This chapter describes the major sources and entry-pathways of pharmaceuticals into the environment and the environmental implications.
Management of Pharmaceutical Household Waste
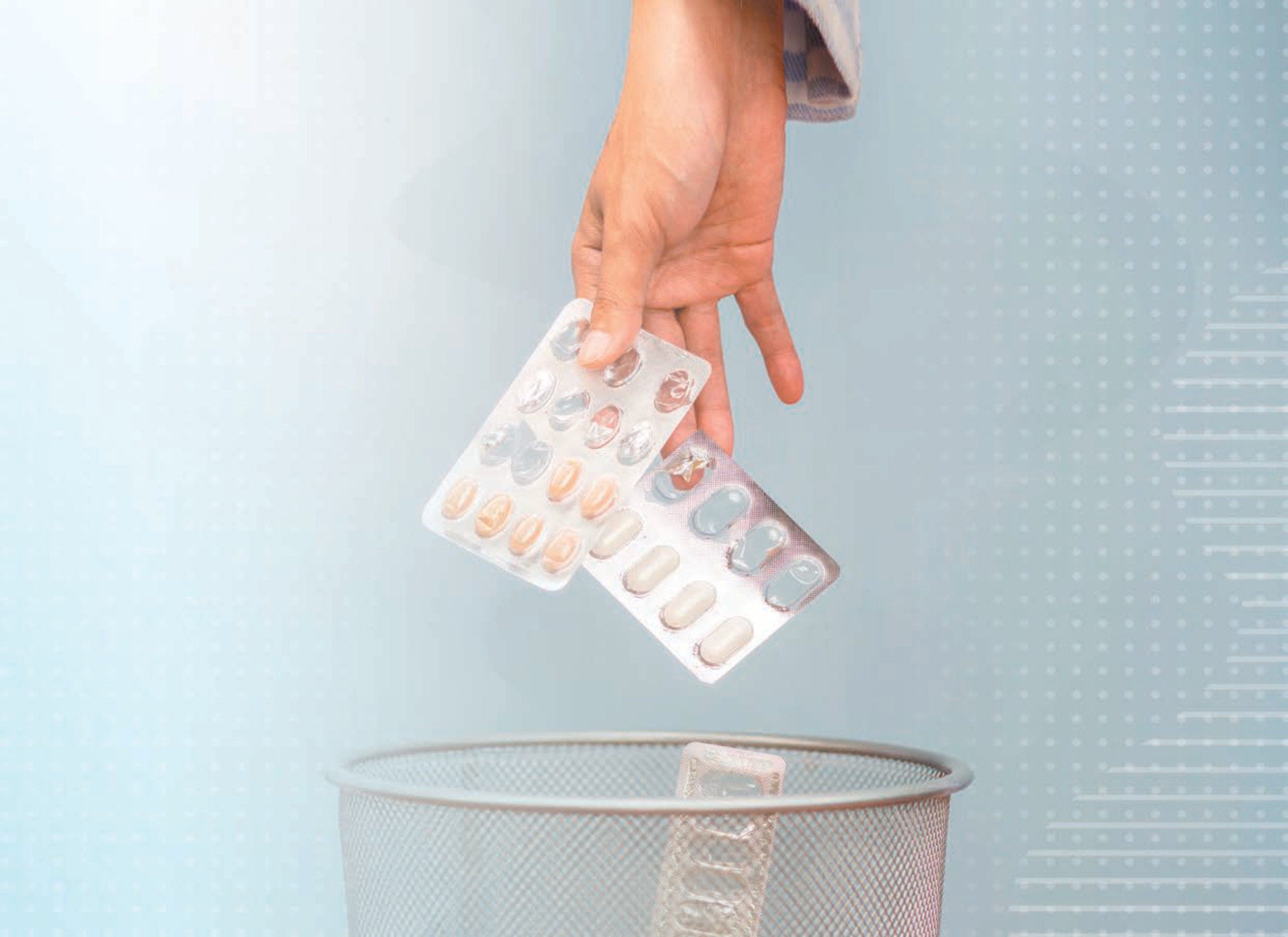
2. Sources and entry-pathways of pharmaceuticals into the environment
Abstract
1.1. Sources
Pharmaceuticals and their metabolites can enter the environment during production, consumption and disposal (Kümmerer, 2009[16]; Lapworth et al., 2012[17]). The following are the key sources.
2.1.1. Households
Excreted pharmaceuticals after consumption make up the largest source of household emissions. Between 30-90% of the oral doses of pharmaceuticals are generally excreted either as original compound or as metabolite. Creams and ointments washed off skin may also end up in wastewater (BIO Intelligence Service, 2013[7]).
Medicine that expires or remains unused is a significant waste stream and when disposed of improperly can also contribute to household emissions. An estimated 3-50% of pharmaceuticals become waste (Chapter 3, Section 3.1). Unused or expired medicines that are disposed of via bathroom sinks and toilets is one source of UEM emission to wastewater. Disposal of UEM via municipal solid waste destined for final disposal in landfills can also lead to leaching of pharmaceutical residues over time, if this leachate is not captured and treated appropriately (Masoner et al., 2014[18]).
Box 2.1. Definition of household disposal and final disposal
Literature and legislation use the term “disposal” for two different practices, at different parts of the waste value chain:
Household disposal refers to the disposal practices of unused or expired drugs by consumers and households (e.g. flushing, household bin, return to collection points, dumping)
Final disposal refers to the treatment practices of municipal solid waste in a given country (e.g. landfilling or incineration of collected UEM)
2.1.2. Hospitals
Hospitals, healthcare services and long-term care facilities are point sources of pharmaceutical substances going into the sewage network. Whilst the pharmaceutical loading of their wastewater is high, their contribution to the overall pharmaceutical contamination of wastewater varies per type of medicine. According to studies in Sweden and Norway, contributions lie around 1-3% for most pharmaceuticals, as much medication prescribed in hospitals is consumed at home and advanced care increasingly takes place in private homes (Thomas et al., 2007[19]; Larsson and Lööf, 2016[20]).1 Another study estimates the contribution of hospitals to the environmental load in the EU at about 10% (Kümmerer and Hempel, 2010[21]). In Denmark’s Copenhagen region, an estimated 24% of the total antibiotic load originates from hospitals. For hospital specific substances such as cytostatic or endocrine medicines or contrast media, the contribution can be higher (BIO Intelligence Service, 2013[7]).
2.1.3. Pharmaceutical production
Industrial chemical residues from manufacturing APIs and pharmaceuticals can enter the water cycle through direct discharge (i.e. industrial wastewater) and indirect discharge (in case of leakage). Globally, this source is considered low compared to the amount excreted by patients, but local hot spots can exist.
2.1.4. Veterinary pharmaceuticals
Pharmaceuticals for veterinary use, aquaculture and agriculture present an important source of pharmaceuticals entering the environment. Similar to human consumption, 30-90% of the pharmaceutical consumed by animals is excreted as original compound or metabolite in animal faeces. Veterinary pharmaceuticals in aquaculture directly enter waterbodies, whereas the common reuse of livestock manure leads to entry pathways into the soil, surface water and groundwater.2
Figure 2.1. Main sources and pathways of human pharmaceutical residues to the environment
2.2. Entry pathways
The key entry pathways into freshwater and terrestrial ecosystems are the following.
2.2.1. Wastewater treatment plants (WWTP)
Conventional WWTPs are not designed to remove all pharmaceuticals, resulting in emissions into waterbodies or land in unchanged or metabolised form, if refined water is reused for agriculture (Box 2.2) (Behera et al., 2011[22]; Hollender et al., 2009[23]; Melvin and Leusch, 2016[24]; OECD, 2019[3]).
Box 2.2. End-of-pipe treatment for removal of pharmaceuticals and its limitations
The degree of pharmaceutical removal in WWTP highly varies depending on the physico-chemical properties of the APIs and the treatment process. Advanced wastewater treatment processes, such as adsorption (powered or granular) via activated carbon, ozonation, filtration by nanofiltration or reverse osmosis membranes, have been demonstrated to effectively remove most pharmaceuticals. These can achieve higher removal rates for pharmaceuticals in comparison to conventional secondary wastewater treatment (activated sludge processes, or other forms of biological treatment such as biofiltration).
However, advanced treatment technologies are generally more cost-intensive than traditional technologies and increase treatment costs by a factor of two to four, depending on technology and WWTP size (Bui et al., 2016[25]). Consequently, these technologies are not so commonly used for public WWTPs, though some countries have decided to upgrade some of their facilities. For instance, Switzerland implemented advanced wastewater treatment on a large scale using ozonation and granulated activated carbon technologies.
Decentralised point-source effluent management from hospitals, healthcare facilities, elderly homes and pharmaceutical manufacturing sites may be another route for end-of-pipe treatment. The high concentration of medicines, contrast media, cytostatics, antimicrobial resistant bacteria and pathogens1 in hospital discharges may provide a case for emission capture and treatment at source. Currently, legislation rarely holds hospitals accountable for non-clinical wastewater discharges and excreted pharmaceuticals. Nonetheless, several newly built hospitals, for instance in the Netherlands, installed on-site treatment facilities on voluntary basis (Dutch Waste Sector, 2018[26]). Trials and pilot projects are also underway in Germany, Ireland and Switzerland (EurEau, 2019[27]).
Waste streams from pharmaceutical production facilities form another possible point-source of API emissions.2 In most OECD countries, this source is under regulatory scrutiny and proper end-of-pipe treatment facilities are in place. However, in less developed countries, where appropriate regulatory frameworks are less defined or insufficiently enforced, this may pose a serious source of contamination that requires policy attention.
1. Many of the antimicrobial resistant bacteria emerge in hospitals and their presence in hospital effluents is thus disproportionate (Rizzo et al., 2013[153]; Hocquet, Muller and Bertrand, 2016[154]).
2. In some countries, studies have identified high concentrations of APIs in the discharge vicinities of pharmaceutical production facilities (Lübbert et al., 2017[155]; Larsson, 2014[156]).
Some pharmaceuticals contained in wastewater are likely to be filtered out by WWTPs and collected in the sewage sludge. Where sewage sludge is applied on land for agricultural use (“landspreading”) or used in compost, pharmaceutical residues may still enter environmental systems. Landspreading and composting are common practice in most OECD countries (Figure 2.2).
Figure 2.2. Method of sewage sludge disposal in selected OECD countries
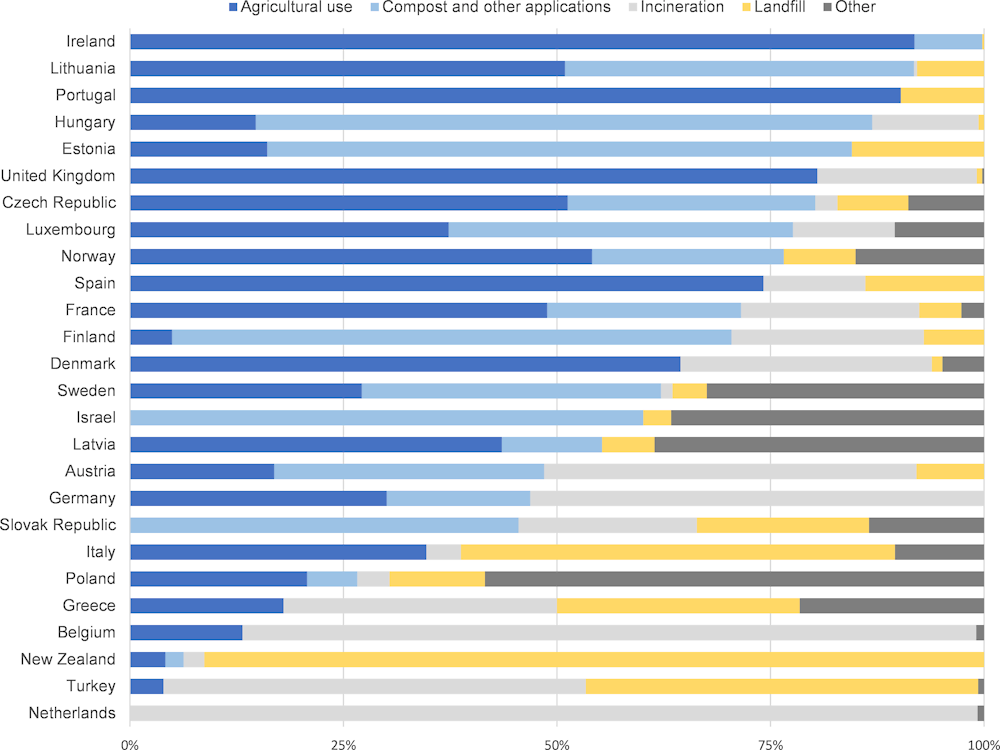
Note: “Other” category for Latvia contains biogas production and temporary storage for future use.
Source: Eurostat (2019 or nearest year) and (OECD, 2019[28]).
Combined sewer systems that collect rainwater runoff, domestic sewage and industrial wastewater in the same pipe pose a particular threat. These systems are designed to overflow occasionally and discharge excess wastewater directly into nearby streams or other water bodies. In the event of strong rainfall, combined sewer overflows lead to direct discharge of untreated human and industrial wastewater in water bodies. Additionally, in areas where households are not connected to a sewage system, pharmaceutical residues can enter the environment through discharge of wastewater from septic tanks.
2.2.2. Landfilled municipal solid waste
In OECD countries, landfill rates vary from around 90% in some countries to close to 0% in others (Figure 2.3). In occasions where mixed MSW is landfilled, pharmaceutical residues risk leaching into environmental systems if leachate is not collected and treated properly. Several studies found residues of pharmaceuticals and their metabolites in landfill leachate across the United States (Masoner et al., 2014[18]; Masoner et al., 2015[29]; Clarke et al., 2015[30]), Shanghai (Sui et al., 2017[31]) and Taiwan (Lu et al., 2016[32]). Collected landfill leachate is commonly discharged to WWTPs for treatment, where it was also proven to contribute to the loading of wastewater influents (Masoner et al., 2020[33]).
Proper management of landfill leachate is critical to avoid dispersion of pharmaceutical residues. Globally, dumpsites without proper infrastructure to manage leachate remain an important mode of MSW final disposal. Clearly, such practices create a significant risk of pharmaceutical residues entering environmental systems (World Bank, 2018[34]).
Figure 2.3. Municipal waste fate in OECD countries
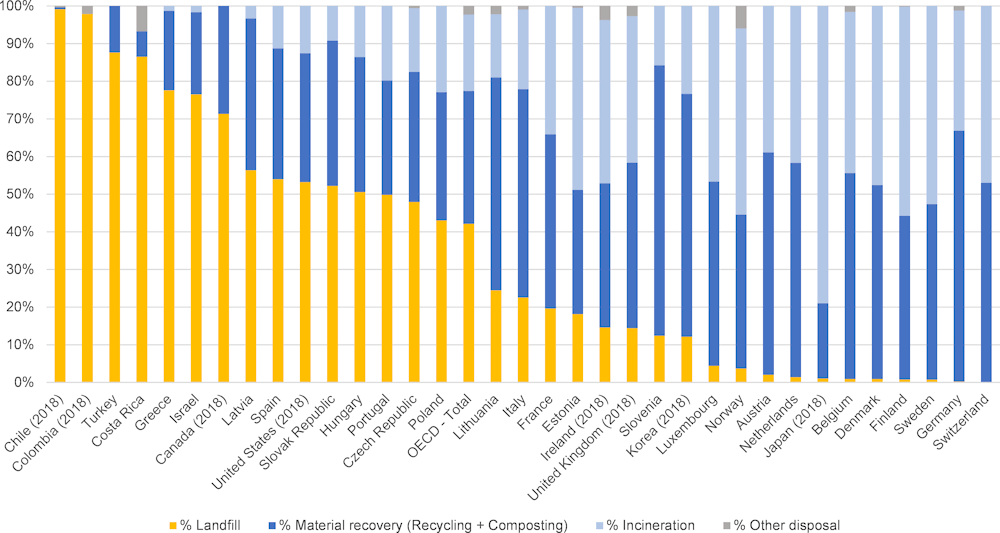
Note: Data for 2019 or nearest year.
Source: OECD Statistics.
2.3. Effects of pharmaceuticals in the environment
The vast majority of pharmaceuticals have not yet been evaluated for their long-term toxicity, occurrence or fate in the environment. Nevertheless, certain pharmaceuticals have been proven to cause undesired adverse effects on ecosystems, including increased mortality in aquatic species and changes to physiology, behaviour or reproduction3 (Table 2.1). For example, antidepressants have been shown to alter fish behaviour; and contraceptive endocrine disrupting pharmaceuticals can interfere with fish reproduction (BIO Intelligence Service, 2013[7]; Santos et al., 2010[35]; Nash et al., 2004[6]). The German Environment Agency (UBA) estimates that 10% of the pharmaceutical products pose a potential environmental risk. Of greatest concern are hormones, antibiotics, analgesics, antidepressants and anticancer pharmaceuticals used for human health. Similar concerns apply for hormones, antibiotics and parasiticides used for veterinary pharmaceuticals (Küster and Adler, 2014[36]). The overuse and discharge of antibiotics to water bodies can also lead to mutations in animals and the development of antimicrobial resistant bacteria, posing severe risks to global health, livelihoods and food security.
Table 2.1. Examples of measured effects of certain pharmaceutical residues on aquatic organisms in laboratory studies
Therapeutic group |
Examples of pharmaceutical |
Impact and effected organisms |
Examples of studies |
---|---|---|---|
Analgesics |
Diclofenac, Ibuprofen |
Organ damage, reduced hatching success (fish) Genotoxicity, neurotoxicity and oxidative stress (mollusc) Disruption with hormones (frog) |
(Näslund et al., 2017[37]) (Mathias et al., 2018[38]) (Xia, Zheng and Zhou, 2017[39]) |
Antibiotics |
- |
Reduced growth (environmental bacteria, algae and aquatic plants) |
(Roose-Amsaleg and Laverman, 2016[42]) (Guo, Boxall and Selby, 2015[43]) (Brain et al., 2008[44]) |
Anti-cancer |
Cyclophosphamide1, mitomycin C, fluorouracil, cisplatin, doxorubicin |
Ecotoxicity, genotoxicity |
|
Antidiabetics |
Metformin |
Potential endocrine-disrupting effects (fish) |
|
Anti- convulsants |
Carbamazepine, phenytoin, valproic acid |
Reproduction toxicity (invertebrates), development delay (fish) |
|
Antifungals |
Ketoconazole, clotrimazole triclosan |
Reduced growth (algae, fish), reduced algae community growth, disruption of hormones (rats) |
|
Antihistamines |
Hydroxyzine, fexofenadine, diphenhydramine |
Behaviour changes, growth and feeding rate (fish) Behaviour changes and reproduction toxicity (invertebrates) |
|
Antiparasitics |
Ivermectin |
Growth and reduced reproduction (invertebrates) |
|
Beta blockers |
Propranolol |
Reproduction behaviour (fish), reproduction toxicity (invertebrates) |
|
Endocrine active pharmaceuticals |
E2, EE2, levonorgestrel |
Disruption with hormones causing reproduction toxicity (fish, frogs) |
(Kidd et al., 2007[63]) (Kvarnryd et al., 2011[64]) (Gyllenhammar et al., 2009[65]) (Armstrong et al., 2016[66]) |
Psychiatric dugs |
Fluoxetine, sertraline, oxazepam, citalopram, chlorpromazine |
Behaviour changes - feeding, boldness, activity, sociality (fish) Disruption with hormones (fish) Behaviour changes - swimming and cryptic (invertebrates) Reproduction toxicity and disruption with hormones (invertebrates) |
(Brodin et al., 2014[69]; Brodin et al., 2013[5]) (Kellner et al., 2016[70]) (De Castro-Català et al., 2017[72]) (Di Poi et al., 2014[73]) |
Note: 1) Transformation of Cyclophosphamide and Ifosfamide. 2) NB, Caldwell et al. (2019[76]) conclude the opposite; that metformin and its transformation product guanylurea indicate no significant environmental risk.
Source: Adapted from (OECD, 2019[3]).
Notes
← 1. Nevertheless, hospital effluents may play an important role in the introduction of pathogens into public wastewaters, especially concerning multi-resistant bacteria. To date, most hospitals are not specifically equipped with wastewater treatment infrastructure to immediately treat their highly concentrated effluent after discharge.
← 2. Note that this report focuses on discharge from human pharmaceuticals. However, pharmaceuticals for veterinary use, aquaculture and agriculture form an important source of pharmaceutical residues in the environment and are included here for completeness.
← 3. See (OECD, 2019[3]) for a more detailed discussion of proven and potential environmental and health effects of pharmaceuticals.