually showed considerable resilience in the face of extreme stress.
Medical devices saw the most critical spike in demand during the first stages of the response to COVID‑19
According to the 2022 OECD country survey on the Resilience of Health Systems, seven in every ten responding countries reported facing problems with the supply of essential medical devices prior to January 2022 (OECD, 2023[8]). Problems in supply of PPE was the most frequently reported issue during the pandemic, resolved at the time of the survey for 88% of respondents but still an issue for one country. Testing materials and ventilators were the second and third types of items with reported shortage issues (83% and 68% of respondents, respectively). In general, countries that reported issues for one device throughout the pandemic also reported it for other categories of products, while some countries did not report any shortage (OECD, 2023[8]).
International trade figures for face masks illustrate the steep surge in demand (see also Section 1.1.2 for a description of the facemask supply chain). Imports of face masks to the United States from March to May 2020 increased 15‑fold in value, from USD 240 million to USD 3.7 billion (OECD, 2022[28]). Similar steep increases in imports of masks were recorded in other major OECD economies, such as Japan, the European Union and Canada. The first surge in demand from March 2020 was met mainly with supplies originating from China, which accounted for 94% of imports to the United States in July 2020. While aggregate demand for masks remained high throughout the pandemic, disaggregated trade data by different mask types (e.g. N95, FFP2) revealed variable supply chains according to the type of mask. By August 2021, the share of disposable face masks imported from China to the United States had dropped to around 60%, with increased supplies from Mexico, Korea and Viet Nam (OECD, 2022[28]).
The concentration of production of essential medical devices in only a handful of countries and locations led to considerable shocks when global demand rapidly increased. A lack of a co‑ordinated response among manufacturers and suppliers upstream from different countries can potentially increase the number and duration of shortages. In late January 2020, several weeks before the wider, international disruption of global value chains, China had already begun imposing restrictions to contain the spread of Sars-CoV‑2. The measures included the closure of several manufacturing plants in the country, resulting in a shortage of inputs for manufacturing plants and finished products in other economies. Surgical masks and disposable respirators are two examples of products that faced an intense shortage after a surge in demand and the closure of factories in China. In the subsequent months, as Chinese manufacturers re‑opened, the disruptions in manufacturing impacted Europe and North America, where COVID‑19 became a major health risk, resulting in a continuous bottleneck in production (Baldwin and Freeman, 2020[99]; OECD, 2020[100]). In March 2020, WHO was also warning countries about severe disruptions in the global supply of PPE caused by a surge in demand and misuse of available stocks. Its estimates at the time indicated that manufacturers would have to increase production by 40% to meet demand (WHO, 2020[101]).
The COVID‑19 pandemic also generated a different set of demands from healthcare services, which resulted in a need for medical device manufacturers to adapt rapidly. With most hospital beds reserved for COVID‑19 patients, and the cancellation of non-urgent and elective procedures, demand for certain products fell sharply, while essential products like PPE and ventilators were in very high demand (see Box 1.7 for the example of CPAP, a device normally used in sleep apnoea that was used to assist breathing in COVID‑19 patients). At the same time, a widespread and sharp increase in costs for different inputs and logistics services during the pandemic strongly impacted some manufacturers’ capacity to supply the market and sustain their operations. In France, manufacturers surveyed by their trade associations estimated that the prices of commodities such as plastic materials and rare minerals saw a 40 to 90% and a 40 to 370% increase, respectively, from 2020 to 2021 (Snitem, 2022[81]). According to the same source, electronic components such as semiconductors, which are used in a variety of medical devices, also went into shortage globally, resulting in a 4‑fold increase in costs. About 50% of respondents reported a suspension in production for 2 weeks to a month after the outbreak, and 90% reported increases in costs. Delivery times were also deeply affected, in some cases taking three times longer than pre‑pandemic timeframes (Snitem, 2022[81]).
COVID‑19 tests – including PCR and antigen tests – were developed and approved for use within the early months of the pandemic, with demand steadily increasing over time. COVID‑19 diagnostic testing was one of the critical components in containing and mitigating the impact of the virus. The tests and associated equipment consist of various components with different manufacturing processes (see Section 1.1.2). Despite a ramping up of production of the components and a substantial increase in trade flows (Amirian, 2022[102]), the supply of tests did not match the demand (Behnam et al., 2020[103]), sometimes the result of supply chain issues (Griffin, 2020[104]). The testing supply chain experienced issues in ascertainment, production, and distribution of almost all component parts. Early 2021 data indicated that clinical laboratories in the United States were operating at 40% of their normal capacity, with key supply shortages of test kits and consumables as well supplies for non-COVID‑19 tests (Congressional Research Service, 2021[105]). Demand for rapid antigen tests was high in early 2021, with shortages seen in the United States (CDC, 2021[106]) and increasing difficulty in obtaining the tests in Europe (Ding, 2022[107]). The reasons for shortages of already approved tests throughout pandemic included lack of raw materials and inputs including chemical reagents, RNA extraction kits, foam swabs etc, issues with logistics and distribution, as well as incorrect demand assessments.
Box 1.7. Challenges to CPAP supply chains during COVID‑19
The production of Continuous Positive Airway Pressure (CPAP) devices is complex, relying on multiple global supply chains and a variety of regulatory frameworks (see Box 1.3 above). These features, together with an increased demand, led to bottlenecks in the supply of CPAP devices during the COVID‑19 pandemic. In the United States, shortages of CPAP also occurred due to a recall due to potential health risks (FDA, 2021[108]).
At the crux of the CPAP device production challenge during COVID‑19 was the heavy reliance on component manufacturers based in affected countries in East Asia. Most interior components of these devices are sourced from this region. In interviews, manufacturers indicated that a significant proportion of communication chips and chip boards were manufactured in Wuhan, China. Such a degree of dependency renders the entire supply chain vulnerable to disruptions, as was evident during the pandemic.
Another striking bottleneck was in raw materials essential for microprocessors. Expert interviews revealed that the majority of microprocessor raw materials originate from a single factory in Chinese Taipei. This degree of centralisation anywhere in the world poses significant risks, as any disruption, be it political, environmental, or economic, can halt production globally.
Overlapping regulations across jurisdictions add to the complexities faced by CPAP device manufacturers, as reported in expert interviews. Ongoing discussions by the US FDA, the UK regulatory agency, and their European and Asian counterparts, on harmonising regulatory frameworks have yet to yield comprehensive results. The recent transition from the Medical Device Directive (MDD) to the Medical Device Regulation (MDR) raised the bar in the European market, with potential consequences expected to include exit from the market by some manufacturers. In such circumstances, navigating regulatory frameworks becomes an added overhead, sometimes overshadowing core production challenges.
During unprecedented health crises, such as the recent pandemic, the specifications and requirements for CPAP devices may differ from the norm. The intrinsic design and function of CPAP devices, and indeed of many medical devices, are optimised for specific sets of conditions and use‑cases. For example, most CPAP machines designed by prominent manufacturers are not optimised for oxygen conservation, given that oxygen supply is not usually a limiting factor in developed countries. However, during health emergencies, oxygen conservation can become pivotal. This calls for rapid design alterations to cater to the emergent demand – a challenge that not all manufacturers may be sufficiently agile to accommodate.
Source: Based on consultations with experts in 2023.
Pharmaceuticals showed relatively greater resilience, although shortages occurred
Pharmaceutical supply chains showed relatively greater resilience in the face of such tremendous stress. Nevertheless, demand increased significantly for essential medicines used in acute care settings, such as anaesthetics, creating disruptions in supply and local shortages (Choo and Rajkumar, 2020[109]; Dey et al., 2021[110]; Gereffi, Pananond and Pedersen, 2022[111]). The OECD analysis in Chapter 11 of Ready for the Next Crisis? (OECD, 2023[8]) discusses the situation for propofol and azithromycin:
Propofol (an intravenous anaesthetic) supply chains were placed under pressure during the early stages of the pandemic, exacerbating existing shortages of anaesthetics. Several countries reported shortages, resulting in the need to request special importations from companies without domestic marketing authorisations. In the United States, many propofol shortages were reported in the American Society of Health-System Pharmacists’ shortage database10, citing issues due to increased demand and shipping delays.
Azithromycin (a macrolide antibiotic) manufacturers coped relatively well with increased demand during the pandemic, but depleted most of their inventories, leading to longer lead times (from 45‑90 days to 6 months). Export restrictions, such as bans or licensing requirements, were imposed on antibiotics, particularly azithromycin, aiming to prioritise domestic markets and prevent exports to other countries. These limitations, even with exceptions for export-specific medicines, caused delays and extra expenses for exporters resulting from approval procedures and border controls, thereby worsening shortages. Moreover, certain markets (e.g. Canada), faced greater challenges due to export bans and logistics issues, stemming from a lack of local production capacity.
New COVID‑19 vaccines were developed with exceptional speed over the course of the pandemic. While the topic of access and availability of these new vaccines is out of scope of this report, Box 1.8 explores some challenges in general vaccine supply chains during COVID‑19.
Box 1.8. Challenges to vaccine supply chains during COVID‑19
The World Health Organization’s 2022 Global Vaccine Market Report indicates that there is no evidence that the global patterns of reported non-COVID‑19 vaccine stockouts (i.e. lack of stock of a particular vaccine for a duration of at least one month) caused by quality issues were altered by the COVID‑19 pandemic, based on data from 2019, 2020 and 2021 (WHO, 2023[21]). However, a 2021 report by UNICEF highlighted issues with the manufacturers of certain non-COVID‑19 vaccines that UNICEF procures for non-OECD countries, citing competition with COVID‑19 vaccines for production and resource allocation (UNICEF Supply Division, 2021[112]). Other issues include difficulties in obtaining raw materials and consumables such as filters, vials and syringes, as well as limitations in skilled workforce and transport.
In the European Union, exports of COVID‑19 vaccines appeared to temporarily displace those of non-COVID‑19 vaccines, leading to reduced exports of these other vaccines in the first half of 2021. However, exports were back up to pre‑COVID‑19 levels by mid‑2021 – Figure 1.14 (OECD, 2022[28]).
Figure 1.14. Exports of vaccines (COVID‑19 and non-COVID‑19) from the EU, 2020-21
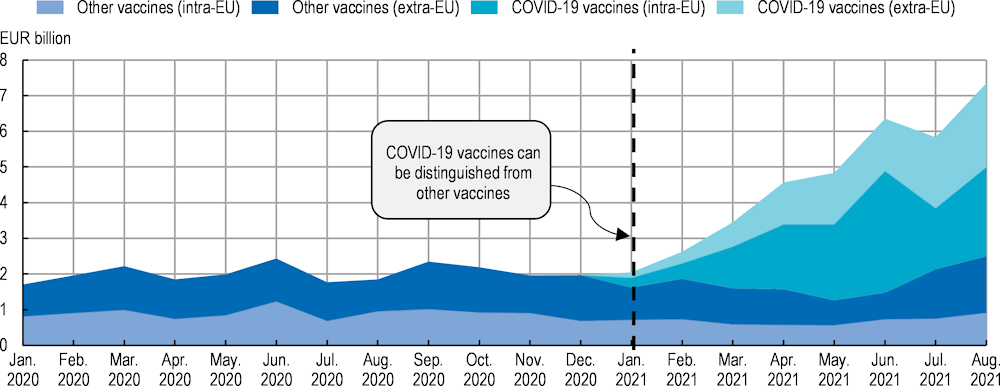
Note: The vertical line indicates the adoption of a separate nomenclature for COVID‑19 vaccines.
Source: Reproduced from OECD (2022[28]), “Global supply chains at work: A tale of three products to fight COVID‑19”, https://doi.org/10.1787/07647bc5-en.
1.3.3. Impact of conflicts and geopolitical tensions on medical supply chains in OECD countries
Regional conflicts can impact global supply chains. The closure of manufacturing plants, disruptions to trade routes, and diversion of the workforce are some of the direct consequences from wars that can affect the availability of essential products. For example, Russia’s war of aggression against Ukraine has caused severe disruptions in the global supply of products such as agricultural fertilisers or some critical raw materials for industrial production and the green transition (OECD, 2022[113]). Early analysis of suppliers’ vulnerability highlighted the risk of inflationary pressures and high oil prices affecting energy costs for manufacturers in many countries. Increase in costs and reduced availability of essential raw materials that had a considerable share of supplies coming from Ukraine and Russia could particularly affect the manufacturing of semiconductors, an important component in some medical devices. For instance, 90% of the highly purified, semiconductor-grade neon chip production used by the United States originates from Ukraine (Athanasia and Arcuri, 2022[114]).
Medical supply chains, however, have suffered less direct impacts from this conflict. The pharmaceutical sector is one of the less impacted when running simulations of trade disruptions on output, including within Ukraine and Russia (Arriola et al., 2023[115]). Nevertheless, there is a relative dependence of Russia on imported pharmaceutical products. Supply chain data also highlight impacts in the pharmaceutical sector of other countries, such as Latvia, through trade linkages.
At the same time, wide‑ranging and increasing sanctions against Russia are generating repeated shocks for pharmaceutical manufacturers – particularly those with plants or providers in that country. Although some exceptions have been put in place to facilitate the supply of medical products, obtaining licenses can be a cumbersome process, with different rules applying to each item, often causing delays and increased costs for firms. Disruptions to transportation routes and the risks of crossing conflict zones further add to the difficulties of maintaining the continuity of supply chains that involve Ukraine and Russia (Fassion, 2022[116]).
References
[33] Acosta, A. et al. (2019), “Medicine Shortages: Gaps Between Countries and Global Perspectives”, Frontiers in Pharmacology, Vol. 10, https://doi.org/10.3389/fphar.2019.00763.
[7] Aguiar, E. and K. Ernest (2021), Study of the trade flows of parallel imported medicines in Europe, Affordable Medicines Europe, https://affordablemedicines.eu/wp-content/uploads/2021/11/Trade-Flow-Study-2020-update.pdf (accessed on 8 December 2023).
[102] Amirian, E. (2022), “Prioritizing COVID-19 test utilization during supply shortages in the late phase pandemic.”, Journal of public health policy, Vol. 43/2, pp. 320-324, https://doi.org/10.1057/s41271-022-00348-8.
[115] Arriola, C. et al. (2023), “Challenges to international trade and the global economy: Recovery from COVID-19 and Russia’s war of aggression against Ukraine”, OECD Trade Policy Papers, No. 265, OECD Publishing, Paris, https://doi.org/10.1787/5c561274-en.
[40] ASHP (2023), Drug Shortages Statistics, American Society of Health-System Pharmacists, https://www.ashp.org/drug-shortages/shortage-resources/drug-shortages-statistics (accessed on 8 December 2023).
[114] Athanasia, G. and G. Arcuri (2022), Russia’s Invasion of Ukraine Impacts Gas Markets Critical to Chip Production, https://www.csis.org/blogs/perspectives-innovation/russias-invasion-ukraine-impacts-gas-markets-critical-chip-production (accessed on 8 December 2023).
[2] BACI (CEPII) (2023), BACI Trade data, http://www.cepii.fr/CEPII/en/bdd_modele/bdd_modele_item.asp?id=37 (accessed on 8 December 2023).
[99] Baldwin, R. and R. Freeman (2020), Supply chain contagion waves: Thinking ahead on manufacturing ‘contagion and reinfection’ from the COVID concussion, CEPR, https://cepr.org/voxeu/columns/supply-chain-contagion-waves-thinking-ahead-manufacturing-contagion-and-reinfection (accessed on 15 November 2023).
[73] BD (2023), BD Vacutainer Product Updates, https://go.bd.com/vacutainer-supply-update.html (accessed on 8 December 2023).
[103] Behnam, M. et al. (2020), COVID-19: Overcoming supply shortages for diagnostic testing, McKinsey & Company, https://www.mckinsey.com/industries/life-sciences/our-insights/covid-19-overcoming-supply-shortages-for-diagnostic-testing (accessed on 8 December 2023).
[66] Beleche, T. et al. (2022), “Characteristics Of Medical Device Shortages In The US, 2006–20”, Health Affairs, Vol. 41/12, pp. 1790-1794, https://doi.org/10.1377/hlthaff.2022.00643.
[47] Benhabib, A. et al. (2020), “The French reporting system for drug shortages: description and trends from 2012 to 2018: an observational retrospective study”, BMJ Open, Vol. 10/3, p. e034033, https://doi.org/10.1136/bmjopen-2019-034033.
[43] Beraud, G. (2021), “Shortages Without Frontiers: Antimicrobial Drug and Vaccine Shortages Impact Far Beyond the Individual!”, Frontiers in Medicine, Vol. 8, https://doi.org/10.3389/fmed.2021.593712.
[78] Better2Know (2021), With a shortage of blood test tubes affecting the UK, Better2Know is continuing to test as normal, https://www.better2know.co.uk/blog/shortage-of-test-tubes-affecting-the-uk/ (accessed on 8 December 2023).
[19] Bown, C. and T. Bollyky (2021), “How COVID‐19 vaccine supply chains emerged in the midst of a pandemic”, The World Economy, Vol. 45/2, pp. 468-522, https://doi.org/10.1111/twec.13183.
[12] Brand, A. et al. (2021), “Review of indications for immunoglobulin (IG) use: Narrowing the gap between supply and demand”, Transfusion Clinique et Biologique, Vol. 28/1, pp. 96-122, https://doi.org/10.1016/j.tracli.2020.12.005.
[23] Cao, W., D. Du and Q. Xia (2023), “Unbalanced global vaccine product trade pattern: A network perspective”, Social Science & Medicine, https://doi.org/10.1016/j.socscimed.2023.115913.
[70] CDC (2022), Blood Lead Collection Tube Shortage, Centers for Disease Control and Prevention, https://www.cdc.gov/nceh/lead/news/blood-lead-collection-tube-shortage.html (accessed on 8 December 2023).
[106] CDC (2021), Lab Advisory: Shortage of COVID-19 Rapid Tests May Increase Demand for Laboratory Testing, Centers for Disease Control and Prevention, https://www.cdc.gov/locs/2021/09-02-2021-lab-advisory-Shortage_COVID-19_Rapid_Tests_Increase_Demand_Laboratory_Testing_1.html (accessed on 8 December 2023).
[93] CDC (2010), CDC H1N1 Flu | Interim Guidance on Infection Control Measures for 2009 H1N1 Influenza in Healthcare Settings, Including Protection of Healthcare Personnel, Centers for Disease Control and Prevention, https://www.cdc.gov/h1n1flu/guidelines_infection_control.htm (accessed on 20 November 2023).
[1] Chapman, S., G. Dedet and R. Lopert (2022), “Shortages of medicines in OECD countries”, OECD Health Working Papers, No. 137, OECD Publishing, Paris, https://doi.org/10.1787/b5d9e15d-en.
[26] Chen, P. et al. (2021), Medical Device Supply Chains: An Overview and Description of Challenges During the COVID-19 Pandemic, RAND Health Care, https://aspe.hhs.gov/reports/medical-device-supply-chains (accessed on 23 November 2023).
[29] Chen, Z., Z. Hu and H. Dai (2012), “Control system design for a continuous positive airway pressure ventilator”, BioMedical Engineering OnLine, Vol. 11/1, p. 5, https://doi.org/10.1186/1475-925x-11-5.
[109] Choo, E. and S. Rajkumar (2020), “Medication Shortages During the COVID-19 Crisis”, Mayo Clinic Proceedings, Vol. 95/6, pp. 1112-1115, https://doi.org/10.1016/j.mayocp.2020.04.001.
[36] Chorba, T. (2023), TB Drug Supply Interruptions and Shortages, https://www.cdc.gov/tb/publications/letters/2023/tb-drug-shortage.html (accessed on 5 November 2023).
[105] Congressional Research Service (2021), COVID-19 Testing Supply Chain, https://crsreports.congress.gov/product/pdf/IF/IF11774 (accessed on 30 January 2024).
[64] Covington, M., C. Voma and S. Stowell (2022), “Shortage of plasma-derived products: a looming crisis?”, Blood, Vol. 139/21, pp. 3222-3225, https://doi.org/10.1182/blood.2021015370.
[48] de Vries, H. et al. (2021), “Short of drugs? Call upon operations and supply chain management”, International Journal of Operations and Production Management, Vol. 41/10, pp. 1569-1578, https://doi.org/10.1108/ijopm-03-2021-0175.
[110] Dey, B. et al. (2021), “A ripple effect of covid-19 pandemic on shortage of medicinal products and its impact on patient care”, International Journal of Applied Pharmaceutics, Vol. 13/5, pp. 364-370, https://doi.org/10.22159/ijap.2021v13i5.42177.
[52] Di Giorgio, D. et al. (2019), “Tackling distribution-related shortages of medicines: An Italian case study evaluated in the European Union framework”, Medicine Access @ Point of Care, Vol. 3, p. 239920261985685, https://doi.org/10.1177/2399202619856859.
[107] Ding, J. (2022), “Why are rapid tests for COVID-19 in such short supply?”, Los Angeles Times, https://www.latimes.com/business/story/2022-01-08/why-are-rapid-tests-for-covid-19-in-such-short-supply (accessed on 30 January 2024).
[3] Drevinskas, E., E. Shing and T. Verbeet (2023), Trade in medical goods stabilises after peaking during pandemic, WTO Data Blog, https://www.wto.org/english/blogs_e/data_blog_e/blog_dta_23may23_e.htm (accessed on 8 December 2023).
[56] EAHP (2023), EAHP 2023 Shortages Survey Report, European Association of Hospital Pharmacists, https://www.eahp.eu/sites/default/files/shortages_survey_report_final.pdf (accessed on 8 December 2023).
[59] ECDC (2017), Rapid risk assessment: Hepatitis A outbreak in the EU/EEA mostly affecting men who have sex with men, 3rd update, 28 June 2017, https://www.ecdc.europa.eu/en/publications-data/rapid-risk-assessment-hepatitis-outbreak-eueea-mostly-affecting-men-who-have-sex (accessed on 17 October 2023).
[58] ECDC (2016), Rapid Risk Assessment: Shortage of acellular pertussis-containing vaccines and impact on immunisation programmes in the EU/EEA (first update), European Centre for Disease Prevention and Control, https://www.ecdc.europa.eu/en/publications-data/rapid-risk-assessment-shortage-acellular-pertussis-containing-vaccines-and-impact (accessed on 17 October 2023).
[79] ECHA (2023), ECHA publishes PFAS restriction proposal, European Chemicals Agency, https://echa.europa.eu/-/echa-publishes-pfas-restriction-proposal (accessed on 8 December 2023).
[80] ECHA (2022), Annex XV Restriction Report, European Chemicals Agency, https://echa.europa.eu/documents/10162/1c480180-ece9-1bdd-1eb8-0f3f8e7c0c49 (accessed on 8 December 2023).
[37] Edwards, S. et al. (2023), Tackling TB in Europe: Treatment gaps and deteriorating treatment availability is undermining progress, https://theunion.org/news/tackling-tb-in-europe-treatment-gaps-and-deteriorating-treatment-availability-is-undermining-progress (accessed on 30 January 2024).
[96] Elbe, S., A. Roemer-Mahler and C. Long (2014), “Securing circulation pharmaceutically: Antiviral stockpiling and pandemic preparedness in the European Union”, Security dialogue, Vol. 45/5, pp. 440-457, https://doi.org/10.1177/0967010614530072.
[44] EMA (2023), Shortage of amoxicillin and amoxicillin/clavulanic acid, European Medicines Agency, https://www.ema.europa.eu/en/news/ema-update-shortages-antibiotics-eu (accessed on 30 January 2024).
[97] EMA (2009), European Medicines Agency recommendations on extension of shelf life for Tamiflu, European Medicines Agency, https://www.ema.europa.eu/en/news/european-medicines-agency-recommendations-extension-shelf-life-tamiflu (accessed on 21 November 2023).
[87] European Academy of Paediatrics (2023), Open letter: Urgent action needed to secure continued access to essential medical devices for children and for patients with orphan diseases, https://www.eapaediatrics.eu/wp-content/uploads/2023/06/Letter-Kyriakides_Med-Devices-signed-270627.pdf (accessed on 22 November 2023).
[88] European Commission (2023), NANDO database regulation (EU) 2017/745 on medical devices, https://webgate.ec.europa.eu/single-market-compliance-space/#/notified-bodies/notified-body-list?filter=legislationId:34 (accessed on 22 November 2023).
[89] European Commission (2023), “Notified Bodies Survey on certifications and applications (MDR/IVDR)”, https://health.ec.europa.eu/latest-updates/updated-document-notified-bodies-survey-certifications-and-applications-mdrivdr-survey-results-data-2023-07-25_en (accessed on 22 November 2023).
[85] European Commission (2021), Q&A: New In Vitro Diagnostic Medical Devices Regulation, https://ec.europa.eu/commission/presscorner/detail/en/qanda_21_5210 (accessed on 22 November 2023).
[24] Evenett, S. et al. (2021), The Covid-19 Vaccine Production Club - Will Value Chains Temper Nationalism?, World Bank, Washington DC, https://openknowledge.worldbank.org/handle/10986/35244 (accessed on 30 January 2024).
[116] Fassion, J. (2022), Unprecedented Sanctions Against Russia Continue to Impact Pharma Supply Chains, SIDLEY, https://goodlifesci.sidley.com/2022/10/25/unprecedented-sanctions-against-russia-continue-to-impact-pharma-supply-chains/ (accessed on 24 November 2023).
[5] FDA (2023), Annual reporting by Prescription Drug Wholesale Distributors and Third-Party Logistics Providers, https://www.fda.gov/drugs/drug-supply-chain-security-act-dscsa/annual-reporting-prescription-drug-wholesale-distributors-and-third-party-logistics-providers (accessed on 29 January 2024).
[108] FDA (2021), UPDATE: Certain Philips Respironics Ventilators, BiPAP Machines, and CPAP Machines Recalled Due to Potential Health Risks: FDA Safety Communication, U.S. Food and Drug Administration, https://www.fda.gov/medical-devices/safety-communications/update-certain-philips-respironics-ventilators-bipap-machines-and-cpap-machines-recalled-due (accessed on 30 January 2024).
[46] FDA (2019), Drug Shortages: Root Causes and Potential Solutions, U.S. Food and Drug Administration, https://www.fda.gov/drugs/drug-shortages/report-drug-shortages-root-causes-and-potential-solutions (accessed on 4 July 2023).
[95] Fidler, D. (2010), “Negotiating equitable access to influenza vaccines: global health diplomacy and the controversies surrounding avian influenza H5N1 and pandemic influenza H1N1.”, PLoS medicine, Vol. 7/5, p. e1000247, https://doi.org/10.1371/journal.pmed.1000247.
[53] Filia, A. et al. (2022), “Are vaccine shortages a relevant public health issue in Europe? Results from a survey conducted in the framework of the EU Joint Action on Vaccination”, Vaccine, Vol. 40/13, pp. 1987-1995, https://doi.org/10.1016/j.vaccine.2022.02.041.
[50] Frank, R., T. McGuire and I. Nason (2021), “The Evolution of Supply and Demand in Markets for Generic Drugs”, The Milbank Quarterly, Vol. 99/3, pp. 828-852, https://doi.org/10.1111/1468-0009.12517.
[111] Gereffi, G., P. Pananond and T. Pedersen (2022), “Resilience Decoded: The Role of Firms, Global Value Chains, and the State in COVID-19 Medical Supplies”, California Management Review, Vol. 64/2, pp. 46-70, https://doi.org/10.1177/00081256211069420.
[6] GIRP (2022), Annual report 2021-2022, European Healthcare Distribution Association, https://girp.eu/sites/default/files/2022-06/GIRP%20Annual%20Report%202021-2022.pdf (accessed on 8 December 2023).
[69] Gosselin, R. et al. (2021), “Guidance on the critical shortage of sodium citrate coagulation tubes for hemostasis testing”, Journal of Thrombosis and Haemostasis, Vol. 19/11, pp. 2857-2861, https://doi.org/10.1111/jth.15514.
[71] Government of Canada (2022), Medical device shortage: BD Vacutainer Tubes, https://www.canada.ca/en/health-canada/services/drugs-health-products/medical-devices/shortages/list/covid-19-bd-vacutainer-tubes.html%203 (accessed on 8 December 2023).
[104] Griffin, S. (2020), “Covid-19: Supply chain problems could delay NHS tests”, BMJ, p. m3916, https://doi.org/10.1136/bmj.m3916.
[83] Haute Autorité de Santé (2022), Commission nationale d’évaluation des dispositifs médicaux et des technologies de santé - Rapport d’activité, https://www.has-sante.fr/upload/docs/application/pdf/2023-06/rapport_dactivite_2022_de_la_cnedimts.pdf (accessed on 10 November 2023).
[65] Hess, J. (2010), “Conventional blood banking and blood component storage regulation: opportunities for improvement”, Blood Transfusion, pp. 9-15, https://doi.org/10.2450/2010.003S.
[94] Institute of Medicine (US) Forum on Medical and Public Health Preparedness for Catastrophic Events (2010), The 2009 H1N1 Influenza Vaccination Campaign: Summary of a Workshop Series., National Academies Press (US), Washington, D.C., https://www.ncbi.nlm.nih.gov/books/NBK54181/ (accessed on 21 November 2023).
[51] IQVIA (2023), Drug Shortages in the U.S. 2023, https://www.iqvia.com/-/media/iqvia/pdfs/institute-reports/drug-shortages-in-the-us-2023/drug-shortages-in-the-us-2023.pdf (accessed on 5 December 2023).
[38] Jongh, T. et al. (2021), Future-proofing pharmaceutical legislation - Study on medicine shortages - Final report (revised), Publications Office of the European Union, https://data.europa.eu/doi/10.2875/211485.
[16] Kluszczynski, E., S. Rohr and R. Ernst (2020), Key Economic and Value Consierations for Plasma-Derived Meicinal Products (PDMPs) in Europe, Vintura, https://www.vintura.com/news/white-paper-key-economic-value-considerations-plasma-derived-medicinal-products-pdmps-europe (accessed on 4 July 2023).
[41] Lau, B. et al. (2022), “COVID-19 and the prevalence of drug shortages in Canada: a cross-sectional time-series analysis from April 2017 to April 2022”, Canadian Medical Association Journal, Vol. 194/23, pp. E801-E806, https://doi.org/10.1503/cmaj.212070.
[17] Marketing Research Bureau (2023), Marketing Research Bureau, https://marketingresearchbureau.com/global-blood-plasma-collection-and-use-2021-2022/ (accessed on 30 January 2024).
[82] Medical Device Network (2022), Medical devices that obtained US approval in April 2022: Exclusive analysis, https://www.medicaldevice-network.com/marketdata/medical-devices-us-approval-april-2022/ (accessed on 10 November 2023).
[84] MedTech Europe (2016), The European Medical Technology Industry in figures, http://www.medtecheurope.org/sites/default/files/resource_items/files/MedTech_FactsFigures2016_20160105.pdf (accessed on 10 October 2023).
[55] Miljković, N. et al. (2019), “Results of EAHP’s 2018 Survey on Medicines Shortages”, European Journal of Hospital Pharmacy, Vol. 26/2, pp. 60-65, https://doi.org/10.1136/ejhpharm-2018-001835.
[92] Murray, M. et al. (2010), “Facial Protective Equipment, Personnel, and Pandemics: Impact of the Pandemic (H1N1) 2009 Virus on Personnel and Use of Facial Protective Equipment”, Infection Control & Hospital Epidemiology, Vol. 31/10, pp. 1011-1016, https://doi.org/10.1086/656564.
[76] NHS (2021), Becton Dickinson blood specimen collection - supply disruption, https://www.rcpath.org/static/633b7d26-dbe8-440a-8ba5596170f40634/B0933-BD-blood-collection-supply-Disruption.pdf (accessed on 8 December 2023).
[74] NHS (2021), Becton Dickinson Blood Specimen Collection Portfolio supply disruption: Recommended actions for medical directors, nursing directors, GPs and pathology laboratories to optimise resources for pathology laboratory work, https://www.england.nhs.uk/wp-content/uploads/2021/08/B0888-becton-dickinson-blood-specimen-collection-portfolio-supply-disruption-v2.pdf (accessed on 8 December 2023).
[45] OECD (2023), Embracing a One Health Framework to Fight Antimicrobial Resistance, OECD Health Policy Studies, OECD Publishing, Paris, https://doi.org/10.1787/ce44c755-en.
[4] OECD (2023), Inter-Country Input-Output (ICIO) Tables, https://www.oecd.org/sti/ind/inter-country-input-output-tables.htm (accessed on 8 December 2023).
[8] OECD (2023), Ready for the Next Crisis? Investing in Health System Resilience, OECD Health Policy Studies, OECD Publishing, Paris, https://doi.org/10.1787/1e53cf80-en.
[28] OECD (2022), “Global supply chains at work: A tale of three products to fight COVID-19”, OECD Policy Responses to Coronavirus (COVID-19), OECD Publishing, Paris, https://doi.org/10.1787/07647bc5-en.
[113] OECD (2022), “The supply of critical raw materials endangered by Russia’s war on Ukraine”, OECD Policy Responses on the Impacts of the War in Ukraine, OECD Publishing, Paris, https://doi.org/10.1787/e01ac7be-en.
[18] OECD (2021), “Using trade to fight COVID-19: Manufacturing and distributing vaccines”, OECD Policy Responses to Coronavirus (COVID-19), OECD Publishing, Paris, https://doi.org/10.1787/dc0d37fc-en.
[100] OECD (2020), “COVID-19 and global value chains: Policy options to build more resilient production networks”, OECD Policy Responses to Coronavirus (COVID-19), OECD Publishing, Paris, https://doi.org/10.1787/04934ef4-en.
[27] OECD (2020), “The face mask global value chain in the COVID-19 outbreak: Evidence and policy lessons”, OECD Policy Responses to Coronavirus (COVID-19), OECD Publishing, Paris, https://doi.org/10.1787/a4df866d-en.
[63] OECD/NEA (2019), The Supply of Medical Isotopes: An Economic Diagnosis and Possible Solutions, OECD Publishing, Paris, https://doi.org/10.1787/9b326195-en.
[77] Osborne, S. (2021), “GPs told to suspend some blood tests as tube shortage worsens”, PULSE, https://www.pulsetoday.co.uk/news/clinical-areas/allergy/gps-told-to-suspend-some-blood-tests-as-tube-shortage-worsens/ (accessed on 8 December 2023).
[62] Pasté, M. et al. (2022), “Addressing vaccine supply challenges in Europe: Expert industry perspective and recommendations”, Health Policy, Vol. 126/1, pp. 35-42, https://doi.org/10.1016/j.healthpol.2021.11.006.
[54] PGEU (2022), Medicine Shortages - PGEU Survey 2022 Results, Pharmaceutical Group of the European Union, https://www.pgeu.eu/wp-content/uploads/2023/01/Medicine-Shortages-PGEU-Survey-2022-Results-1.pdf (accessed on 7 November 2023).
[15] PPTA (2022), EU needs effective measures to facilitate access to lifesaving medicines made of human plasma (PDMPs): PPTA position statement on stockpiling, Plasma Protein Therapeutics Association, https://www.euneedsmoreplasma.com/images/files/euregwg22057a_ppta%20position%20statement%20on%20stockpiling_final_05.08.2022.pdf (accessed on 4 July 2023).
[72] Raiken, A. (2022), “’Widespread disruption’ of STD testing has begun due to lack of blood sample tubes”, Independent, https://www.independent.co.uk/life-style/std-testing-shortage-test-tubes-b2017759.html (accessed on 8 December 2023).
[39] Ravela, R., M. Airaksinen and A. Lyles (2023), “State of the European Union’s early notification obligation for drug shortages: enforcement and compliance in eight European countries (2020–2022)”, Journal of Pharmaceutical Policy and Practice, Vol. 16/1, https://doi.org/10.1186/s40545-023-00646-w.
[67] Rimmer, A. (2021), “What has caused the NHS blood tube shortage, and how is it affecting doctors and patients?”, BMJ, p. n2174, https://doi.org/10.1136/bmj.n2174.
[42] Sabogal De La Pava, M. and E. Tucker (2022), “Drug shortages in low- and middle-income countries: Colombia as a case study”, Journal of Pharmaceutical Policy and Practice, Vol. 15/1, p. 42, https://doi.org/10.1186/s40545-022-00439-7.
[13] Schmidt, A. and M. Refaai (2022), “Blood derivative therapy”, Annals of Blood, Vol. 7, pp. 7-7, https://doi.org/10.21037/aob-21-64.
[86] Shatrov, K. and C. Blankart (2022), “After the four-year transition period: Is the European Union’s Medical Device Regulation of 2017 likely to achieve its main goals?”, Health Policy, Vol. 126/12, pp. 1233-1240, https://doi.org/10.1016/J.HEALTHPOL.2022.09.012.
[81] Snitem (2022), Panorama et analyse qualitative de la filière industrielle des dispositif médical en France en 2021, https://www.snitem.fr/publications/guides-et-documents-de-reference/panorama-des-entreprises-du-dispositif-medical-en-2021/ (accessed on 3 August 2023).
[91] Sodhi, M. and C. Tang (2021), “Rethinking industry’s role in a national emergency”, MIT Sloan Management Review Summer, https://sloanreview.mit.edu/article/rethinking-industrys-role-in-a-national-emergency/.
[90] Sodhi, M. and C. Tang (2021), “Supply Chain Management for Extreme Conditions: Research Opportunities”, Journal of Supply Chain Management, Vol. 57/1, pp. 7-16, https://doi.org/10.1111/jscm.12255.
[10] Strengers, P. (2023), “Challenges for Plasma-Derived Medicinal Products”, Transfusion Medicine and Hemotherapy, Vol. 50/2, pp. 116-122, https://doi.org/10.1159/000528959.
[11] Strengers, P. (2017), “Evidence-based clinical indications of plasma products and future prospects”, Annals of Blood, pp. 1-7, https://doi.org/10.21037/aob.2017.12.03.
[61] TGA (2023), Medicine shortage reports database, Therapeutic Goods Administration, https://apps.tga.gov.au/Prod/msi/search?shortagetype=All (accessed on 8 December 2023).
[75] The Royal College of Pathologists (2021), Supply Disruption of BD Vacutainer Blood Specimen Collection Tubes, https://www.rcpath.org/profession/on-the-agenda/supply-disruption-of-bd-vacutainer-blood-specimen-collection-tubes.html (accessed on 8 December 2023).
[34] Troein, P. et al. (2020), Reporting of Medicine Shortages in Europe [White Paper], IQVIA, https://www.iqvia.com/library/white-papers/reporting-of-medicine-shortages-in-europe (accessed on 19 January 2021).
[68] Tsang, P., S. Absar and D. Gingrich (2021), “Applying Best Practice to Converting Blood Collection Tube Suppliers: Overcoming Laboratory Supply Shortages”, American Journal of Clinical Pathology, Vol. 156/Supplement_1, pp. S148-S149, https://doi.org/10.1093/ajcp/aqab191.316.
[112] UNICEF Supply Division (2021), COVID-19 Impact Assessment on Global Logistics and Supplies, https://www.unicef.org/supply/media/9741/file/COVID-19-Impact-on-Global-Logistics-and-Supplies-September-2021.pdf.
[9] US Congress (2018), Committee on Energy and Commerce: Letter to the Commissioner of FDA The Honorable Scott Gottlieb, M.D., https://www.fdanews.com/ext/resources/files/2018/02-02-18-Heparin.pdf?1518507802 (accessed on 23 January 2023).
[98] US Government Accountability Office (2011), Lessons from the H1N1 Pandemic Should Be Incorporated into Future Planning, https://www.gao.gov/assets/gao-11-632.pdf (accessed on 21 November 2023).
[20] Vaccines Europe (2020), How are vaccines produced?, https://www.vaccineseurope.eu/about-vaccines/how-are-vaccines-produced (accessed on 14 November 2023).
[22] Vaccines Europe (2019), The EU Vaccine Industry in Figures, https://www.vaccineseurope.eu/about-us/the-eu-vaccine-industry-in-figures/ (accessed on 17 November 2023).
[35] WHO (2023), As European Region continues positive trend in TB control, WHO/Europe warns of challenges that could threaten gains, World Health Organization, https://www.who.int/europe/news/item/22-09-2023-as-european-region-continues-positive-trend-in-tb-control-who-europe-warns-of-challenges (accessed on 5 November 2023).
[21] WHO (2023), Global vaccine market report 2022: a shared understanding for equitable access to vaccines, World Health Organization, https://iris.who.int/handle/10665/367213.
[25] WHO (2023), Health products policy and standards, World Health Organization, https://www.who.int/teams/health-product-policy-and-standards/assistive-and-medical-technology/medical-devices/nomenclature (accessed on 8 December 2023).
[14] WHO (2023), Web Annex A. World Health Organization Model List of Essential Medicines - 23rd List, 2023, World Health Organization, https://www.who.int/publications/i/item/WHO-MHP-HPS-EML-2023.02.
[30] WHO (2020), Priority medical devices list for the COVID-19 response and associated technical specifications, World Health Organization, https://www.who.int/publications/i/item/WHO-2019-nCoV-MedDev-TS-O2T.V2.
[101] WHO (2020), Shortage of personal protective equipment endangering health workers worldwide, World Health Organization, https://www.who.int/news/item/03-03-2020-shortage-of-personal-protective-equipment-endangering-health-workers-worldwide (accessed on 20 November 2023).
[60] WHO (2017), Disease Outbreak News: 2017 - United States of America, World Health Organization, https://www.who.int/emergencies/disease-outbreak-news/item/07-june-2017-hepatitis-a-en (accessed on 17 October 2023).
[32] WHO (2017), Meeting Report: Technical Definitions of Shortages and Stockouts of Medicines and Vaccines, World Health Organization, https://cdn.who.int/media/docs/default-source/medicines/meeting_report_october_shortages.pdf?sfvrsn=1a902eab_3&download=true (accessed on 6 November 2023).
[31] World Health Assembly (2017), Addressing the global shortage of, and access to, medicines and vaccines: report by the Secretariat, World Health Organization, https://iris.who.int/handle/10665/274799.
[49] Yurukoglu, A., E. Liebman and D. Ridley (2017), “The Role of Government Reimbursement in Drug Shortages”, American Economic Journal: Economic Policy, Vol. 9/2, pp. 348-382, https://doi.org/10.1257/pol.20160035.
[57] Ziesenitz, V. et al. (2017), “U.S. vaccine and immune globulin product shortages, 2001–15”, American Journal of Health-System Pharmacy, Vol. 74/22, pp. 1879-1886, https://doi.org/10.2146/ajhp170066.
Notes
← 1. For example, see the European Medicines Agency’s responsibilities by type of medical device at the following link: www.ema.europa.eu/en/human-regulatory/overview/medical-devices (last accessed 21 November 2023).
← 2. Biologicals are medicines manufactured in, or sourced from, living systems such as microorganisms, or plant or animal cells. Most biologicals are very large, complex molecules or mixtures of molecules. For more details, see www.who.int/health-topics/biologicals (last accessed 7 August 2023).
← 3. With the Harmonised System (HS), a standardised classification of 5 000 products at the six‑digit level developed by the World Customs Organization.
← 4. In this chart, the European Union is regarded as a single economy, with “domestic” value‑added including intra-EU transactions.
← 5. Wholesaler activity consists of the purchase, warehousing, storage, order preparation and delivery of medicines. Pharmaceutical full-line wholesalers carry and distribute the complete assortment of products in range and depth within the framework set by the authorities and the market to meet the needs of those with whom they have normal business relations.
← 6. Information provided by GIRP, the European Healthcare Distribution Association, via personal correspondence in 2023.
← 7. Market value, at ex-factory prices. See https://girp.eu/sites/default/files/2022-06/GIRP%20Annual%20Report%202021-2022.pdf.
← 8. Commercial reasons were defined in the report as “company-driven decisions linked to business aspects such as pricing negotiations; discontinuation; change in reimbursement status; low sales (i.e. low number of patients); business strategies prioritising other markets” (Jongh et al., 2021[38]).
← 9. For Belgium, the observation period was July 2022–November 2022.
← 10. These data are from the American Society of Health-System Pharmacists (ASPH) Drug Shortages website and include shortages that do not meet the criteria defined by the US FDA. For example, FDA only lists shortages on its website once it is confirmed that overall market demand is not met by the manufacturers, while the ASHP lists those in shortage even if full market demand is met. ASHP therefore commonly lists more shortages than FDA. Explanations of these differences are available at www.ashp.org/drug-shortages/current-shortages/fda-and-ashp-shortage-parameters?loginreturnUrl=SSOCheckOnly, last accessed 29 January 2024.
← 11. The is calculated as the sum of the squares or market shares of each supplier (HHI=s12 + s22 + s32 +... + sn2, where si represents the market share (%) of supplier i). It can range from close to 0 to 10 000, where the latest represents a single supplier.
← 12. An extended unit is defined as a millilitre for some injections, and as a pill for oral solids (see Definitions for more details).
← 13. DT-containing vaccines protect against diptheria (D) and tetanus (T). dT-containing vaccines have a reduced dose of dipetheria.
← 14. Based on archived data downloaded from Australia’s medicines shortages register on 19 November 2023 (TGA, 2023[61]). For more information see www.tga.gov.au/safety/shortages.
← 15. “Global shortages” were reported by 13 countries, and reported for BCG vaccine, DT-containing vaccines, hepatitis A, adult hepatitis B, and rabies vaccines.
← 16. Vaccine’s Europe is a specialised group within the European Federation of Pharmaceutical Industries and Associations (EFPIA).
← 17. For example, see the requirements for notifying the FDA about medical device shortages at the following link: www.fda.gov/medical-devices/medical-device-safety/medical-device-supply-chain-and-shortages#authority (last accessed 18 January 2024).
← 18. A 510(k) is a premarket submission made to FDA to demonstrate that the device to be marketed is as safe and effective, that is, substantially equivalent, to a legally marketed device (Section 513(i)(1)(A) FD&C Act).