This chapter analyses current policies and outlines policy reform avenues towards mitigating greenhouse gas (GHG) emissions in agriculture. It first presents agriculture’s contribution to climate change, both through direct emissions linked to on-farm production, and indirectly through land use change. The chapter then outlines opportunities for agriculture to contribute to climate change mitigation, either by reducing its emissions or through carbon sequestration within agricultural biomass and soils. A comprehensive overview of what the 54 countries covered in OECD’s Agricultural Policy Monitoring and Evaluation 2022 are doing to contribute to climate change mitigation highlights strategies and mitigation targets, and specific measures implemented. The final section discusses the impacts of current agricultural support policies on GHG emissions. The chapter concludes with key recommendations for reforming agricultural policies to address climate change mitigation objectives.
Agricultural Policy Monitoring and Evaluation 2022
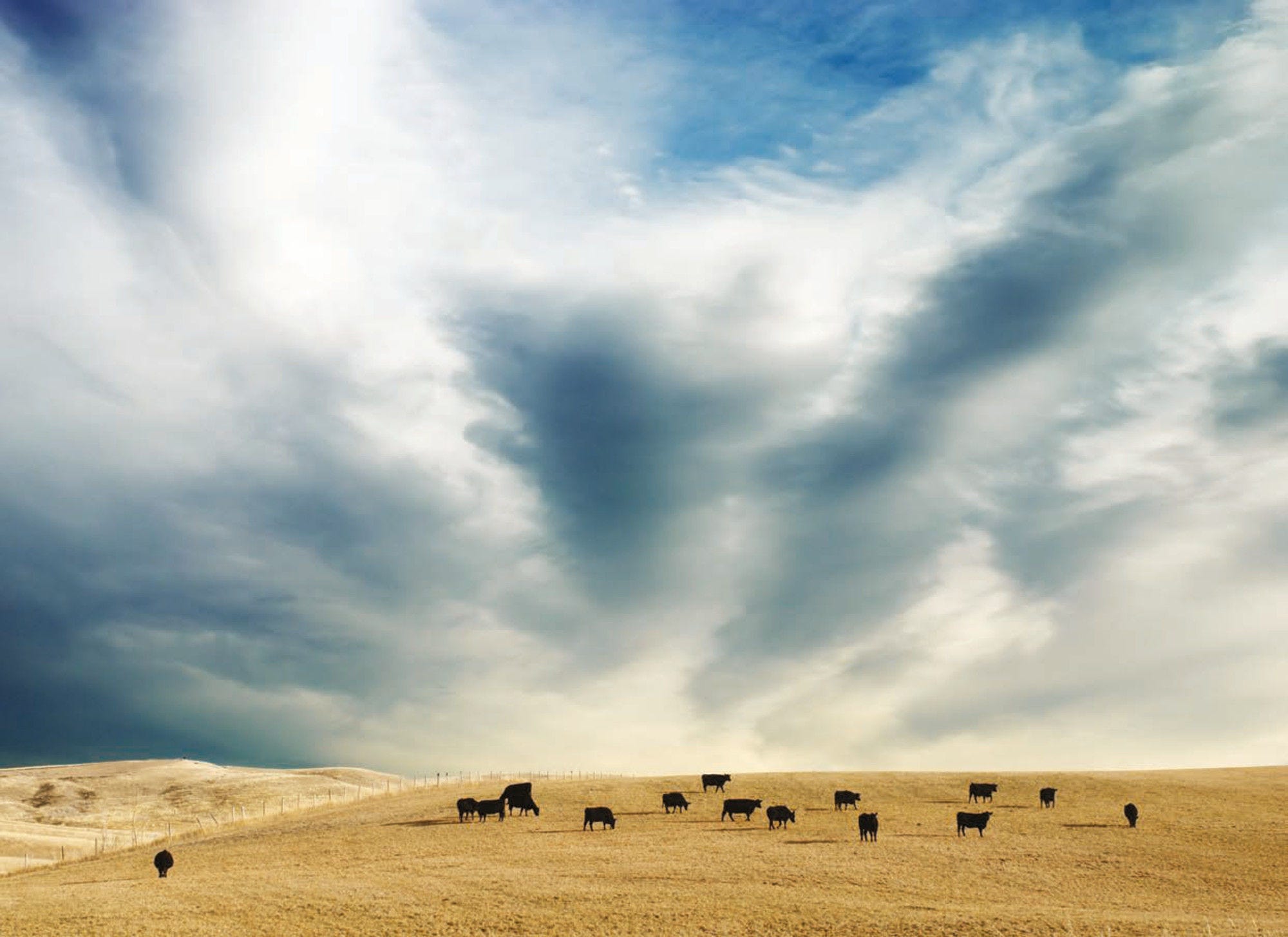
1. Reforming agricultural policies for climate change mitigation
Copy link to 1. Reforming agricultural policies for climate change mitigationAbstract
Agriculture faces a complex and unique challenge in the context of climate change. First, agriculture is particularly vulnerable to climate change, due to its dependency on weather and climatic conditions. It is already experiencing negative impacts from climate change from higher temperatures, increased variability of rainfall, invasive pests, and the greater frequency of extreme weather events. Around the world, building the resilience of the sector and ensuring adaptation to climate change is a significant challenge, particularly in the poorest countries in which agriculture both plays an important role for the economy and basic subsistence needs, but where climate change impacts are expected to hit the hardest.
Second, agriculture is itself a major source of global greenhouse gas (GHG) emissions, both directly, through on-farm emissions linked to production, and indirectly, through land use change due to agricultural expansion. In the absence of action, emissions from agriculture are projected to continue to rise and the sector’s share of total emissions to increase as efforts to decarbonise other sectors accelerate. That said, there are ample opportunities for agriculture to contribute to global efforts to mitigate climate change, by reducing both direct and indirect emissions.
Third, unlike many other emissions-intensive sectors, agriculture also has the potential to contribute positively to reducing emissions by removing carbon from the atmosphere, through efforts to sequester carbon in biomass and soils. This can be achieved through practices that also raise productivity, such as conservation agriculture and the restoration of degraded agricultural lands, both to mitigate direct emissions and prevent further indirect emissions from land use change.
There are also particular challenges to tackling GHG emissions in agriculture. The sector is subject to a wide range of government policies, including significant support policies in OECD countries. A key question is thus the extent to which existing policies help or hinder efforts to adapt to, or mitigate climate change in agriculture. Equally as important is examining the types of mitigation policies that governments have adopted, or are considering, to combat agricultural emissions. With the strategic importance of food security set to increase, as population growth and rising incomes continue to boost extra demand for food, agriculture lies at the heart of the triple challenge facing food systems: providing adequate, affordable, safe and nutritious food for a growing global population; providing livelihoods all along the food value chain; and doing so while increasing the sustainability of the sector and its contribution to combatting climate change.
Against this background, this chapter discusses the contribution of agriculture and current agricultural policies to climate change and how policy changes can help the sector to become a greater part of the solution to reducing global GHG emissions. While resilience and adaptation are key issues, they have already been the subject of extensive work (see Box 1.1) and are not covered further here. Furthermore, while GHG emissions from other pre- and post-production segments of food systems have witnessed substantial increases in recent decades, this chapter focuses on the contribution of agriculture and closely related issues where agricultural policymakers may be more directly involved (such as food loss and waste and consumer demand). The chapter begins with an overview of agriculture’s contribution to climate change; it then discusses the opportunities for the sector to contribute to emission reductions. The chapter then looks ahead to country targets under the Paris Agreement and policy actions taken by countries to mitigate agricultural emissions. It concludes by discussing the extent to which current agricultural support policies may help or hinder global efforts to mitigate GHG emissions, and offers a way forward with recommendations for countries to reform their agricultural policies to address climate change mitigation objectives.
Box 1.1. Adaptation to climate change and resilience of the agricultural sector
Copy link to Box 1.1. Adaptation to climate change and resilience of the agricultural sectorAccording to the Intergovernmental Panel on Climate Change (IPCC), global temperatures have already increased by 1.1°C on average over the past decade, compared to preindustrial levels (IPCC, 2022[1]). As a consequence, human activities are already exposed to changing climatic patterns, highlighting the urgent need for effective mitigation action. While agriculture has strong potential to contribute to GHG emissions reduction and carbon sequestration efforts, it is also among the most exposed sectors to changing weather patterns and natural disasters. Low income countries are particularly vulnerable, as a large part of their population still depends on agricultural activities for their livelihoods and rural poverty is at the core of many development challenges.
For these reasons, climate change mitigation needs to be complemented by adaptation efforts, and agriculture needs to find new and innovative pathways combining these two transformation agendas. Adaptation strategies can rely on both the adoption of new management practices (e.g. crop diversification, improved water management) that are better suited to the changed environmental conditions, and new technologies (e.g. flood or heat-resistant crop varieties) supported by R&D investments (Ignaciuk and Mason-D’Croz, 2014[2]).
In the face of increasingly unpredictable climatic events, agriculture also needs to strengthen its resilience – defined as its ability to prepare and plan for, absorb, recover from, and more successfully adapt and transform in the face of shocks – by revising its approach to risk (OECD, 2020[3]). In particular, risk management should move from focusing on individual agents to a more systemic perspective, and involve different decision levels. Policies should be put in place to not only help farmers to recover from various shocks, but also to build capacities to adapt in response to new risks, and to transform in order to eliminate these risks to the best possible extent.
OECD work has emphasised five dimensions that should be considered by public and private actors when designing their risk management strategies in agriculture (OECD, 2020[3]). These should pay attention to: 1) the time-frame, taking early ex-ante actions and targeting for the long-term; 2) possible trade-offs between policy objectives and actor interests, comparing outcomes of alternative options; 3) participatory collaborative processes involving multiple stakeholders; 4) investments in on-farm resilience capacity, based on strengthening human capital and supporting the uptake of adapted technologies and practices; 5) no regret policies, taking into account of possible future scenarios on climate change and other economic and environmental conditions.
Country case studies underscore how this framework for agricultural resilience can help address natural disasters faced by the agricultural sector (OECD/FAO, 2021[4]). Key elements include setting the right incentives at policy level to trigger action at the farm level; providing the data to help farmers choose the right strategic investments on the farm; and engaging with trusted stakeholders to help ensure the effective implementation of policy actions by farmers.
Measuring agriculture’s contribution to climate change
Copy link to Measuring agriculture’s contribution to climate changeDirect and indirect emissions
Agriculture is a major driver of climate change via two main channels:
Emissions from the sector itself, linked to production. In particular, the agricultural sector is a major source of non-CO2 emissions, notably methane (CH4) and nitrous oxide (N2O) directly emitted from crop and livestock production.1
Emissions related to land use, land use change and forestry (LULUCF).2 The main sources of LULUCF emissions related to agriculture include net forest conversion to cropland and pasture, conversion of native grasslands to cropland, tropical forest fires, peat fires, soil organic carbon changes and drained organic soils (Figure 1.1).
Together, both these elements – agriculture and LULUCF – are referred to as agriculture, forestry, and other land use (AFOLU). Over the period from 2010 to 2019, average annual net GHG emissions from AFOLU represented around 21% of total global anthropogenic GHG emissions, and that share increased to 22% by 2019.3 Of this, emissions from LULUCF accounted for around 11% of global GHG emissions,4 while on-farm emissions linked to agricultural production accounted for a further 11%.5 In other words, AFOLU represents roughly one-fifth of anthropogenic GHG emissions, half of which comes from CO2 LULUCF emissions and the other half from CH4 and N2O direct emissions from agricultural production.
Figure 1.1. Global net anthropogenic emissions from agriculture, forestry and other land use (AFOLU) and other sectors, total and decomposition by gas, annual average for 2010-19
Copy link to Figure 1.1. Global net anthropogenic emissions from agriculture, forestry and other land use (AFOLU) and other sectors, total and decomposition by gas, annual average for 2010-19
Note: LULUCF: Land use, land use change and forestry. Data labels indicate the percentage share of Agriculture, LULUCF (CO2) and Other sectors in the total emissions from each individual GHG, as well as total global net anthropogenic GHG emissions. Other sectors correspond to buildings, transport, industry, other energy, and also include fossil fuel emissions at farm level, consistent with IPCC nomenclature. Other gases include fluorinated gases such as chlorofluorocarbons (CFCs), hydrofluorocarbons (HFCs), hydrochlorofluorocarbons (HCFCs), perfluorocarbons (PFCs) and sulphur hexafluoride (SF6). All values expressed in units of CO2eq are based on IPCC AR6 100-year Global Warming Potential (GWP100) values with climate-carbon feedbacks (CH4 = 27.0; N2O = 273). LULUCF only shows CO2 emissions, as reported in IPCC (2022[5]). Non-CO2 LULUCF emissions (not shown) represent an additional 0.6 GtCO2eq, due to emissions from vegetation and peatland burnings.
Source: Compiled from IPCC (2022[5]) and EDGAR (Minx et al., 2021[6]).
Direct on-farm emissions from agriculture contribute much more to non-CO2 gases than other sectors: they generate 42% of global anthropogenic methane emissions and 70% of global nitrous oxide emissions.6 These gases have a much higher impact on global warming than CO2, and the 100-year global warming potential (GWP100)7 of methane has been regularly revised upward over the past two decades by the IPCC (from 21 in 1995 to 27 currently for agriculture). Furthermore, methane is a short-lived gas, which means its climate impact is much stronger over a shorter time-frame, whereas its effect becomes negligible faster when compared with carbon dioxide. That is, the GWP for non-fossil methane increases from 27 over a 100-year period to close to 80 over a time horizon of 20 years. This means that methane emissions are set to have an effect on global temperatures by mid-century about three times larger than indicated by the usual calculations which take a 100-year perspective.
Indirect emissions from agriculture are mainly CO2 emissions, particularly from the clearing of forests and other natural vegetation and the drainage of wetlands and peatlands for agricultural purposes. Land clearing removes carbon stored in above-ground biomass, while organic soil drainage leads to the oxidation of soil carbon, and this important below-ground carbon sink continues to emit for many years following the land conversion. The burning of biomass on agricultural and forest land and the combustion of organic soils (peatland fires) also contribute to GHG emissions from forestry and other land use.
On the other hand, agriculture can also contribute to GHG removals, through carbon sequestration in agricultural plantations, and in cropland and grassland soils, as well as partially rewetted peatlands (Henderson et al., 2022[7]). Overall, the capacity of land to act as a natural sink of CO2 will be affected by both climate change and by future agricultural activities (IPCC, 2022[5]).
Global emissions of carbon dioxide from AFOLU have remained relatively constant over the past few decades. In contrast, non-CO2 emissions from AFOLU increased by 15% between 1990 and 2019. This was primarily driven by direct agricultural emissions, which represented 91% of AFOLU’s non-CO2 emissions on average over the period.8
Direct GHG emissions from agriculture vary across countries due to differences in factors such as agricultural land area, size of the agricultural sector, mix of commodities produced, and the structure of agricultural production. Total agricultural GHG emissions across all 54 countries covered in this report contribute about two-thirds of total global agricultural GHG emissions.9 The five largest emitters are India, the People’s Republic of China (hereafter “China”), the United States, Brazil and the EU-27, collectively accounting for 72% of the total across all 54 countries. Conversely, the five smallest emitters (Iceland, Israel, Costa Rica, Norway and Switzerland) represent just 0.4% of total agricultural GHG emissions (see Figure 1.2 for direct agricultural GHG emissions in 2019 from these 54 countries). To allow the relevant compositional breakdown to be legible, this chart is presented in total, and then as three separate charts covering groups of countries according to the size of their agricultural emissions: low, medium and high.
Enteric fermentation and manure management from livestock account for more than 50% of direct emissions from agriculture across all 54 countries. Enteric fermentation, a digestive process of cattle, sheep, goats and other ruminant livestock which generates methane, accounts for the vast majority of these emissions (42% of direct agricultural emissions). Manure management contributes 8%, both from methane and nitrous oxide emissions. The share of these livestock emissions in total agricultural emissions varies across the 54 countries covered in this report, ranging from 19% in the Philippines to 78% in Australia and New Zealand. Livestock is also responsible for additional emissions due to manure deposition on grassland and manure applications to croplands (see further below on agricultural soils). When manure left on pasture is added to the sources above, livestock accounts in total for two-thirds of agricultural emissions over the 54 countries covered.10
Rice cultivation is also a significant source of methane emissions, and is responsible for 11% of direct agricultural emissions across the 54 countries. These emissions are concentrated in Asia, with five countries (China, India, Indonesia, the Philippines and Viet Nam) collectively accounting for 67% of global rice production and 91% of total emissions from rice cultivation across the 54 countries (OECD/FAO, 2021[8]). On average across the OECD, rice cultivation only represents 2% of direct emissions from agriculture.
Agricultural soils are the principal driver of nitrous oxide emissions, due to the application of synthetic nitrogen or organic fertiliser, crop residues, as well as manure and urine deposited on grassland by ruminant livestock. These emissions account for 37% of direct agricultural emissions across all 54 countries, but with high variation across countries: agricultural soils account for 76% of direct agricultural emissions in Ukraine, and more than half of total agricultural emissions in Israel and the United States.
Other sources of direct agricultural emissions come from more marginal sources across all 54 countries, and represent only 2% of total agricultural emissions. These include carbon dioxide from liming, urea application and other carbon-containing fertilisers as well as methane and nitrous oxide from prescribed burning of savannahs and field burning of agricultural residues. In addition, some on-farm emission sources are not accounted for as direct agricultural emissions under the UNFCCC inventory typology, but can be non-marginal: they cover in particular energy consumption on the farm, such as fuel for agricultural machinery, other energy sources used for barns and glasshouses, as well as irrigation. Box 1.2 provides more detail on these sources, including accounting of GHG emissions through a food systems lens.
Figure 1.2. Direct GHG emissions from agriculture, by country and source, 2019
Copy link to Figure 1.2. Direct GHG emissions from agriculture, by country and source, 2019
Note: Data from 2019, except for Chile, Israel, Korea (2018); Mexico (2015); Colombia and Argentina (2014). CO2: Carbon Dioxide, CH4: Methane, N2O: Nitrous oxide.
Source: (OECD.Stat, 2021[9]).
Box 1.2. How do food systems contribute to global GHG emissions?
Copy link to Box 1.2. How do food systems contribute to global GHG emissions?In addition to the emissions generated by agricultural production, land use and land use change, food systems contribute to GHG emissions through various pre- and post-production processes such as fertiliser manufacturing, food processing, packaging, transport, retail, household consumption and food waste disposal. However, there are major knowledge gaps and large uncertainties regarding the quantification of food systems emissions. Estimates from (IPCC, 2022[10]) based on (Crippa et al., 2021[11]) and (FAO, 2021[12]) indicate that food systems emitted 16.8 GtCO2eq per year in 2018 (95% confidence range: 13-23 GtCO2eq per year), equivalent to 31% (range 23-42%) of total anthropogenic GHG emissions.11 This represents an increase of 16% over 1990 levels, primarily driven by non-AFOLU emissions which have grown to represent 39% of food systems emissions in 2018 (compared with 28% in 1990) (Figure 1.3).
Figure 1.3. GHG emissions from global food systems by sector and gas, 1990 and 2018
Copy link to Figure 1.3. GHG emissions from global food systems by sector and gas, 1990 and 2018
Note: Solv+Product Use: Solvent and Other Product Use. LULUCF: Land Use, Land-Use Change and Forestry. Data labels indicate the percentage share of each individual sector/gas in total global net anthropogenic GHG emissions.
Source: (IPCC, 2022[10]).
Emissions from energy use occur throughout the food supply chain and consist almost entirely of CO2 emissions. In 2018, two-thirds of energy emissions came from energy industries supplying electricity and heat, manufacturing and construction, and transport. Refrigeration is an important source of energy use in the retail sector, and leads to substantial increases in fuel consumption during distribution. Refrigeration in supermarkets is energy intensive and also contributes to leakages of fluorinated gases (F-gases). Transport represents just 5-6% of food systems emissions and is dominated by road transport (92% of food systems transport emissions), followed by marine shipping (4%), rail (3%) and aviation (1%).
Emissions from industrial processes in food systems consist of emissions from refrigerants (F-gases) and the fertiliser industry (CO2 from ammonia production and N2O from nitric acid). Emissions from F-gases can have disproportionately large effects on global temperatures even at small atmospheric concentrations. Although F-gases contributed only 3% of global food systems emissions in 2018, this share is projected to increase rapidly due to growth in cold chains and refrigerated storage capacity in developing countries.
Waste accounts for 10% of food systems emissions, and includes domestic and commercial wastewater (55% of food systems waste emissions), solid waste management (36%), industrial wastewater (8%) and waste incineration and other waste management systems (1%). Food waste decay also generates significant quantities of methane, through the decomposition of organic materials in landfills.
Source: (IPCC, 2022[10]).
Emissions intensity of the agricultural sector
To account for the vast differences in the size of countries’ agricultural sectors, emissions can also be expressed relative to agricultural output, or relative to a factor of production, such as agricultural land. Measuring agricultural emissions per USD of production value reveals the emissions intensity of agricultural output (Figure 1.4). Countries with a strong share of ruminant products in agricultural production (e.g. Brazil, Argentina, New Zealand, Mexico) or low domestic market prices (e.g. Kazakhstan, Philippines) rank the highest. On the other hand, countries with high value of production (e.g. Japan, Korea, Switzerland) and/or low share of ruminant products (e.g. China) tend to show a low emission intensity of agriculture. Overall, the emissions intensity of agricultural output in the OECD is slightly higher than in the 11 emerging economies covered in this report.12
When looking at the emissions intensity of agricultural land, measured as agricultural emissions relative to total agricultural land area, countries with large territories such as Australia, Kazakhstan and South Africa tend to have the lowest agricultural emissions per hectare. Agricultural emissions per hectare tend to be higher in countries with a relatively small agricultural area, and where emissions-intensive commodities (e.g. rice cultivation in Korea, Japan, Viet Nam and the Philippines; or livestock production in Norway, Switzerland and New Zealand) represent an important share of agricultural production. In addition to geographical characteristics, differences across countries can also be explained by variations in production systems and the extent to which land contributes to output relative to other factors of production. By this metric, agricultural emissions per hectare in the OECD are lower than the average for the 11 emerging economies.13However, it is important to note that this indicator does not capture indirect agricultural emissions (i.e. those relating to changes in land use), which are substantial in a number of countries. High land emissions intensity, when driven by production intensification, may also result in GHG emissions offsets associated with reduced land use expansion.
Figure 1.4. Emissions intensity of agricultural output and land across countries
Copy link to Figure 1.4. Emissions intensity of agricultural output and land across countries
Notes: Data is from 2019, except for Canada, Chile, Israel, Korea (2018); Mexico (2015); Argentina and Colombia (2014). The emissions intensity of agricultural output is calculated as the ratio of direct GHG emissions from agriculture to the value of agricultural production. Agricultural GHG emissions per hectare is calculated as the ratio of direct GHG emissions from agriculture to total agricultural land area.
The OECD total does not include the non-OECD EU Member States.
The 11 emerging economies include Argentina, Brazil, China, India, Indonesia, Kazakhstan, the Philippines, Russian Federation, South Africa, Ukraine and Viet Nam.
Source: OECD.Stat (2021), Agri-environmental indicators, https://stats.oecd.org/# ; OECD (2022), “Producer and Consumer Support Estimates”, OECD Agriculture statistics (database), http://dx.doi.org/10.1787/agr-pcse-data-en.
GHG emissions intensities also vary significantly when comparing across food commodities. Poore and Nemecek (2018[13]) provide estimates of GHG emissions intensities, expressed in kg CO2eq per kg of product and per nutritional functional unit (e.g. 100g of protein) for more than 40 commodities (Figure 1.5).14 Emissions intensities are measured using attributional Life Cycle Assessment considering the full supply chain.
On average, emissions intensities are highest for ruminant meat, notably beef from beef herds, and lamb.15 Emissions from dairy systems are shared between milk and meat production, resulting in a lower carbon footprint for beef from dairy cattle.16 Emissions intensities are significantly lower for plant-based food products. While rice is more emissions intensive, most other grains generate relatively low emissions per unit of product, and most fruits, vegetables, roots and tubers have even lower average emissions.17
GHG emission intensities for individual products also vary considerably when considering the full heterogeneity of farms, depending on where and how the relevant product is produced (Figure 1.5). For example, the highest emissions from beef producers are more than five times greater than those from the lowest emitters in the case of beef herds, and nearly twelve times those from the least emitting dairy herd producers.18 This wide variation reflects differences in production systems, which vary in terms of productivity, diet composition, diet quality, and feed use efficiency. Livestock in grazing systems mostly consume grass and tend to have higher emission intensities than mixed crop-livestock systems, where animal feed rations can be more easily optimised. Average emissions intensities are particularly high in grazing systems that lead to the expansion of pasture and hence to additional emissions from land-use change, as well as systems characterised by low feed digestibility, poor animal husbandry and lower slaughter weights (Herrero et al., 2013[14]; Gerber et al., 2013[15]).
Within major staple crops such as wheat and maize, the highest emissions per kg are three times greater than those from the lowest emitters. Rice is the most emissions-intensive staple crop, as the production of rice in flooded paddies blocks oxygen from penetrating the soil, facilitating the growth of methane-producing bacteria (Adhya et al., 2014[16]). However, the range of emissions among rice farms can vary considerably, with the highest emissions from rice farms reaching levels six times greater than those of the lowest emitters.
Figure 1.5. GHG emissions intensity of food commodities
Copy link to Figure 1.5. GHG emissions intensity of food commoditiesMean, 10th and 90th percentile emissions intensities (per kg of food product and per 100g of protein)

Note: Aggregation of CO2, CH4, and N2O emissions in (Poore and Nemecek, 2018[13]) updated to use IPCC-AR6 100-year GWP. Data for capture fish, crustaceans, and cephalopods from (Parker et al., 2018[17]), with post-farm data from (Poore and Nemecek, 2018[13]), where the ranges represent differences across species groups. CH4 emissions include emissions from manure management, enteric fermentation, and flooded rice only.
*Grains are not generally classed as protein-rich, but they provide ~41% of global protein intake. Here grains are a weighted average of wheat, maize, oats, and rice by global protein intake (FAO Food Balance Sheets).
**Conversion of annual to perennial crops can lead to carbon sequestration in woody biomass and soil, shown as negative emissions intensity.
Looking ahead
With the global population projected to reach 9.7 billion in 2050, agricultural emissions are expected to continue to rise in the coming years. Projections from the OECD/FAO Agricultural Outlook 2022-2031 forecast that direct emissions from agriculture should grow by 6% between 2019-21 and 2031 (assuming no changes in current policies and on-trend technological progress). Livestock would account for more than 85% of the global increase, and agricultural emissions are expected to rise in nearly all regions over the next decade (except for Europe and Central Asia) (Figure 1.6). Emissions growth should be highest in middle and low-income regions, which are characterised by faster output growth and more emissions-intensive production systems. Most of the increase is to occur in Sub-Saharan Africa, where direct GHG emissions from agriculture are projected to grow by 17% over that period.
That said, in all regions, agricultural production growth is expected to exceed the growth in direct GHG emissions from agriculture, resulting in a partial decoupling of emissions from production and a decline in the carbon intensity of agricultural production over the next decade. The decoupling of emissions from production represents the continuation of a longstanding trend over the past few decades, and will primarily be driven by yield improvements and a decline in the share of ruminant livestock in total agricultural production (OECD/FAO, 2022[18]).
Note: This figure shows projected annual growth in direct GHG emissions from agriculture together with annual growth in the estimated net value of production of crop and livestock commodities covered in the Outlook (measured in constant USD 2014-16 prices). Estimates are based on historical time series from the FAOSTAT Emissions Agriculture databases which are extended with the Outlook database.
Source: (OECD/FAO, 2022[19]).
While longer-term projections vary considerably, most studies predict a continuation of the increase in agricultural GHG emissions by mid-century in the absence of sustained efforts to mitigate these emissions. Under a business-as-usual scenario, FAO (2018[20]) forecasts a 50% expansion in global agricultural output between 2012 and 2050, as well as an 18% increase in harvested areas, a 46% increase in total animal herd size, a 50% growth in nitrogen fertiliser consumption, and a 20% increase in global agricultural GHG emissions. Increases in agricultural emissions could easily exceed 50% over the same time period if less favourable conditions are considered (OECD, 2016[21]; Popp et al., 2017[22]; Springmann et al., 2018[23]).
Opportunities for agriculture to contribute to climate change mitigation
Copy link to Opportunities for agriculture to contribute to climate change mitigationAs a major source of global GHG emissions, agriculture has an important role to play in helping to meet the world’s climate change mitigation objectives. Indeed, the Paris Agreement targets will remain out of reach if mitigation efforts do not include the agriculture and food sectors (Clark et al., 2020[24]). The latest assessment report from the IPCC finds that rapid deployment of mitigation measures in AFOLU will make an essential contribution to all potential pathways to limit the increase in global temperatures to 1.5°C above pre-industrial levels (IPCC, 2022[5]). Based on integrated assessment modelling and technical bottom-up studies, it is estimated that AFOLU as a whole could contribute 20-30% of global mitigation efforts for a 1.5°C or 2°C pathway by 2050, at a relatively modest cost (IPCC, 2022[5]). The largest share of this reduction would come from the protection and restoration of forests and other natural ecosystems, with a smaller but still important contribution coming from agriculture alone (OECD, 2019[25]).
There are two major areas of opportunity for the agricultural sector to mitigate GHG emissions:
Supply side options. These involve different areas of intervention aimed at various stages of the production process:
Reducing direct on-farm emissions from agricultural production: increasing productivity and efficiency in input use through better technology and management, as well as specific technical options reducing agricultural emissions.
Reducing indirect emissions from land use change and increasing carbon stocks in agricultural soils: reducing the expansion of agricultural land, including through advances in land productivity, restoring degraded lands and rewetting drained peatland, increasing soil carbon sequestration on cropland and grassland, and afforestation.
Reducing emissions from food losses: limiting losses in the field, and post-harvest losses on the farm.
Demand side options. These options correspond to changes in the demand for agricultural products, at the consumer level, due to changes in dietary preferences, purchase of food with lower embedded emission and reductions of food waste, all leading to lower emission footprint at consumer level.
In addition, agriculture can contribute to some extent to global mitigation through bioenergy production. These options do not primarily aim at reducing agricultural emissions, but rather to use agricultural production to reduce emissions from fossil fuels in other sectors (see Box 1.4).
This section provides more details on these technical options, both on the supply and the demand side, followed by an overview of their mitigation potentials.
Reducing direct on-farm emissions from agricultural production
On-farm emissions mostly relate to non-CO2 emissions associated with fertiliser use, rice cultivation and, in the case of the livestock sector, enteric fermentation, manure management and manure deposition on soil. Some fossil fuels are also consumed on the farm, for the use of machinery, irrigation, heating of barns, etc., and can form part of mitigation efforts, even if they are not directly accounted as part of the AFOLU sector.
Crop cultivation emissions reduction
For many crops, the largest source of emissions is nitrous oxide related to the use of synthetic or organic fertilisers. Improved cultivation practices and more efficient usage of synthetic fertilisers and organic manure have allowed many countries to reduce their nitrous oxide emissions, while steadily expanding agricultural production. However, considerable scope remains to reduce emissions without compromising productivity and food security, as 45% of nitrogen added to fields globally is not absorbed by crops (Blandford and Hassapoyannes, 2018[26]). Advances in precision agriculture and the use of nitrification and urease inhibitors can further enhance the management of crop nutrient cycling, but should be complemented by more holistic approaches, relying on integrated crop management and improved crop rotations (e.g. with legumes and cover crops), as well as crop-livestock systems integration.
Irrigated rice is a significant source of methane emissions, generated by the area flooded for its cultivation. Bacterial methane production in rice paddies can be strongly influenced by changes in water management regimes, such as the duration of flooding intervals and frequency of flooding. Midseason drainage, a common irrigation practice in China and Japan, along with intermittent irrigation in northwest India, can result in significantly lower methane emissions (Wassmann, Hosen and Sumfleth, 2009[27]). Improvements in rice yields can also help to reduce emissions from rice cultivation, although there can be trade-offs between improved water management to reduce methane emissions, and offsetting increased emissions from fertiliser use, and yield size.
Livestock emissions reduction
As noted above, the most prominent source of GHG emissions in agriculture is from enteric fermentation of ruminant livestock, with beef having the largest emissions footprint globally by a wide margin per unit of protein produced (Blandford and Hassapoyannes, 2018[26]). On the supply side, the emissions intensity of ruminant products can be reduced through improved productivity at the animal or herd level, or via more direct interventions aimed at limiting enteric fermentation.
Increasing feed conversion efficiency in livestock production can be achieved through advances in herd genetics, improving feed and pasture quality, and strengthening farm and animal management, including through disease prevention (MacLeod et al., 2015[28]). All these options also allow for higher production and larger incomes for a given number of animal heads. Due to the large heterogeneity in livestock productivity and emission intensities around the world (Herrero et al., 2013[14]), they could be particularly useful in low and middle income countries to deliver jointly climate mitigation and improvements in food security and nutrition. For advanced economies, the use of methane inhibitors appears more promising, with new technologies being developed, such as chemically synthesised inhibitors, specific species of seaweed that could be used as feed supplements, and anti-methanogen vaccine solutions (Reisinger et al., 2021[29]). Many of these options have been intensively studied over the past decade and could soon become available commercially. That said, questions remain as to their long term efficacy and effects on animal health and productivity, their social acceptability and the required regulatory framework. Feed supplement options may also not be well placed to address emissions from extensive agricultural systems where ruminants are largely grass-fed.
Manure management also contributes significantly to livestock GHG emissions. Options to limit associated CH4 and N2O emissions include improved application methods, storage and composting, the use of nitrification inhibitors for stored manure and urine patches, alteration of animal diets and grazing practices, manure acidification and solid liquid separation, and the use of anaerobic biodigesters. Such options have already been widely deployed in some regions, with small and large-scale biodigesters producing biogas. While emissions abatement estimates for such mitigation technologies are more limited than for enteric fermentation, reduction potentials exist, in particular in developed regions and for intensive management systems (IPCC, 2022[5]).
Other on-farm emissions
On-farm energy consumption from electricity, heat and fuels is an important source of emissions. Various technologies exist to reduce these emissions, including switching to renewable sources of energy such as solar and wind power, and adopting greener and more efficient fuels to power agricultural machinery. The deployment of renewable energies on agricultural land can also provide farmers with an opportunity to earn additional income, for example with special fees for locating wind turbines on their land (the electricity production from which then contributes to the decarbonisation of other sectors). The share of emissions associated with on-farm energy consumption remains small compared to other AFOLU sources, but studies suggest these categories could be relatively cheap to abate and more easily adopted by the farming community (MacLeod et al., 2015[28]).
Reducing land use change emissions and increasing carbon stocks in agricultural soils
While productivity improvements in agriculture have reduced the need for agricultural land expansion (see Box 1.3), land use change emissions from agriculture have nonetheless been considerable, due to conversion of forests, grasslands and other carbon stocks into cropland or pastures. Agriculture currently uses approximately half of the world’s habitable land (IPCC, 2019[30]). Livestock occupies about 78% (40 million km2) of all agricultural land; this includes 35% of global crop production which is devoted to the production of animal feed (Dasgupta, 2021[31]). Empirical studies have shown that agricultural expansion is among the largest drivers of deforestation and degradation worldwide (Busch and Ferretti-Gallon, 2017[32]; Curtis et al., 2018[33]). Recent estimates suggest that large-scale commercial agriculture (i.e. cattle ranching, soy production and palm oil plantations) accounts for about 40% of tropical and sub-tropical deforestation, while local subsistence agriculture is responsible for a further 33% (Hosonuma et al., 2012[34]; FAO and UNEP, 2020[35]). Land use change is also a major cause of declining biodiversity and the depletion of soil carbon, in particular in carbon-rich peat organic soils (IPCC, 2019[30]).
Reducing deforestation induced by agricultural expansion is critical to mitigate LULUCF emissions, particularly in tropical regions where agricultural production has expanded significantly into new areas. On the production side, increasing agricultural productivity and yields is key to reducing the need for additional land to meet food demand, as well as reducing (but not eliminating) trade-offs between increased food production and negative impacts on natural ecosystems (see Box 1.3). Productivity gains can also help offset emissions through carbon sequestration, by providing the opportunity to restore and reforest marginal lands. These measures can also be accompanied by demand side options to further reduce the demand for land, as highlighted further below.
However, achieving forest protection requires additional policy interventions, such as the establishment of protected areas, effective law enforcement and forest governance, improvements in land tenure and sustainable management certification. Better forest protection is seen as a major source of emissions abatement, with the largest mitigation potential mostly located in deforestation hotspot regions, in Latin America, Africa and Southeast Asia. Agricultural activities can also lead to the conversion and degradation of other lands, such as grasslands and savannahs; however, the mitigation potential associated with protecting these lands is lower.
Among sensitive ecosystems, peatlands deserve particular attention due to the significant carbon stocks that they contain. Peatland drainage leads to large GHG emission releases through soil mineral carbon oxidation, which persist over time, and through peat fires. Globally, around 25 million hectares of drained peatlands (about 0.6% of agricultural land) are estimated to generate around 2% of total anthropogenic emissions (FAO, 2022[36]), and will continue to do so unless these are rewetted. This phenomenon is particularly acute in Southeast Asia, due to the expansion of palm and rubber plantations, which account for 80% of global peat emissions (Leifeld and Menichetti, 2018[37]; IPCC, 2022[5]). Halting and reversing peatland conversion is seen as an important emissions abatement action, at a relatively low cost (Henderson et al., 2022[7]).
Box 1.3. Total factor productivity growth contribution to climate change mitigation
Copy link to Box 1.3. Total factor productivity growth contribution to climate change mitigationSince the 1960s, the relationship between agricultural production growth and input use has evolved. Notwithstanding continued deforestation associated with expansion of agriculture in the tropics, the growing demand for food has progressively moved production away from increasing use of factors (land, labour, capital) and emission-intensive variable inputs (synthetic fertilisers, animal feed) thanks to total factor productivity (TFP) gains (Figure 1.7). TFP improvements have been the most important source of additional production since the 1990s, based on improved farm management practices, new crop varieties and breeds, and innovations related to digitisation.
Note: Each bar represents the annual average growth rate over that period. Agricultural TFP growth is estimated as the residual between output growth and input growth. The aggregate input index is calculated according to the “cost decomposition” methodology, which multiplies the growth rate of each input by their respective factor shares, revealing the extent to which each input contributes to changes in unit costs of production (Fuglie, 2015[38]). Capital includes farm machinery and livestock inventories. Variable inputs include fertilisers and animal feed (all types, except forages and silage).
Source: (USDA, 2021[39]).
Improvements in TFP have greatly mitigated the upward trend in agricultural emissions by decreasing the emissions intensity of agricultural production (i.e. emissions per unit of output) through the more efficient use of agricultural inputs (higher output per hectare of cropland or pasture, per kg of fertiliser, per animal, per kg of animal feed, and per litre of fuel or kW of electricity). Direct emissions from agriculture grew by approximately 0.5% per year between 1990 and 2016, while crop production grew by an estimated 2.5% per year and livestock production grew by about 1.9% per year over the same period (OECD, 2021[40]). This has primarily been achieved through new production techniques implying substitution of labour by capital and more efficient use of inputs, such as fertilisers, animal feed and land.
TFP growth has also enabled a partial “decoupling” of food production growth and land use change, leading to a more than three-fold increase in agricultural production since 1960, while agricultural land use for crops and pasture grew by only 10-15% over the same period (OECD, 2021[40]). Although land use changes from agriculture are still a major concern, productivity growth has been indispensable in enabling agriculture to feed the world. For instance, in spite of increased fertiliser emissions, past land use intensification is estimated to have led to an overall saving of 590 GtCO2e between 1961 and 2005 through avoided conversion of natural land (Burney, Davis and Lobell, 2010[41]).
Continued improvements in agricultural TFP should therefore contribute to reductions in emissions intensity, both through decreases in the use of emission-intensive inputs and mitigation of land use change. However, it is critical that productivity improvements do not come at the expense of other sustainability dimensions. Productivity improvements do not necessarily lead to a reduction in all input use (substitution may occur) and land use intensification can impact biodiversity and water pollution. This underscores the importance of improving the measurement of TFP to take account of environmental externalities.
Additionally, while TFP growth reduces emissions intensity, this does not necessarily result in lower overall emissions. By lowering agricultural production costs and improving output (and food security), improvements in TFP can also trigger increased production and consumption, thereby offsetting part of the reduction in GHG emissions (Blandford and Hassapoyannes, 2018[26]). This phenomenon is known as the Jevons paradox, which describes the tendency of a resource exploitation to rebound, when its use benefits from an efficiency improvement. This paradox is particularly relevant in the case of large impacts at the margin of agricultural production, such as land use change emissions (Villoria, 2019[42]; Hertel, Ramankutty and Baldos, 2014[43]) or water resource impacts (Grafton et al., 2018[44]).
Notwithstanding these caveats, improving productivity remains fundamental to the mitigation intervention portfolio, in particular for crop and livestock emissions. That said, productivity improvements alone are insufficient and accompanying measures need to be in place to protect natural resources. A better understanding of the trade-offs and synergies in productivity and sustainability efforts is also needed, including through the development of indicators to take account of sustainability impacts in conjunction with TFP increases.
Agricultural soils are also an important reservoir of carbon, and the evolution of these stocks depends importantly on crop and livestock management practices. Crop rotations, residue management, tillage intensity, water management and irrigation practices, and biochar application all affect cropland carbon stocks. On grasslands, management of vegetation, cattle stocking density and grazing pressure, as well as fire management also determine the evolution of soil organic carbon. Although conservation practices involving reduced tillage have shown great efficacy in very dry environments, large uncertainty remains in wetter conditions, and soil carbon sequestration measurement and monitoring is key to ensure the potential from this sink is realised. Overall, the economic mitigation potential from soil carbon sequestration remains considerable, as it could offset 4% of total anthropogenic emissions (Henderson et al., 2022[7]).
Planting trees on agricultural land also appears to be a promising avenue for carbon sequestration. This can take the form of agricultural plantations (e.g. palm oil on existing agricultural land, orchards, dedicated bioenergy crops), agroforestry (combining trees and shrubs with annual crops or livestock), or simply afforestation of agricultural land. The most suitable options will depend on local and market conditions and policy incentives, but could deliver significant temporary or permanent carbon sequestration. However, an important caveat of afforestation on agricultural land is the indirect land use change effect, as agricultural production could be displaced to other areas, driving additional deforestation.
Reducing food losses
Reducing food losses should also provide a significant opportunity to limit emissions related to food production. Food losses occur at all stages of the supply chain, including production, harvesting, transport, storage and distribution. Losses that take place at retail and public or household consumption stage are rather classified as waste (see further below). According to FAO (2019[45]), 14% of food production is estimated to be lost along the supply chain, from post-harvest phase to distribution (but excluding retail). This generates substantial GHG emissions through the need for food production that is not consumed. At the harvest stage, losses can be reduced through better crop quality using agronomic techniques, better timing of harvests and improvements to harvesting equipment. Food losses can be further reduced at the post-harvest stage through better storage infrastructure, and optimisation of food processing facilities, in particular by more efficient transformation processes, improvements in the logistical chain and reduction of contamination. Packaging improvements should also help reducing losses from damages in the logistical chain, while reducing material consumption.
Demand side options: dietary changes and consumer waste reduction
Demand side options have gained increased attention, as the general public becomes more aware of the impact of consumption choices and behaviours on climate change. Two main mitigation opportunities can be identified on the demand side: changes in dietary patterns, and reduction of consumer waste.
The emissions abatement potential of shifting consumption away from food products with high emissions intensities (e.g. ruminant meat and dairy) and replacing them with less emissions-intensive sources of nutrition (such as plant-based or more efficient animal-based protein, see Figure 1.5) has been well-documented (Stehfest et al., 2009[46]; Popp, Lotze-Campen and Bodirsky, 2010[47]; Tilman and Clark, 2014[48]). Estimates of protein consumption levels in different parts of the world (Figure 1.8) reveal significant potential for reductions in animal-based protein in developed economies, while still remaining within the recommended dietary intakes for proteins. According to a broad literature review by the IPCC (2022[5]), changing diets globally has a feasible potential of 1-2.7 GtCO2eq for direct agricultural emissions, and up to 4 GtCO2eq when indirect emissions are also accounted for. For instance, when compared to a conventional (omnivorous) diet, a Mediterranean (less meat and more fruits and vegetables), pescatarian (no meat, more seafood proteins) or vegetarian (only plant based proteins) diet has been shown to deliver important GHG emissions savings globally, as well as substantial health co-benefits (Tilman and Clark, 2014[48]). Food consumption changes towards low emission diets need however to remain nutritionally adequate to be an acceptable option, which requires considering all macro- and micro-nutrients to ensure healthy nutrition (Willett et al., 2019[49]). The feasibility of such large dietary changes at global scale, satisfying both climate and health requirement, is debated. The IPCC indicates that shifting away from high emission intensity products should be feasible in many regions, but economic studies also highlighted the potentially higher cost for the consumer associated with a fully sustainable and healthy diet, in particular in low income regions (Hirvonen et al., 2020[50]).
Note: Meat includes beef and veal, pork, poultry and sheep meat; Other animal products include dairy products, fish and eggs; Plants include vegetable oil, pulses, roots and tubers and cereals (maize, wheat and rice). The recommended WHO dietary intake for protein is 0.83g/kg body weight per day i.e. 58 g/day for a 70 kg adult.
Source: (OECD/FAO, 2021[8]).
Beside climate change and nutritional benefits, dietary changes are also expected to deliver other sustainability benefits. For instance, pasture represents two-thirds of agricultural land globally, rising to 78% of farming land when feed crop requirements are included. The expansion of cattle systems is strongly tied to deforestation patterns and the loss of biodiverse ecosystems in Latin America. Overgrazing is also responsible for large carbon and biodiversity losses for grass-fed systems, while for more intensive ones, high stocking densities lead to nutrient surpluses that are important sources of water pollution. That said, reducing animal products in diets poses immense challenges for livelihoods and for rural development. Livestock represents an important part of agricultural value added in many developed economies, while in poorer regions, rural populations are often dependent on cattle rearing for their basic income and food security. Pasture land cannot always be converted to other agricultural use due to topographical and climate constraints, and animals can also be a source of organic fertilisers that would require substitutes. Transformations would therefore need to be context-specific, progressive and accompanied with appropriate reconversion programmes and social safety nets, as well as local development and landscape adaptation plans.
Besides dietary changes, reducing food waste represents an important potential mitigation option, with possible decreases in the amount of production needed without impacting food consumption (Table 1.1). Beyond behavioural changes driven by increased consumer awareness, possible interventions include regulation and taxation targeting retailers and large businesses; reporting and reduction targets; longer-lasting products; and incentives for consumers to purchase cosmetically imperfect products. Food waste reduction would also bring a number of environmental co-benefits related to lower production; but, again, would also have revenue implications for farmers.
Table 1.1. Waste in food consumption
Copy link to Table 1.1. Waste in food consumption
|
Average food waste (kg/capita/year) |
Global food waste in 2019 (Mt) |
|
---|---|---|---|
|
High income countries |
World* |
|
Household |
79 |
74 |
569 |
Food service |
26 |
32 |
244 |
Retail |
13 |
15 |
118 |
Total |
118 |
121 |
931 |
Note: *World estimates are based on a sample covering 75% of the world population for “Household”, 32% for “Food services” and 14% for “Retail”.
Source: UNEP (2021[51]).
Box 1.4. Bioenergy from agricultural sources
Copy link to Box 1.4. Bioenergy from agricultural sourcesConventional bioenergy is not a supply-side option for mitigating agricultural emissions in a strict sense, as its main mitigation effect comes from the replacement of fossil fuels consumed in the rest of the economy. Because the absorption of CO2 during plant growth offsets emissions subsequently generated through the combustion of the biomass, the convention is to not account for emissions resulting from their combustion. This assumption, called carbon neutrality, does not mean that biofuels completely offset fossil fuel emissions, because the cultivation, collection and transformation of feedstocks to produce these fuels is also a source of GHG emissions. The final levels of GHG savings associated with biofuels is determined by a life cycle assessment (LCA), comparing emissions from the biofuels supply chain with the emissions from fossil fuel alternatives along their production and combustion cycle (OECD, 2008[52]).
The largest source of bioenergy at present is solid biomass from forests used in energy power plants (modern biomass) and traditional use at home for cooking and heating through wood collection, common in developing countries (traditional biomass, considered unsustainable). In contrast, agricultural feedstocks are used mainly for the production of liquid biofuels and biogas, and their use has recently become more mainstream. The most common feedstocks for biofuels are sugar cane in Brazil and maize in the United States, both of which are converted into ethanol, as well as vegetable oils from palm and rapeseed that are used for biodiesel, for instance in Europe and Southeast Asia.
Feedstocks derived from crops that are used for biofuels, also called first-generation feedstocks, show relatively mixed performance in terms of GHG savings, and have made a limited contribution to overall mitigation to date (OECD, 2019[25]). More advanced feedstocks based on agricultural residues (sugar cane bagasse, cereal straw, corn stover, rice husk) are often considered more promising, but their availability remains limited, and, similar to first-generation feedstocks, may compete with alternative uses. These feedstocks can be used as solid biomass or transformed through more advanced processes to be used as liquid biofuels with much higher environmental benefits. Similarly, dedicated lignocellulosic crops can be grown to produce such fuels with higher efficiency, and with the possibility of growing on marginal land. Yet these so-called second-generation energy crops remain expensive to convert into fuels and their deployment currently remains limited in scale.
Biofuels may also be a direct mitigation option for the AFOLU sector. Biodigesters reduce non-CO2 emissions from manure management and produce biogas that substitutes for fossil fuel energy sources. Dedicated energy crops can also sequester carbon in the soil through reduced tillage management, whereas palm tree and wood plantations increase carbon storage time in landscape vegetation. Bioenergy with carbon capture and sequestration (BECCS) involves reinjecting emissions from the biofuel production process into geological reservoirs. Although considered as the most efficient route, this latter technology is not mature at the present time.
The overall environmental benefits of land-based biofuels depend not only on their local management, but also on their indirect effects on land use. The displacement of crops and animals to convert land to biofuels has been heavily debated as a possible source of additional GHG emissions that may occur in different regions of the world and the associated potential emissions are generally not considered in LCA. For this reason, some countries have considered additional safeguards or restrictions on their use (minimum emission saving thresholds, incorporation rate caps, or specific certification criteria). Due to the significant need for decarbonisation across the rest of the economy to achieve carbon neutrality, bioenergy will likely remain part of the mitigation options to which agriculture will need to contribute, in particular for sectors that currently have limited alternatives to reduce their emissions (e.g. international aviation), and where land use impacts can be contained.
Mitigation measure potentials
The potential contribution of the different measures above to climate change mitigation varies depending on their nature, the sources they are targeting and the regions where they are applied. The IPCC estimates that AFOLU as a whole has a technical potential of 28 GtCO2eq per year, bioenergy excluded, which is about half of annual anthropogenic emissions over 2010-2019. The economic potential would, however, be lower, with 8-14 GtCO2eq of reduction per year achievable at a cost less than USD 100 per tCO2eq, 30-50% of which would be actionable for less than USD 20 per tCO2eq. The detailed mitigation potentials per AFOLU action area are presented in Table 1.2 below, as identified by the IPCC, based on bottom-up sectoral assessments, and corresponding to the upper bound of the 8-14 GtCO2eq total feasible economic potential.19 Supply side measures represent an economic mitigation potential of about 10 GtCO2 per year, but only 0.6 GtCO2eq are estimated as being achievable through reductions of on-farm non-CO2 emissions, representing only 10% of these agricultural sources, underscoring the importance of action in the LULUCF domain. Agricultural soils can in particular contribute 1.6 GtCO2eq per year according to IPCC, which is also consistent with the estimate from Henderson et al. (2022[7]).
Demand side measures are shown to have relatively high potential, at 4.2 GtCO2eq per year, and could reach much higher levels if land expansion emission savings, as well as other supply chain emissions, are also accounted for (up to 8 GtCO2eq per year).20 The comparative cost-efficiencies of demand-side versus supply-side measures are subject to debate. The IPCC estimates an economically feasible potential of 2.2 Gt CO2eq per year for demand side measures, but many of these options depend on consumer behavioural changes, which, while potentially requiring lower upfront investment, may be hard to introduce due to normative, cultural and institutional resistance. In terms of efficacy, both channels of action are seen as having significant mitigation potential for the same sources (OECD, 2019[25]), and there is a growing consensus on the need to use both in combination.
The current composition of food production, relying on a large share of land and crop production dedicated to animal products, is at the core of the climate mitigation challenge for agriculture. However, it is also important to recognise the heterogeneity of emission intensities on the supply side. Targeting emissions hotspots and addressing the most inefficient and emissions-intensive producers could drastically reduce GHG emissions without necessarily impacting consumption.21
Table 1.2. Global AFOLU abatement potentials relevant for agriculture, 2020-2050 time horizon
Copy link to Table 1.2. Global AFOLU abatement potentials relevant for agriculture, 2020-2050 time horizonAverage IPCC estimates (with reviewed range in parenthesis) – GtCO2eq
|
Abatement technical potentials |
Abatement economic potentials (cost < USD 100 per tCO2eq) |
---|---|---|
Supply side measures |
21.3 (5.4 – 49.6) |
10.0 (4.9 – 17.4) |
Direct on farm emissions |
1.7 (0.5 – 3.2) |
0.6 (0.3 – 1.3) |
Crops cultivation |
0.3 (0.06 – 0.7) |
0.2 (0.05 – 0.6) |
Rice cultivation |
0.3 (0.1 – 0.8) |
0.2 (0.05 – 0.3) |
Enteric fermentation |
0.8 (0.2 – 1.2) |
0.2 (0.1 – 0.3) |
Manure management |
0.3 (0.1 – 0.5) |
0.1 (0.09 – 0.1) |
Land use and agricultural soils* |
19.6 (4.9 – 46.4) |
9.4 (4.6 – 16.1) |
Deforestation |
4.5 (2.3 – 7.0) |
3.4 (2.3 – 6.4) |
Afforestation and reforestation |
3.9 (0.5 – 10.1) |
1.6 (0.5 – 3.0) |
Other LUC conversion |
0.2 (0.1 – 0.4) |
0.04 (0.0 – 0.1) |
Peatlands protection and restoration |
1.6 (0.9 – 3.3) |
0.9 (0.4 – 1.3) |
Soil organic carbon |
||
Cropland |
1.9 (0.4 – 6.8) |
0.6 (0.4 – 0.9) |
Grassland |
1.0 (0.2 – 2.6) |
0.9 (0.3 – 1.6) |
Biochar |
2.6 (0.2 – 6.6) |
1.1 (0.3 – 1.8) |
Agroforestry |
4.1 (0.3 – 9.4) |
0.8 (0.4 – 1.1) |
Other AFOLU non relevant for agriculture |
2.9 (1.2 – 8.4) |
1.4 (0.7 – 2.4) |
Demand side measures** |
4.2 (2.2 - 7.1) |
2.2 (1.1– 3.6) |
Diet change*** |
N/A |
1.7 (1.0 – 2.7) |
Food waste and losses*** |
N/A |
0.5 (0.0 – 0.9) |
TOTAL AFOLU (agriculture related)* |
25.5 (7.6 – 56.7) |
12.2 (6.0 – 21.0) |
Note: Land use categories relevant for agriculture indicate the full mitigation potential of the category, even if only a part of it can be achievable through the agricultural sector (e.g. afforestation). All estimates are based on sectoral assessment data and reflect averages. Uncertainty ranges are documented in IPCC (2022[5]).
* Total excluding “Other AFOLU non relevant for agriculture”, featured for completeness. The following categories are not accounted: forest management, fire management, coastal wetlands protection and restoration. ** IPCC only provides a total estimate for technical potential of demand side measures. The split between dietary change and food waste is however available for economically feasible potentials. *** Estimates corresponding to avoided agricultural production emissions (land use change excluded to limit double-counting).
Source: (IPCC, 2022[5])
What are countries doing to mitigate agricultural emissions?
Copy link to What are countries doing to mitigate agricultural emissions?Considering the role that AFOLU has to play to meet the 2015 Paris Agreement’s objectives, ambitious policy action on agriculture is needed to ensure that countries take advantage of the opportunities available for mitigation. This section provides an overview of the targets set by countries and the policies introduced to mitigate agricultural emissions. While the coverage is not exhaustive, it aims to shed light on the main policies and instruments relating to mitigation in agriculture.
Setting mitigation targets for agriculture
All of the 54 countries covered in this report have submitted Nationally Determined Contributions (NDCs) under the Paris Agreement of the United Nations Framework Convention on Climate Change (UNFCCC). However, national ambitions and commitments to mitigate emissions vary considerably across countries (Table 1.3). While most of the countries covered in this report have set intermediate targets for 2030 and net zero targets for 2050 (or in some cases, 2060 or 2070), not all countries have established these as binding targets within their legislation. Out of the 54 countries covered in this report, 36 countries (plus the European Union as a whole) have communicated their long-term strategies to the UNFCCC.
Although agricultural emissions are included in most countries’ NDCs, only 16 out of the 54 countries have set specific emissions reduction targets for their agricultural sectors. Where agricultural targets have been defined by countries, they are typically lower than the reductions needed to stabilise global temperatures at 2°C (Henderson, Frezal and Flynn, 2020[53]).
Table 1.3. Economy-wide and agriculture-specific GHG mitigation targets
Copy link to Table 1.3. Economy-wide and agriculture-specific GHG mitigation targets
Economy-wide emissions reduction targets |
Long-term strategy submitted to UNFCCC |
Agriculture-specific target (base year/level) |
Global methane pledge (reduce global CH4 -30% from 2020 levels by 2030) |
||
---|---|---|---|---|---|
2030 target (base year/level) |
2050 target |
||||
Argentina |
Max 359 MtCO2eq |
None |
No |
None |
Yes |
Australia |
-26-28% (2005) |
Net zero |
Yes |
None |
No |
Brazil |
-50% (2005) |
Net zero |
No |
None |
Yes |
Canada |
-40-45% (2005) |
Net zero |
Yes |
-30% fertiliser emissions by 2030 (2020) |
Yes |
Chile |
Max 95 MtCO2eq |
Net zero |
Yes |
None |
Yes |
China |
Peak CO2 ; -65% GDP emission intensity (2005) |
Net zero by 2060 |
Yes |
None |
No |
Colombia |
-51% (BAU) |
Net zero |
Yes |
None |
Yes |
Costa Rica |
Max 9.11 MtCO2eq |
Net zero |
Yes |
None |
Yes |
European Union |
-55% (1990) |
Net zero |
Yes |
None at EU level |
Yes |
EU Member States |
18 out of 27 countries (except BGR, CYP, EST, GRC, HRV, IRL, ITA, POL, ROU) |
2030 targets: BEL -25% (2005); DNK -55% (1990); DEU -31-34% (1990); FRA -18% (2015); IRL -22-30% (2018) PRT -11% (2005) |
19 out of 27 countries (except AUT, CZE, HUN, LVA, LTU, POL, ROU, SVK) |
||
Iceland |
-55% (1990) |
“Largely neutral” by 2040 |
Yes |
None |
Yes |
India |
-45% GDP emission intensity (2005) |
Net zero by 2070 |
No |
None |
No |
Indonesia |
-29% from BAU; up to -41% conditional on int. support |
Net zero by 2060 |
Yes |
None |
Yes |
Israel |
-27% (2015) |
-85% from 2015 levels |
No |
None |
Yes |
Japan |
-46% (2013) |
Net zero |
Yes |
49.5 MtCO2eq by 2030 |
Yes |
Kazakhstan |
-15% (1990) |
None |
No |
None |
No |
Korea |
-40% (2018) |
Net zero |
Yes |
-27.1% by 2030; -37.7% by 2050 (2018) |
Yes |
Mexico |
-22% (BAU); up to -36% conditional on int. support |
None |
Yes |
-8% by 2030 (BAU) |
Yes |
New Zealand |
-50% (2005) |
Net zero except methane |
Yes |
-24-47% reduction in biogenic methane by 2050 |
Yes |
Norway |
-50-55% (1990) |
-90-95% (1990) |
Yes |
Voluntary agreement with agriculture sector: -5 MtCO2eq by 2030 |
Yes |
Philippines |
-2.7% (2020); up to -75% conditional on int. support |
None |
No |
-29.4% by 2030 (BAU) conditional on int. support |
Yes |
Russia |
-30% (1990) |
Net zero by 2060 |
No |
None |
No |
South Africa |
350-420 MtCO2eq (BAU 398-614 MtCO2eq) |
None |
Yes |
None |
No |
Switzerland |
-50% (1990) |
Net zero |
Yes |
-40% by 2050 (1990) |
Yes |
Turkey |
-21% (BAU) |
Net zero by 2053 |
No |
None |
No |
Ukraine |
-65% (1990) |
Net zero by 2060 |
Yes |
None |
Yes |
United Kingdom |
-68% (1990) |
Net zero |
Yes |
-17-30% by 2030 ; -24-40% by 2035 (2019) |
Yes |
United States |
-50-52% (2005) |
Net zero |
Yes |
None |
Yes |
Viet Nam |
-9% (BAU); up to -27% conditional on int. support |
Net zero |
No |
-20% every 10 years |
Yes |
Some countries have set targets to reduce specific GHGs, such as methane or nitrous oxide emissions. Under the Zero Carbon Amendment Act 2019, New Zealand has set separate long-term emission reductions targets for long-lived and short-lived GHG emissions. This includes a specific objective for methane, targeting a reduction in biogenic methane emissions of 10% by 2030 and 24-47% by 2050 (relative to 2017 levels). Canada set a national target to reduce emissions from fertilisers by 30% from 2020 levels by 2030, and will work with fertiliser manufacturers, farmers, provinces and territories to develop an approach to meet the target. China’s first NDC submitted in 2016 included a target for achieving zero growth in fertiliser and pesticide utilisation by 2020, which the government reported as achieved in 2018, as well as broad objectives to control methane emissions from rice fields and nitrous oxide emissions from farmland. Korea set a target of reducing methane emissions by 30% by 2030 (relative to 2018 levels), and 20.6% in the agricultural sector.
Policy levers to mitigate agricultural emissions
Governments have a range of policy instruments at their disposal to mitigate agricultural emissions. These can be divided into four broad categories: emissions pricing instruments; agricultural support, grants and preferential credits; environmental regulations; and R&D and knowledge transfer.
Research shows that these policy approaches perform quite differently with respect to their effectiveness in reducing emissions, cost effectiveness, and impacts on producers, consumers and government budgets (OECD, 2019[25]). Emissions pricing instruments based on the “polluter pays principle”, by either taxing emissions or establishing tradeable permits, are the most effective at reducing emissions for a given carbon price because they provide incentives to adopt low emission measures, switch from higher to lower-emission commodities, and scale back overall production and emissions. These policies also raise revenue for governments. On the other hand, they also impose costs on producers, particularly farmers producing emission-intensive commodities, and consumers, and these welfare impacts need to be managed. Regulations restricting specific high emission practices can also impose costs on producers and consumers, but they lack the efficiency and cost effectiveness of policies based on the “polluter pays principle” (Baumol and Oates, 1988[54]).
Policies based on the “beneficiary pays principle” that subsidise emission reductions can provide an alternative market-based approach, and one which does not impose costs on producers or raise food prices. However, these policies require careful design to ensure that producers are not over-compensated, they tend to be less effective, and they can impose large costs on governments and other sectors purchasing emission reductions (if implemented on a large scale). The use of grants to support the adoption of low emission practices, either directly or via cross-compliance requirements, shares some similarities with abatement subsidies. However, since grants do not use competitive market-based approaches to disburse funds and typically do not have stringent emission measurement requirements, they do not set an explicit carbon price and are less efficient than abatement subsidies (OECD, 2019[25]).
Other supply-side mitigation policy approaches such as R&D and knowledge transfer, and preferential credit schemes are particularly relevant for stimulating the adoption of profitable mitigation measures that are un- or under-utilised due to knowledge and financing barriers. They can also provide an enhanced enabling environment to improve the performance of other mitigation policies and, in the case of R&D and knowledge transfer, they can stimulate innovation and competitiveness over the longer term and help drive down emissions without imposing costs on producers and consumers (OECD, 2019[25]). Investment in accurate and affordable measurement, reporting and verification (MRV) procedures and technologies is also critical, particularly for enabling the efficient functioning of emission pricing policies.
Table 1.4 presents specific policy instruments corresponding to these categories and some selected examples of countries that have applied these instruments.
Table 1.4. Policy levers to mitigate agricultural emissions
Copy link to Table 1.4. Policy levers to mitigate agricultural emissions
Policy category |
Specific instrument |
Examples |
---|---|---|
Emissions pricing instruments |
Emissions taxes |
|
Emissions trading schemes / carbon offsets |
New Zealand (NZ ETS) |
|
Abatement subsidies / auctions |
Australia (Emissions Reduction Fund) |
|
Agricultural support, grants and preferential credits |
Agricultural support |
EU (CAP); Canada; other countries |
Grants |
United States (biogas); China; Australia |
|
Dedicated credit line |
Brazil (ABC programme) |
|
Environmental regulations |
Pollution regulations |
EU (Nitrates directive and pollution control) |
R&D and knowledge transfer |
R&D |
Global Research Alliance |
Knowledge transfer |
Multiple countries |
Emissions pricing instruments
Emissions pricing instruments aim to influence incentives for production and consumption. Mechanisms that put a price on emissions include carbon pricing through emissions taxes and emissions trading schemes, carbon offsets, and some abatement subsidies (e.g. those that are delivered via auctions). There are relatively few examples of countries that have introduced emissions pricing to mitigate agricultural emissions.
Australia’s Emissions Reduction Fund (ERF) was established in 2015 and is a voluntary scheme providing incentives for businesses to undertake emissions reductions and carbon sequestration projects that meet strict integrity requirements, including in relation to additionality. For agriculture, landowners and farmers can earn income by generating Australian Carbon Credit Units (ACCUs) for every tonne of emissions reduced or carbon stored through a project, and selling these to the government or to third parties. As of April 2022, the ERF had committed AUD 2.7 billion (USD 2 billion) through 14 auctions for a total of 217 MtCO2eq of abatement, including 15.2 MtCO2eq of agricultural emissions (of which just 1.1 MtCO2eq of abatement has been delivered so far). Japan introduced the J-Credit scheme in 2013, providing certified carbon credits for emissions reductions and carbon sequestration activities such as introduction of energy-saving technologies and forest management. As of January 2022, 107 projects were registered in the agriculture, forestry and fisheries sectors, with expected emission reductions or avoidance totalling 1.5 MtCO2eq.
Ultimately, the scale of such voluntary market-based approaches is limited by the availability of funding from the government and private sector to pay producers for emissions reductions (Henderson, Frezal and Flynn, 2020[53]). Combining emissions abatement with other environmental services offers one possibility for farmers to increase and diversify their sources of funding. Australia recently launched the Carbon + Biodiversity Pilot, trialling a market-based approach to pay farmers for long-term biodiversity improvements, on top of income they can earn from the ERF for carbon sequestration projects. Landholders are required to plant, manage and maintain their carbon plantings in line with biodiversity protocols developed by the Australian National University.
Emissions pricing instruments that apply the “polluter pays” principle are not subject to these constraints. New Zealand has developed an Emissions Trading Scheme, which covers all sectors of the economy, although it does not currently cover methane and nitrous oxide emissions from agricultural production. Forestry emissions are included in the scheme, increasing the incentives for farmers and landowners to reduce deforestation and store carbon by converting pastureland to forests. Companies in the agricultural supply chain (e.g. meat processors, dairy processors, nitrogen fertiliser manufacturers and importers) are required to report on their agricultural emissions, but are not required to pay for their emissions. The New Zealand ETS also imposes a cost on emissions from transport fuels, electricity production, synthetic GHGs, waste and industrial processes, including in the primary sectors. Options for pricing agricultural emissions are currently under discussion.
Agricultural emissions tend to be excluded from most other economy-wide carbon pricing schemes, and are often dealt with through other mechanisms. The EU emissions trading scheme (EU-ETS) provides a framework for emissions reductions in the power, manufacturing and aviation industries, but does not include agricultural emissions, which are subject to annual mitigation targets under the EU’s Effort Sharing Decision (for non-CO2 emissions from agriculture) and the LULUCF Decision (for CO2 emissions from land use change). The Korean Emissions Trading Scheme (KETS) was introduced in 2015, and imposes emission reduction obligations on companies that exceed a defined GHG emissions threshold. While agriculture is not currently included in the KETS, the Korean Ministry of Agriculture, Food and Rural Affairs operates voluntary emission reduction and offset projects to reduce emissions in the agricultural sector, and subsidises the cost of verification. Farmers can obtain certified offset credits for emission reduction projects and sell these in the emissions trading market. Several regional and state-level emissions trading schemes are in place or in the process of being set up in the United States, including in California, Washington, Connecticut, Delaware, Maine, Maryland, Massachusetts, New Hampshire, New Jersey, New York, Rhode Island, Vermont and Virginia. While agriculture is not required to reduce GHG emissions under these programmes, it is a permitted source of offsets in all of them.
Several countries have introduced taxes on emissions, but these also exclude the agricultural sector. Canada’s carbon pollution pricing system, in place in every jurisdiction since 2019, largely excludes the agricultural sector. Indonesia has established legislation to introduce a carbon tax, due to be implemented in July 2022. The carbon tax is initially limited to coal fired power plants, and will provide the basis for the development of a broader carbon tax mechanism and a carbon exchange where companies can trade their emissions permits by 2025. Norway’s agricultural sector is largely exempt from the country’s carbon tax, with the exception of emissions from fossil fuel use in agriculture. South Africa introduced a national carbon tax under the 2017 Carbon Tax Act, however primary agriculture is exempted from the current Phase 1.
Grants, income support and credit programmes
Subsidised loans are sometimes used as a tool to encourage emissions reductions in agriculture. Brazil’s Low Carbon Agricultural Programme or GHG Emission Reduction Program (ABC programme) was launched in 2010, and provides resources and incentives to farmers for adopting sustainable agricultural practices and technologies. The ABC programme provides low-interest loans to farmers for activities that reduce emissions, such as recovering fragile areas and pastureland, expanding integrated crop-livestock-forestry systems and no-till farming, adopting forest conservation practices, improving unproductive and degraded soils, forest planting, organic agriculture, bio-inputs and bio-fertilisers and renewable energy generation for agriculture. The programme reduced an estimated 166 MtCO2eq of emissions over the period from 2010 to 2019. The United States has introduced initiatives providing preferential credit and grants to promote the adoption of GHG mitigation practices. For example, the Rural Energy for America Program (REAP) provides guaranteed loan financing and grant funding to agricultural producers for the adoption of renewable energy systems and energy efficiency improvements. The AgSTAR: Biogas Recovery in the Agriculture Sector programme helps producers to find information and financing for biogas recovery systems to reduce methane emissions from livestock waste.
Several countries have provided funding for initiatives that promote afforestation and soil carbon sequestration. Canada’s Natural Climate Solutions Fund has made more than USD 2 billion available over ten years to plant two billion trees, USD 48 million over two years to protect existing wetlands and trees on farms, and USD 470 million for projects that conserve, restore and enhance wetlands, peatlands and grasslands. China’s Grain for Green programme was introduced in 2000 and provides direct payments to farmers to re-establish forest and shrub vegetation on sloped cultivated land at risk of erosion, and to afforest large tracts of barren land. The programme is estimated to have achieved 29 million hectares of afforestation, including converting 9 million hectares of cropland to forestland. India’s Ministry of Environment, Forest and Climate Change has been conducting the National Afforestation Programme since 2000, targeting community-based activities such as agro-forestry, improved soil conservation and restoration of degraded forests. Ireland’s Afforestation Scheme was established in 2014 and provides grants and financial support to encourage the establishment and maintenance of new forests and woodlands.
Ukraine introduced the Green Country large-scale afforestation of Ukraine initiative, which aims to plant one billion trees over the next three years, and increase forested areas by one million hectares over the next ten years. The United Kingdom has introduced several new environmental land management schemes that contribute to the mitigation of emissions through tree planting and peatland management and restoration. These include England’s Farming in Protected Landscapes Scheme, Scotland’s Forestry Grant Scheme, and Wales’ Forests for Our Future Programme. The United States set up the temporary Pandemic Cover Crop Program (PCCP), which encourages the adoption of cover crops by providing reduced crop insurance premiums for producers who plant a qualifying cover crop during the 2021 or 2022 crop years.
India has several programmes in place to reduce emissions from rice production, including systems of rice intensification under the National Food Security Mission (currently being implemented in 24 states), providing farm equipment to enable timely sowing in standing paddy residues, and Custom Hiring Centres and Farm Machinery Banks to enable sowing of wheat crops without the burning of paddy residues. Japan provides farmers with direct payments for mitigation activities, such as applying compost, extending the period of mid-season drainage in paddy rice fields, and reducing the use of synthetic fertilisers. Area-based payments are provided to dairy farmers for implementing environmentally friendly practices such as no-till farming in conjunction with reducing the use of synthetic fertilisers and pesticides. Investment support is provided to farmers for introducing renewable energy, biogas plants and composting facilities for better manure management and clean energy production, and biomass-based greenhouse heating systems in horticulture. Since 2014, Switzerland has provided resource efficiency contributions to farmers to support the use of environmentally friendly techniques such as conservation tillage systems (no-till, strip-till and mulch tillage), emission-reducing application methods for farmyard manure, and nitrogen-reduced phase feeding of pigs.
Environmental regulations
Regulatory policy instruments to reduce diffuse pollution from agricultural inputs such as fertilisers and manure can also have a strong influence on agricultural GHG emissions. The EU Nitrates Directive was established in 1991 and aims to prevent nitrate pollution of surface water and groundwater resources by promoting the use of good farming practices. While nitrate is not a GHG, by restricting nitrogen inputs into the agricultural system the directive also helps to mitigate important sources of nitrous oxide emissions. This includes establishing limitations on the application of nitrogen fertilisers and livestock manure on land, restricting livestock stocking rates, setting minimum storage requirements for livestock manure, and establishing crop rotations, soil winter cover and catch crops to prevent nitrate leaching and runoff. Norway has established regulations for manure and fertiliser management to control emissions from these sources, and restricted the cultivation of peat bogs to prevent additional emissions from organic soils. As of 2025, agricultural buildings will be prohibited from using fossil fuels for heating (this ban is in effect for other building types as of 2020). Switzerland’s water quality plan adopted in 2022 introduces a minimum reduction target of 20% for nitrogen and phosphorus fertiliser losses by 2030. More stringent environmental cross-compliance requirements relating to manure application will further increase farmers’ incentives to reduce their use of fertilisers.
EU cross-compliance rules can also support efforts to mitigate agricultural emissions by requiring farmers to respect EU rules on public, animal and plant health, animal welfare and the environment. In addition, farmers receiving CAP support are required to respect EU standards on good agricultural and environmental condition of land, including standards to prevent soil erosion, maintain soil organic matter and soil structure, maintain permanent grassland, protect biodiversity and protect and manage water. In Kazakhstan, some interest rate subsidies provided to livestock producers come with an obligation to rehabilitate their pasture lands, which could potentially help to lower agricultural emissions. Korea introduced a direct payment system with enhanced environmental cross-compliance requirements and increased green coverage through expanded urban farming. The United States also ties eligibility for federal farm programmes and subsidies to conservation practices.
Deforestation is an important driver of agricultural emissions in many countries. In Argentina, the 2007 National Law of Native Forests, the 2015 National Forest Management Plan with Integrated Livestock (MBGI) and the Law for the Promotion of Forests are efforts to ensure good practices and curb deforestation. Brazil’s Forestry Code contains regulations to constrain land use change, and makes access to subsidised credit conditional on compliance with environmental regulations.
Regulations to promote biofuels can to some extent contribute to reductions in emissions from fossil fuels. For example, Canada’s Clean Fuel Standard (CFS) will support the domestic production of biofuels by requiring liquid fuel suppliers to gradually reduce the carbon intensity of their fuels over time. The CFS will establish a regulated market for carbon credits, allowing ethanol and bio-diesel producers to earn credits for supplying low carbon intensity biofuels. It is complemented by the government’s recent USD 1.1 billion investment in the Low-carbon and Zero-emissions Fuels Fund, which will support the domestic production of feedstocks for biofuels.
R&D and knowledge transfer programmes
Many of the countries covered in this report provide funding for R&D and knowledge transfer programmes to support the mitigation of agricultural GHG emissions. Australia’s Long-Term Emissions Reduction Plan sets out the government’s plan to achieve net zero emissions by 2050. It includes the Technology Investment Roadmap, which aims to accelerate the development and commercialisation of new and emerging low emissions technologies, including in the agricultural sector. Canada’s 2030 Emissions Reduction Plan provides USD 366 million for the On-Farm Climate Action Fund to help farmers adopt climate friendly practices such as nitrogen management, cover cropping and rotational grazing, and USD 234 million for the Agricultural Clean Technology Programme to support R&D, commercialisation and the adoption of new clean technologies for the agricultural sector. In addition, USD 78 million is provided for Transformative Science and USD 117 million is allocated for a resilient Agricultural Landscape Programme. Chile’s Long-Term Climate Strategy was launched at COP26 in November 2021, and contains several objectives relating to the promotion of R&D and extension services to reduce agricultural emissions. Under the Agricultural Technology Development and Application Plan to Achieve Carbon Neutrality by 2050, Korea’s Rural Development Administration is working to expand the development of low-carbon technologies for agriculture such as alternate wetting and drying for rice cultivation and recycling of livestock manure, increase the use of renewable energy and energy-efficient technologies, and enhance the carbon sequestration capacity of soils. The United States Department of Agriculture’s Climate Hubs develop science-based information and technologies, and deliver them in co-operation with USDA agencies and partners to support the implementation of climate-smart practices. State-level extension services also provide outreach, training, technical assistance and on-farm testing of climate mitigation practices.
A number of countries have established research initiatives to tackle livestock emissions. Australia is providing USD 23.1 million in funding over six years for the Methane Emissions Reduction in Livestock (MERiL) grants programme. MERiL supports trials, development and commercialisation of new livestock feed technologies and low-emission feed supplement delivery technologies to reduce enteric methane emissions from cattle and sheep. China launched several collaborative research projects with academia and the private sector in 2018, to identify novel feed solutions and estimate emission reductions from more sustainable dairy farming practices. Colombia is currently implementing several projects on sustainable livestock production and has established the Roundtable on Sustainable Livestock, an inter-agency public-private body for technical consultations. Costa Rica trained 200 extension service providers to formulate diets for animal feed, monitor livestock farms, and implement mitigation actions such as rotational grazing and silvopasture systems. New Zealand’s He Waka Eke Noa – Primary Sector Climate Action Partnership provides extension and advisory services for farmers to measure and manage their emissions, and R&D investments in mitigation technologies such as methane inhibitors and a methane vaccine. The New Zealand Government also researches mitigation technologies for ruminant livestock through the New Zealand Agricultural Greenhouse Gas Research Centre, the Pastoral Greenhouse Gas Research Consortium, and in co-ordination with the member countries of the Global Research Alliance on Agricultural Greenhouse Gases.
Several countries are supporting the development of climate-smart agriculture. Iceland is implementing the Climate-Friendly Agriculture project, which provides comprehensive advice and education to farmers with the aim of reducing GHG emissions from agriculture and land use. The project is a part of the 2020 Climate Action Plan, which also includes actions to reduce the use of mineral fertilisers, improve livestock feeding to reduce enteric fermentation, increase domestic vegetable production, and achieve carbon neutrality in cattle breeding. India has increased funding for R&D in technologies to convert agricultural stubble into biogas or other energy products. The Indonesian Agency of Agriculture Research and Development has established a number of R&D and extension programmes focusing on climate-smart practices and technologies, including the development of plant varieties resistant to climate stress, a planting calendar adjustment system, and efficient agricultural equipment and machinery.
Israel has introduced several programmes to strengthen conservation and regenerative agricultural practices (e.g. minimum tillage, cover crops, applying organic matter to soils), reduce the use of natural and synthetic fertilisers, improve the treatment of organic agricultural waste, develop know-how for climate-smart agriculture, protect trees and forests to sequester carbon, and facilitate the role of farming in renewable energy production. Mexico’s agricultural sector strategy promotes agricultural practices adapted to climatic and environmental conditions, such as soil conservation and reduced burning of residues, considering community and scientific knowledge; and adopting agroforestry, agroecology and biodigesters on livestock farms. The Philippines is promoting new technologies and practices to reduce emissions, such as Alternate Wetting and Drying for irrigated rice cultivation, microbial inoculants, biochar, livestock feed supplements and nature-based solutions. Ukraine has introduced minimum-tillage techniques and a ban on stubble burning in fields, improved agricultural practices in zones vulnerable to nitrate pollution, increased support for restoring degraded land, and is supporting the use of manure in biogas production.
Building the capacity for measurement, reporting and verification (MRV) of farm-level emissions can help to pave the way for the introduction of carbon pricing policies. Australia launched the three-year USD 38.1 million National Soil Carbon Innovation Challenge to identify and fast-track low-cost, accurate technological solutions for measuring soil carbon at below USD 2.25 per hectare per year on average. The five-year USD 5.9 million Soil Carbon Data Programme is working to improve soil carbon data, build confidence in low-cost alternatives for measuring and estimating soil carbon, and contribute to a national soil data repository. Viet Nam’s Ministry of Agriculture and Rural Development is also establishing MRV systems for agriculture and LULUCF under its Plan to Implement the Paris Agreement on Climate Change for 2021‑30.
International policy initiatives
The Global Research Alliance on Agricultural Greenhouse Gases was launched in 2009, and includes 65 member countries that work together to increase co-operation and investment in R&D to reduce the emissions intensity of agricultural production and increase the potential for soil carbon sequestration. The Coalition on Sustainable Productivity Growth for Food Security and Resource Conservation aims to accelerate the transition to more sustainable food systems through sustainable agricultural productivity growth. It was launched at the 2021 UN Food Systems Summit and is supported by 46 countries (including the European Union), as well as a broad range of academic and research organisations, private sector associations, and industry bodies.
Several international initiatives were launched at COP26 in Glasgow in November 2021, including:
The Global Methane Pledge, signed by over 100 countries including 29 OECD members and the European Union as a whole. Countries joining the Pledge agreed to take voluntary actions to reduce global methane emissions by at least 30% from 2020 levels by 2030, potentially eliminating over 0.2°C of warming by 2050. While the target is global and any reductions in national methane emissions are made on a voluntary basis, participation sends a strong signal of a country’s willingness to substantially reduce its methane emissions by 2030.
The Glasgow Leaders’ Declaration on Forests and Land Use was signed by 141 countries, and calls for efforts to halt and reverse forest loss and land degradation by 2030 through efforts to conserve and restore forests and other terrestrial ecosystems and accelerate their restoration.
The Agriculture Innovation Mission for Climate (AIM for Climate) was launched at COP26 with 31 countries and over 48 non-government partners, and aims to significantly increase investment in agricultural innovation for climate-smart agriculture and food systems over the next five years. The initiative will also support technical discussions and promote expertise across international and national levels of innovation, and will facilitate co-operation on climate-related agricultural innovation on shared research priorities.
The Policy Action Agenda for Transition to Sustainable Food and Agriculture sets out pathways and actions that countries can take to repurpose public policies and support to food and agriculture, to deliver these outcomes and enable a just rural transition.
Impacts of current agricultural support on climate change
Copy link to Impacts of current agricultural support on climate changeAgricultural support policies have significant consequences for climate change and environmental sustainability. Governments across the 54 countries covered in this report provided USD 817 billion per year in transfers to agriculture in 2019-21, of which USD 611 billion per year was provided as positive support to individual producers. The remainder was almost equally split between support for general services (USD 106 billion) and budgetary transfers to consumers (USD 100 billion). Some emerging economies also implicitly taxed their producers by an average of USD 117 billion per year.
Support policies can influence GHG emissions and environmental outcomes in different directions depending on their design. By changing agricultural market prices, they can influence farmers’ decisions to produce and the emissions generated through changes in production volume. If they target input or factor prices, they can also affect the way in which farmers produce, by encouraging substitution between intermediate inputs and primary production factors (e.g. land, capital and labour), affecting the emissions intensity of production, either on the farm or through changes in land use (Henderson and Lankoski, 2019[55]). Due to these effects, support policies, whether provided in the form of market transfers or budgetary payments, can work against other policy interventions for climate change mitigation.22 Support policies can also be targeted to incentivise environmentally beneficial practices, or can provide for broader general services with potential to support emissions reductions, such as support for R&D and innovation.
Against this background, this section discusses the impacts of current support policies on GHG emissions reduction incentives and efforts.
Emissions impacts of production support policies
Direct agricultural support for the production of specific commodities
Agricultural support policies are often aimed at facilitating the production of specific commodities. For example, market price support (MPS) corresponds to policies that create a price gap between domestic market prices and border prices for specific agricultural commodities. Import licences, tariffs, tariff rate quotas and minimum prices are examples of measures that result in higher prices paid by consumers. MPS increases the price received by the producer, thus providing incentives for additional production, the intensification of input use, the allocation of land to supported crops, and the entry of land to the agricultural sector. Other types of direct production support include coupled payments, whether they are based on output, current cultivation area, or number of animals. These also typically encourage farmers to increase their production, either through intensification, expansion of land, or the retention of farms that would be financially unviable without support. On the consumer side, however, the impacts of these interventions differ: MPS increases market prices, which (all else equal) will result in reduced domestic consumption. On the other hand, coupled payments decrease market prices, which ultimately supports and stimulates domestic consumption.
Direct support to the production of specific agricultural products is monitored in the context of this report by the single commodity transfer (SCT) indicator, which takes into account both MPS and coupled payments to single products. On average across all 54 countries covered in this report, SCTs accounted for half of the support provided directly to producers, or USD 247 billion in 2019-21 (USD 362 billion in positive transfers and USD 115 billion in implicit taxation). Support for livestock products, which tend to have high GHG emissions intensities, amounted to USD 111 billion, or 31% of total positive SCTs (Figure 1.9). Transfers to livestock products represent more than 60% of positive SCTs in Iceland, the United Kingdom, Norway, Switzerland, Canada, and the European Union.
Notes: Countries are ranked according to the % SCT levels.
1. EU28 for 2019, EU27 and the United Kingdom for 2020 and EU27 for 2021.
2. The OECD total does not include the non-OECD EU Member States.
3. The 11 emerging economies include Argentina, Brazil, China, India, Indonesia, Kazakhstan, the Philippines, Russian Federation, South Africa, Ukraine and Viet Nam.
4. The All countries total includes all OECD countries, non-OECD EU Member States, and the emerging economies.
Source: OECD (2022), “Producer and Consumer Support Estimates”, OECD Agriculture statistics (database), http://dx.doi.org/10.1787/agr-pcse-data-en.
Among the commodities receiving the highest SCTs, several show particularly high emissions intensities as measured in kgCO2eq per USD of gross farm receipts (Figure 1.10). In particular, three high emission intensity products (in terms of emissions per USD of production value – including receipts), representing 47% of the direct agricultural emissions covered in this report, receive a large volume of positive support: USD 25 billion for beef, USD 7 billion for sheep and goat meat, and USD 44 billion for rice. From a climate perspective, this corresponds to an equivalent transfer of USD 22, USD 31 and USD 115 per tCO2eq, for these three products respectively. This support mostly comes in the form of market price support, however, rather than direct payments to farmers.
Figure 1.10. Emission intensity mapped to single commodity transfers (SCTs)
Copy link to Figure 1.10. Emission intensity mapped to single commodity transfers (SCTs)
Note: Data show single commodity transfers from all 54 countries covered by this report. The dark blue bars correspond to positive SCTs, whereas the light blue bars show negative SCTs.
Source: OECD (2022), “Producer and Consumer Support Estimates”, OECD Agriculture statistics (database), http://dx.doi.org/10.1787/agr-pcse-data-en.
The overall climate impact of SCTs ultimately depends on the specific instruments used. The potentially most environmentally harmful measures are output-based support, including both MPS and output payments, as they increase directly domestic production, as well as input-based payments, except if they improve the competitiveness of low-emission commodities relative to high emission ones or lessen the use of emission-intensive inputs relative to other inputs. At a global level, the widespread use of market price support has more uncertain impacts on overall production and may even lower global emissions, if raising the competitiveness of low emission production systems (Laborde et al., 2021[56]; Guerrero et al., 2022[57]).23 The overall climate impact of reforming MPS will therefore depend on relative differences in emissions intensities in the regions where production is relocated, and the potential productivity gains associated with the reform. Local contexts and accompanying conditions for specific forms of support should also be considered. Nonetheless, SCTs generally provide relatively untargeted transfers to producers, and therefore are not as effective as targeted investments in emission-saving technologies and management practices, or other incentives to accelerate mitigation options (Gautam et al., 2022[58]). Lastly, less distortive forms of SCTs, such as payments based on area or animal numbers, can increase emissions if they favour production of more GHG intensive products, which is often the case with livestock payments. However, when associated with cross-compliance requirements on farm management practices these forms of support may also provide incentives for the adoption of mitigation measures.
Payments based on variable input use
Payments based on variable inputs deserve specific attention, as this category represented USD 60 billion of support in 2019-2021, and a large part is not covered by the SCT category. Variable inputs targeted by this category typically include the use of fertilisers, fossil fuels or irrigation, which are direct GHG emissions sources or a source of extra energy demand, and which can also cause other environmental impacts. Over application of fertilisers and animal manure leads to substantial nitrogen surpluses, which, in addition to nitrous oxide emissions, generates local pollution, damaging freshwater ecosystems, harming invertebrates and fish, and causing acidification and eutrophication, which stimulate the growth of toxic algae and lower oxygen levels in water (hypoxia) (Guerrero, 2018[59]; Sud, 2020[60]). Similarly, irrigation subsidies can generate significant resource overexploitation issues and exacerbate water scarcity in already vulnerable regions (OECD, 2017[61]).
In most countries, there are few restrictions to protect against the over-utilisation of supported inputs, which leaves their GHG emissions impacts unabated. The optimal policy mix for support related to the use of environmentally harmful inputs would be to impose a tax rather than a subsidy to account for the damage they cause to climate, waterways and natural ecosystems (Anderson and Valenzuela, 2021[62]).
Other forms of payments
Other forms of support to producers – non-coupled area payments and other non-commodity specific payments – have a less direct impact on emissions from production and can sometimes provide other forms of environmental and social benefits. Nevertheless, payments based on current land area, even if not directed to specific crops, still create incentives to expand cultivated areas and maintain marginal lands in production. If crop area payments favour arable farming over livestock production, they may induce a shift away from livestock and a reduction in agricultural GHG emissions and nutrient surpluses. Conversely, area payments may increase GHG emissions in countries where crops account for the dominant share of agricultural GHG emissions (Henderson and Lankoski, 2019[55]).
Fully decoupled payments based on non-current crop area (e.g. payments based on historical entitlements or overall farming income) are among the least environmentally harmful support policies (Henderson and Lankoski, 2019[55]). These measures allow farmers to follow market signals in their production decisions, and in some cases, production is not required for farmers to receive support payments. If historical acreage is fixed for payments, then there is no incentive to bring additional land into the sector (Lankoski and Thiem, 2020[63]). However, payments based on historical entitlements could still affect incentives, if farmers expect their current decisions to influence future payments (DeBoe et al., 2020[64]). Moreover, by supplementing farmer incomes and making agriculture more profitable relative to other land uses, decoupled payments could still stifle structural change and hinder the conversion of agricultural land to more sustainable land uses. Ultimately, the climate impact of decoupled payments depends on the type and effectiveness of mandatory management practices and environmental requirements (cross compliance) that accompany payments (DeBoe, 2020[65]).
Reorienting agricultural support towards more decoupled payments and away from the most production distorting forms of support should support reduction of climate impacts and strengthen further the sustainability of production. At the same time, it is important to recognise that agricultural policies can shape the structure and intensity of production over the long term. Decoupling is therefore unlikely to be sufficient on its own, particularly in countries with a high livestock density and intensive production systems (OECD, 2020[66]; Lankoski and Thiem, 2020[63]). In such cases, more targeted measures may be needed to ensure that policies and market prices reflect the negative environmental externalities associated with agricultural production.
Policies that encourage emissions reductions
Payments for environmental and climate services
Agricultural policies can also be designed to generate positive environmental outcomes, by encouraging farmers to provide environmental goods and services such as carbon sequestration, preservation of rural landscapes, resilience to natural disasters, pollination, habitat provision, and control of invasive species. Agri-environmental payments that encourage the use of environmentally friendly inputs or practices (e.g. compliance with fertiliser use restrictions) are potentially among the most environmentally beneficial types of support measures (DeBoe, 2020[65]). This applies in particular to climate change mitigation, which requires very specific management changes and technologies. That said, only USD 1.7 billion of the USD 293 billion per year of budgetary payments to producers in 2019-21 was purely dedicated to the provision of environmental public goods (i.e. payments based on specific non-commodity outputs). Larger support could however have strong direct or indirect impacts on environmental goods, through decoupled payments (see above), for instance in the case of support to organic farming. Cross-compliance associated to direct payments to producers can also bring environmental benefits compensating for the potentially environmentally harmful impacts from subsidies.
Other policies can have positive climate effects. For example, land retirement policies can create incentives for farmers to switch from crop production to permanent pasture or forests, encouraging a contraction of agricultural land and reducing environmental pressures. However, if not well-managed, a contraction of agricultural land resulting from land abandonment can in some instances lead to negative environmental outcomes such as biodiversity loss, increases in invasive species, or a greater risk of wildfire (DeBoe et al., 2020[64]). While reductions in agricultural land use often have beneficial climate effects by enhancing carbon stocks, they can also be accompanied by the intensification of production on remaining land areas, potentially resulting in unintended negative environmental impacts, including extra fertiliser emissions.
This underscores the importance of carefully managing the reform process to account for potential unintended environmental consequences. For example, reductions in market price support can also result in land abandonment and further intensification of production, with potential negative consequences for biodiversity and landscape ecology. Furthermore, agri-environmental schemes could benefit from improvements in their design to better integrate climate mitigation objectives and in the design of mandatory constraints to better deliver environmental improvements (DeBoe, 2020[65]).
Support for agricultural R&D and innovation
Support for agricultural R&D and innovation plays a vital role in helping to mitigate agricultural emissions. There is ample evidence that public investments in agricultural R&D also generate large rates of return (Alston, Pardey and Rao, 2021[67]; Alston et al., 2010[68]; Piesse and Thirtle, 2010[69]). Agricultural R&D is a key driver of productivity growth, which can help to reduce emissions by allowing more food to be produced with the same amount or fewer emissions-intensive inputs (e.g. land, fertilisers, feed). Innovations such as improvements in farm management practices, new crop varieties and livestock breeds, and new digital technologies (e.g. precision agriculture) can reduce the emissions intensity of production (i.e. emissions per unit of output) while mitigating emissions from land use change.
Support for agricultural innovation remains low at just 0.7% of the value of agricultural production for the 54 countries covered in this report (Figure 1.11). In OECD countries as a whole, public spending on agricultural innovation systems is 1.1% of the value of agricultural production, significantly higher than the average for the 11 emerging economies (0.4%). Support for agricultural innovation is highest in Switzerland, Norway and Korea, where it amounts to more than 2% of the value of agricultural production.
Notes: Countries are ranked according to the share of government expenditure on agricultural innovation in the value of agricultural production. “AIS” refers to the Agricultural knowledge and innovation system.
1. EU28 for 2019, EU27 and the United Kingdom for 2020 and EU27 for 2021.
2. The OECD total does not include the non-OECD EU Member States.
3. The 11 emerging economies include Argentina, Brazil, China, India, Indonesia, Kazakhstan, the Philippines, Russian Federation, South Africa, Ukraine and Viet Nam.
4. The All countries total includes all OECD countries, non-OECD EU Member States, and the emerging economies.
Source: OECD (2022), “Producer and Consumer Support Estimates”, OECD Agriculture statistics (database), http://dx.doi.org/10.1787/agr-pcse-data-en.
In some situations at the local level, productivity improvements may trigger increased production and will not necessarily result in lower emissions (see Box 1.3). However, channelling a greater share of R&D spending towards mitigation measures can help to foster sustainable intensification and is more likely to be successful in reducing agricultural emissions. While support for mitigation measures is increasing, there is limited evidence to suggest that it represents an important share of total funding for agricultural R&D and innovation. For example, Australia’s support for the development of innovative livestock feed technologies amounts to USD 23 million over six years, which on an annual basis represents less than 0.4% of total annual spending on agricultural innovation (USD 994 million in 2019-21). Until recently, Canada’s On-Farm Climate Action Fund (USD 160 million for 2021-24) represented just 6% of the agricultural innovation budget (USD 842 million in 2019-21), although new investments have just been proposed. These two examples relate to countries that have relatively high rates of support for agricultural R&D and innovation, suggesting that there is ample scope to further increase funding for R&D focused on the mitigation of agricultural emissions.
Recent studies have shown that using public expenditures on agricultural support to invest in the development and adoption of green innovations (i.e. new technologies that reduce emissions and increase productivity, such as climate smart agriculture) can reduce emissions from agriculture and land use by more than 40%, returning 105 million hectares of agricultural land to habitats (Gautam et al., 2022[58]). Unfortunately, growth in public agricultural R&D investment has been slowing over the past decade in high-income countries (Heisey and Fuglie, 2018[70]). Reversing this trend and increasing support for the development of new technologies and innovations to mitigate agricultural emissions is therefore of critical importance to mitigate agricultural emissions. Agricultural R&D will however require time to deliver its impacts, therefore this policy measure should be used in complement to some other more immediate channels of action.
Conclusion and summary of recommendations: Reforming agricultural policies to address climate change mitigation objectives
Copy link to Conclusion and summary of recommendations: Reforming agricultural policies to address climate change mitigation objectivesAgriculture is a major driver of climate change, both through direct on-farm emissions and indirect emissions from land use change. AFOLU currently accounts for 22% of global anthropogenic GHG emissions; this share is projected to rise as global population growth and rising incomes continue to drive increases in food demand, and as other sectors decarbonise. Respecting the Paris Agreement's commitment of keeping global temperatures within 1.5°C above pre-industrial levels will not be possible without agriculture contributing to global mitigation efforts. The sector has significant potential to reduce its GHG emissions, and is also uniquely positioned to contribute to carbon dioxide removals through carbon sequestration. At the same time, agriculture faces unique challenges as it needs to adapt to a changing climate while providing safe and nutritious food for all and supporting rural incomes and livelihoods.
Greater policy ambition is needed for the mitigation of agricultural GHG emissions
Although agricultural emissions are included in most countries’ NDCs, only 16 out of the 54 countries covered in this report have set some form of mitigation target for their agricultural sector. Some of these countries have included these targets in their NDCs or in national strategic plans, but these are not, in most cases, legally binding. Only a few countries have included agriculture in carbon pricing schemes and environmental regulations are often lagging behind when it comes to the climate dimension. There is therefore considerable scope for policy reforms to intensify and accelerate emission reductions in the sector in support of climate stabilisation.
Existing agricultural support policies contribute to higher emissions
The structure of support to agricultural production has changed little over the past decade and continues to contribute to increasing GHG emissions. Of the USD 611 billion in annual support to individual producers in 2019-21, more than half (USD 361 billion) was provided as positive transfers to specific commodities. This includes market price support (MPS) resulting from policies raising domestic market prices of agricultural products above international market levels, and payments targeted to specific commodities. These support measures provide incentives for additional domestic production, the intensification of input use, and the expansion of agricultural land, which all result in increased domestic GHG emissions (although the effect of market price support on global emissions is likely to be small and potentially negative due to differences in emissions intensities across countries). MPS could therefore be perceived as a way to reduce import-embedded emissions for low-emission intensity countries. However, such an indirect approach has uncertain impacts and is unlikely to be as effective as direct emissions pricing or targeted mitigation investments.
Countries should reduce and reform support targeting emissions-intensive products
The role of support for livestock production is particularly sensitive in this regard. Livestock is responsible for the largest share of agricultural GHG emissions, and is a strong contributor to the methane footprint globally, in particular due to enteric fermentation from ruminants. Support for livestock products, which tend to have high GHG emissions intensities, amounted to USD 111 billion, or 31% of total positive transfers to specific commodities. From a climate perspective, product-specific support is for instance equivalent to a USD 22 per tCO2eq subsidy for beef, and a USD 31 per tCO2eq subsidy for sheep and goat meat. Rice also contributes significantly to emissions compared to other crops, due to methane from flooded areas. Support linked to rice production amounted to USD 44 billion, i.e. USD 115 per tCO2eq. Most of the support above is in the form of MPS which encourages local production and may reduce domestic consumption through higher prices, but does not incentivise investments to reduce emissions. Support to other crops contributes relatively less to climate change impact, but still contributes to higher GHG emissions through fertiliser use. These forms of support should be reduced and reformed, while taking national conditions into consideration, as well as the specifics of policy design.
Subsidies for environmentally harmful inputs should be phased-out
Current policies also directly subsidise the use of variable inputs such as fertilisers, feed and fuel, amounting to USD 60 billion per year in 2019-21. Subsidies for synthetic fertilisers provided without appropriate constraints leads to increased nitrous oxide emissions, and nutrient leaching and runoff causing severe damage to freshwater resources. Subsidies for feed directly incentivise increased livestock production and related GHG emissions, whereas fossil fuel subsidies encourage carbon dioxide emissions from increased on-farm energy use. These inputs should be taxed rather than subsidised when they prove to be environmentally harmful, to account for their negative environmental externalities.
Targeted interventions towards sustainable management and productivity growth are needed
Reducing support for emissions-intensive products and inputs will not be sufficient on its own, and more targeted interventions will be needed for strong emissions abatement. Reducing direct on-farm emissions from agricultural production will require improvements in productivity and the efficiency of input use, greater deployment of new technologies, and improvements in farm management. For many crop producers, this entails improving cultivation practices, increasing the efficiency of fertiliser use, and promoting the use of precision agriculture and integrated crop management. Livestock emissions can be addressed through a combination of improvements in feed conversion efficiencies, better feed and pasture quality, strengthening farm and animal management, as well as methane inhibitors such as feed supplements. Production needs can be decreased by limiting on-farm losses through more resistant crops, improved harvesting equipment and techniques, better storage infrastructure and logistics. On-farm energy consumption can also be reduced by promoting renewable energies and the adoption of greener and more efficient fuels to power agricultural machinery. Agriculture can also help reducing fossil fuels consumption via bioenergy sustainable production.
On the land use change side, there are also several avenues to significantly reduce emissions. Forest protection, coupled with improvements in agricultural productivity, can play an essential role in limiting the expansion of agricultural land and can also create opportunities to sequester carbon by restoring and reforesting marginal lands. Halting and reversing peatland conversion can also be achieved at relatively low cost. Soil carbon sequestration can be achieved through measures such as improved management of crop rotations, residues, vegetation, cattle stocking densities and cropland-pasture integration. Agricultural plantations, agroforestry and afforestation on agricultural land are also promising avenues for carbon sequestration.
Support should transition towards less coupled payments and payments for environmental public goods
Other forms of support to producers, including non-coupled area payments and other non-commodity specific payments, have less of a direct impact on emissions from production, and bring environmental co-benefits through cross-compliance. Nevertheless, payments based on current land area, even if not directed to specific crops, may still create incentives to expand cultivated areas and maintain marginal lands in production. There is large room to make these payments more beneficial for climate action. Payments can be made conditional on the provision of environmental goods and services such as carbon sequestration, afforestation and the restoration and rehabilitation of marginal lands. In 2019-21, only USD 1.7 billion of the USD 293 billion per year of budgetary payments to producers was purely dedicated to the provision of environmental public goods (i.e. payments based on specific non-commodity outputs). Larger support could however have strong direct or indirect impacts on environmental goods, through decoupled payments (e.g. for organic farming). Funding to climate services in agriculture should be ramped up to accelerate the adjustments needed at farm level.
Including agriculture in carbon pricing schemes could incentivise the transition to low-emission agriculture
Mechanisms that put an explicit price on emissions are the most efficient way to minimise the abatement burden for the sector, by recognising the heterogeneity of abatement costs across producers. Carbon pricing options include emissions taxes and emissions trading schemes, carbon offsets, and some abatement subsidies (e.g. delivered via auctions). Participation in voluntary schemes such as carbon offsetting and abatement subsidy programmes are limited by the availability of public and private sector funding to pay producers for emissions reductions. They also require strong transparency and integrity standards to ensure additionality, potentially limiting their scope and effectiveness. Conversely, instruments that apply the “polluter pays” principle such as emissions taxes are among the most effective and efficient policies to mitigate agricultural emissions, but they shift a part of the burden on consumers, which may require accompanying measures.
In spite of their efficiency and use in other sectors, there are currently relatively few examples of countries that have introduced emissions pricing to mitigate agricultural emissions. Agricultural emissions tend to be excluded from most economy-wide carbon taxes and emissions trading schemes, and are often dealt with through other complementary mechanisms. Applying pricing schemes to the agricultural sector could support more ambitious mitigation plans, even if such schemes will need to be adapted to the specific context and constraints related to the sector.
In addition to emissions pricing instruments, stronger environmental regulations and cross compliance can also help to reduce emissions
Strengthening environmental regulations can also accelerate progress on the mitigation of agricultural emissions. For example, governments can link support with measures to prevent additional clearing of forests and expansion of agricultural land. As a good practice, support should not be provided to farmers participating in illegal deforestation or conversion and drainage of peatland. Cross-compliance attached to farm payments could also be used to broaden the adoption of climate-friendly practices in agriculture. Tighter environmental regulations and standards may also be needed in other related policy domains such as water and air quality to foster climate action in agriculture.
Demand-side measures may also be needed, including efforts to reduce the emissions intensity of consumer diets
More sustainable production will help to limit the climate impacts of agriculture but may not be sufficient for the level of transformation needed. Deeper structural change will also have to take place to reduce the carbon footprint of agricultural production, requiring a food systems perspective and adaptation along the supply chain and in demand patterns. This may require changes in consumer behaviour to reduce the consumption of emissions-intensive products, in particular products of animal origin, in countries where per capita protein consumption far exceeds dietary guidelines. Dietary changes could also bring potential co-benefits for consumers in terms of health and nutrition improvements. Lower consumption of livestock products can also reduce deforestation and biodiversity losses due to the expansion of pasture and cropland for feed production. Actions encouraging consumers to limit food waste and overconsumption can also help to mitigate agricultural emissions by reducing the volume of production needed, even though abatement scope is more limited for that route.
Greater support should be provided for R&D and innovation to mitigate climate change
Last, support for agricultural R&D and innovation has a vital role to play in agricultural emissions mitigation. Support for agricultural innovation amounted to only USD 26 billion in 2019-21 and remains low at just 0.7% of the value of agricultural production. While support for mitigation measures has been increasing, its share of total funding for agricultural R&D and innovation remains small. Channelling a greater share of R&D spending towards mitigation measures can help to foster sustainable productivity growth and develop the new technologies needed for low-emissions agriculture. Governments should improve public agricultural R&D funding, create the conditions to attract private investment and facilitate public-private partnerships and international R&D co-operation, with the involvement of farmers and other stakeholders.
Climate action for agriculture should build on synergies and manage potential trade-offs
Considering the urgency of the climate change challenge, agriculture should embrace climate action rapidly. However, optimal policies should take account of wider implications for food systems, exploit synergies with other social and environmental objectives and be balanced in each context with potential adverse impacts. For example, measures aiming to reduce deforestation or limit the use of synthetic fertilisers also result in improvements in biodiversity, soil health and water quality, but may come in conflict with agriculture production needs. Demand-side policies that encourage shifts towards lower emission intensity diets can have potential co-benefits for public health but may also pose a threat to farmers living from livestock production.
The introduction of emissions taxes may imply higher costs for some producers and consumers, and should be accompanied by transitional assistance and targeted transfers to the most vulnerable populations that may be affected by higher food prices. On the other hand, paying farmers to reduce their emissions can ease the impact on producers and consumers, but may also put pressure on public finances unless balanced by a reduction in existing agricultural support. A broader food systems approach is required to address these challenges and to take a holistic view on the performance of climate measures, in light of other multiple policy objectives and implications for the various stakeholders.
As with other food systems issues, effective climate action for agriculture will require collaboration between different policy communities (climate, agriculture, rural development, food security, public health), as well as overcoming barriers related to facts, interests, and values (OECD, 2021[40]). Robust, inclusive, and evidence-based processes are thus essential.
References
[16] Adhya, T. et al. (2014), Wetting and Drying: Reducing Greenhouse Gas Emissions and Saving Water from Rice Production, World Resources Institute, https://files.wri.org/d8/s3fs-public/wetting-drying-reducing-greenhouse-gas-emissions-saving-water-rice-production.pdf.
[68] Alston, J. et al. (2010), A Meta-Analysis of Rates of Return to Agricultural R&D: Ex Pede Herculem?, International Food Policy Research Institute (IFPRI) Research Report 113.
[67] Alston, J., P. Pardey and X. Rao (2021), “Payoffs to a half century of CGIAR research”, American Journal of Agricultural Economics, Vol. 104/2, pp. 502-529, https://doi.org/10.1111/ajae.12255.
[62] Anderson, K. and E. Valenzuela (2021), “What impact are subsidies and trade barriers abroad having on Australasian and Brazilian agriculture?”, Australian Journal of Agricultural and Resource Economics, Vol. 65/2, https://doi.org/10.1111/1467-8489.12413.
[54] Baumol, W. and W. Oates (1988), The theory of environmental policy, Cambridge University Press, Cambridge, https://doi.org/10.1017/cbo9781139173513.
[26] Blandford, D. and K. Hassapoyannes (2018), “The role of agriculture in global GHG mitigation”, OECD Food, Agriculture and Fisheries Papers, No. 112, OECD Publishing, Paris, https://doi.org/10.1787/da017ae2-en.
[41] Burney, J., S. Davis and D. Lobell (2010), “Greenhouse gas mitigation by agricultural intensification”, Proceedings of the National Academy of Sciences, Vol. 107/26, pp. 12052-12057, https://doi.org/10.1073/pnas.0914216107.
[32] Busch, J. and K. Ferretti-Gallon (2017), “What Drives Deforestation and What Stops It? A Meta-Analysis”, Review of Environmental Economics and Policy, Vol. 11/1, pp. 3-23, https://doi.org/10.1093/reep/rew013.
[24] Clark, M. et al. (2020), “Global food system emissions could preclude achieving the 1.5° and 2°C climate change targets”, Science, Vol. 370/6517, pp. 705-708, https://doi.org/10.1126/science.aba7357.
[11] Crippa, M. et al. (2021), “Food systems are responsible for a third of global anthropogenic GHG emissions”, Nature Food, Vol. 2, pp. 198-209, https://doi.org/10.1038/s43016-021-00225-9.
[33] Curtis, P. et al. (2018), “Classifying drivers of global forest loss”, Science, Vol. 361/6407, pp. 1108-1111, https://doi.org/10.1126/science.aau3445.
[31] Dasgupta, P. (2021), The Economics of Biodiversity: The Dasgupta Review. Abridged Version, HM Treasury, London, https://assets.publishing.service.gov.uk/government/uploads/system/uploads/attachment_data/file/957292/Dasgupta_Review_-_Abridged_Version.pdf.
[65] DeBoe, G. (2020), “Impacts of agricultural policies on productivity and sustainability performance in agriculture: A literature review”, OECD Food, Agriculture and Fisheries Papers, No. 141, OECD Publishing, Paris, https://doi.org/10.1787/6bc916e7-en.
[64] DeBoe, G. et al. (2020), “Reforming Agricultural Policies Will Help to Improve Environmental Performance”, EuroChoices, Vol. 19/1, pp. 30-35, https://doi.org/10.1111/1746-692X.12247.
[36] FAO (2022), FAOSTAT Emissions Totals database, Food and Agriculture Organization of the United Nations, Rome, Italy, https://www.fao.org/faostat/en/#data/GT (accessed on 8 March 2022).
[12] FAO (2021), The share of agri-food systems in total greenhouse gas emissions: Global, regional and country trends 1990–2019, Food and Agriculture Organization of the United Nations, Rome, Italy, https://fenixservices.fao.org/faostat/static/documents/EM/cb7514en.pdf.
[45] FAO (2019), The State of Food and Agriculture 2019. Moving forward on food loss and waste reduction, https://www.fao.org/3/ca6030en/ca6030en.pdf.
[20] FAO (2018), The future of food and agriculture – Alternative pathways to 2050, FAO, Rome, https://www.fao.org/3/I8429EN/i8429en.pdf.
[35] FAO and UNEP (2020), The State of the World’s Forests 2020. Forests, biodiversity and people., FAO, Rome, https://doi.org/10.4060/ca8642en.
[38] Fuglie, K. (2015), “Accounting for growth in global agriculture”, Bio-based and Applied Economics, Vol. 4/3, pp. 201-234, https://doi.org/10.13128/BAE-17151.
[58] Gautam, M. et al. (2022), Repurposing Agricultural Policies and Support: Options to Transform Agriculture and Food Systems to Better Serve the Health of People, Economies, and the Planet, World Bank, Washington, DC., https://openknowledge.worldbank.org/handle/10986/36875.
[15] Gerber, P. et al. (2013), Tackling climate change through livestock – A global assessment of emissions and mitigation opportunities, Food and Agriculture Organization of the United Nations (FAO), Rome, https://www.fao.org/3/i3437e/i3437e.pdf.
[44] Grafton, R. et al. (2018), “The paradox of irrigation efficiency”, Science, Vol. 361/6404, pp. 748-750, https://doi.org/10.1126/science.aat9314.
[59] Guerrero, S. (2018), “Farmland Birds under Pressure”, EuroChoices, Vol. 17/3, pp. 24-25, https://doi.org/10.1111/1746-692X.12204.
[57] Guerrero, S. et al. (2022), “The Impacts of Agricultural Trade and Support Policy Reform on Climate Change Adaptation and Environmental Performance: A Model-Based Analysis”, OECD Food, Agriculture and Fisheries Papers, No. 180, OECD Publishing, Paris, https://www.oecd-ilibrary.org/agriculture-and-food/oecd-food-agriculture-and-fisheries-working-papers_18156797.
[70] Heisey, P. and K. Fuglie (2018), “Public agricultural R&D in high-income countries: Old and new roles in a new funding environment”, Global Food Security, Vol. 17, pp. 92-102, https://doi.org/10.1016/j.gfs.2018.03.008.
[53] Henderson, B., C. Frezal and E. Flynn (2020), “A survey of GHG mitigation policies for the agriculture, forestry and other land use sector”, OECD Food, Agriculture and Fisheries Papers 145, https://doi.org/10.1787/59ff2738-en.
[72] Henderson, B. and J. Lankoski (2020), “Assessing the Environmental Impacts of Agricultural Policies”, Applied Economic Perspectives and Policy, pp. 1-16, https://doi.org/10.1002/aepp.13081.
[55] Henderson, B. and J. Lankoski (2019), “Evaluating the environmental impact of agricultural policies”, OECD Food, Agriculture and Fisheries Papers, No. 130, OECD Publishing, Paris, https://doi.org/10.1787/add0f27c-en.
[7] Henderson, B. et al. (2022), “Soil carbon sequestration by agriculture: Policy options”, OECD Food, Agriculture and Fisheries Papers, No. 174, OECD Publishing, Paris, https://doi.org/10.1787/63ef3841-en.
[14] Herrero, M. et al. (2013), “Biomass use, production, feed efficiencies, and greenhouse gas emissions from global livestock systems”, Proceedings of the National Academy of Sciences, Vol. 110/52, pp. 20888-20893, https://doi.org/10.1073/pnas.1308149110.
[43] Hertel, T., N. Ramankutty and U. Baldos (2014), “Global market integration increases likelihood that a future African Green Revolution could increase crop land use and CO2 emissions”, Proceedings of the National Academy of Sciences, Vol. 111/38, pp. 13799-13804, https://doi.org/10.1073/pnas.1403543111.
[50] Hirvonen, K. et al. (2020), “Affordability of the EAT–Lancet reference diet: a global analysis”, The Lancet Global Health, Vol. 8/1, pp. e59-e66, https://doi.org/10.1016/s2214-109x(19)30447-4.
[34] Hosonuma, N. et al. (2012), “An assessment of deforestation and forest degradation drivers in developing countries”, Environmental Research Letters, Vol. 7/4, https://doi.org/10.1088/1748-9326/7/4/044009.
[2] Ignaciuk, A. and D. Mason-D’Croz (2014), “Modelling Adaptation to Climate Change in Agriculture”, OECD Food, Agriculture and Fisheries Papers, No. 70, OECD Publishing, Paris, https://doi.org/10.1787/5jxrclljnbxq-en.
[10] IPCC (2022), Chapter 12: Cross-sectoral perspectives, Working Group III contribution to the Sixth Assessment Report (AR6) of the Intergovernmental Panel on Climate Change (IPCC), https://report.ipcc.ch/ar6wg3/pdf/IPCC_AR6_WGIII_FinalDraft_Chapter12.pdf.
[5] IPCC (2022), Chapter 7: Agriculture, Forestry and Other Land Uses (AFOLU), Working Group III contribution to the Sixth Assessment Report (AR6) of the Intergovernmental Panel on Climate Change (IPCC), https://report.ipcc.ch/ar6wg3/pdf/IPCC_AR6_WGIII_FinalDraft_Chapter07.pdf.
[1] IPCC (2022), Climate Change 2022: Impacts, Adaptation and Vulnerability. Working Group II Contribution to the IPCC Sixth Assessment Report, Cambridge University Press, https://www.ipcc.ch/report/sixth-assessment-report-working-group-ii/.
[30] IPCC (2019), “Summary for Policymakers”, in Climate Change and Land: an IPCC special report on climate change, desertification, land degradation, sustainable land management, food security, and greenhouse gas fluxes in terrestrial ecosystems, Intergovernmental Panel on Climate Change (IPCC), https://www.ipcc.ch/srccl/chapter/summary-for-policymakers/.
[71] IPCC (2019), “Summary for Policymakers”, in Climate Change and Land: an IPCC special report on climate change, desertification, land degradation, sustainable land management, food security, and greenhouse gas fluxes in terrestrial ecosystems, https://www.ipcc.ch/srccl/chapter/summary-for-policymakers/.
[56] Laborde, D. et al. (2021), “Agricultural subsidies and global greenhouse gas emissions”, Nature Communications, Vol. 12/2601, https://doi.org/10.1038/s41467-021-22703-1.
[63] Lankoski, J. and A. Thiem (2020), “Linkages between agricultural policies, productivity and environmental sustainability”, Ecological Economics, Vol. 178, https://doi.org/10.1016/j.ecolecon.2020.106809.
[37] Leifeld, J. and L. Menichetti (2018), “The underappreciated potential of peatlands in global climate change mitigation strategies”, Nature Communications, Vol. 9/1, https://doi.org/10.1038/s41467-018-03406-6.
[28] MacLeod, M. et al. (2015), “Cost-Effectiveness of Greenhouse Gas Mitigation Measures for Agriculture: A Literature Review”, OECD Food, Agriculture and Fisheries Papers, No. 89, OECD Publishing, Paris, https://doi.org/10.1787/5jrvvkq900vj-en.
[6] Minx, J. et al. (2021), “A comprehensive and synthetic dataset for global, regional, and national greenhouse gas emissions by sector 1970–2018 with an extension to 2019”, Earth System Science Data, Vol. 13/11, pp. 5213-5252, https://doi.org/10.5194/essd-13-5213-2021.
[40] OECD (2021), Making Better Policies for Food Systems, OECD Publishing, Paris, https://doi.org/10.1787/ddfba4de-en.
[66] OECD (2020), “Exploring the Linkages between Agricultural Policies, Productivity and Environmental Sustainability”, COM/TAD/CA/ENV/EPOC(2019)4/FINAL, https://one.oecd.org/document/COM/TAD/CA/ENV/EPOC(2019)4/FINAL/en/pdf.
[3] OECD (2020), Strengthening Agricultural Resilience in the Face of Multiple Risks, OECD Publishing, Paris, https://doi.org/10.1787/2250453e-en.
[25] OECD (2019), Enhancing Climate Change Mitigation through Agriculture, OECD Publishing, Paris, https://doi.org/10.1787/e9a79226-en.
[61] OECD (2017), Water Risk Hotspots for Agriculture, OECD Studies on Water, OECD Publishing, Paris, https://doi.org/10.1787/9789264279551-en.
[21] OECD (2016), Alternative Futures for Global Food and Agriculture, OECD Publishing, Paris, https://doi.org/10.1787/9789264247826-en.
[52] OECD (2008), Biofuel Support Policies: An Economic Assessment, OECD Publishing, Paris, https://doi.org/10.1787/9789264050112-en.
[9] OECD.Stat (2021), Agri-environmental indicators, https://stats.oecd.org/# (accessed on 9 March 2022).
[19] OECD/FAO (2022), “OECD Agriculture statistics (database)”, OECD-FAO Agricultural Outlook, https://doi.org/10.1787/agr-outl-data-en.
[18] OECD/FAO (2022), OECD-FAO Agricultural Outlook 2022-2031, OECD Publishing, Paris.
[4] OECD/FAO (2021), Building Agricultural Resilience to Natural Hazard-induced Disasters: Insights from Country Case Studies, OECD Publishing, Paris, https://doi.org/10.1787/49eefdd7-en.
[8] OECD/FAO (2021), “OECD Agriculture statistics (database)”, OECD-FAO Agricultural Outlook, https://doi.org/10.1787/agr-outl-data-en (accessed on 10 March 2022).
[17] Parker, R. et al. (2018), “Fuel use and greenhouse gas emissions of world fisheries”, Nature Climate Change, Vol. 8, pp. 333-337, https://doi.org/10.1038/s41558-018-0117-x.
[69] Piesse, J. and C. Thirtle (2010), “Agricultural R&D, technology and productivity”, Philosophical transactions of the Royal Society B, Vol. 365/1554, pp. 3035-3047, https://doi.org/10.1098/rstb.2010.0140.
[13] Poore, J. and T. Nemecek (2018), “Reducing food’s environmental impacts through producers and consumers”, Science, Vol. 360/6392, pp. 987-992, https://doi.org/10.1126/science.aaq0216.
[22] Popp, A. et al. (2017), “Land-use futures in the shared socio-economic pathways”, Global Environmental Change, Vol. 42, pp. 331-345, https://doi.org/10.1016/j.gloenvcha.2016.10.002.
[47] Popp, A., H. Lotze-Campen and B. Bodirsky (2010), “Food consumption, diet shifts and associated non-CO2 greenhouse gases from agricultural production”, Global Environmental Change, Vol. 20/3, pp. 451-462, https://doi.org/10.1016/j.gloenvcha.2010.02.001.
[29] Reisinger, A. et al. (2021), “How necessary and feasible are reductions of methane emissions from livestock to support stringent temperature goals?”, Philosophical Transactions of the Royal Society A: Mathematical, Physical and Engineering Sciences, Vol. 379/2210, p. 20200452, https://doi.org/10.1098/rsta.2020.0452.
[23] Springmann, M. et al. (2018), “Options for keeping the food system within environmental limits”, Nature, Vol. 562/7728, pp. 519-525, https://doi.org/10.1038/s41586-018-0594-0.
[46] Stehfest, E. et al. (2009), “Climate benefits of changing diet”, Climatic Change, Vol. 95/1-2, pp. 83-102, https://doi.org/10.1007/s10584-008-9534-6.
[60] Sud, M. (2020), “Managing the Biodiversity Impacts of Fertiliser and Pesticide Use: Overview and insights from trends and policies across selected OECD countries”, OECD Environment Working Papers, No. 155, OECD Publishing, Paris, https://doi.org/10.1787/63942249-en.
[48] Tilman, D. and M. Clark (2014), “Global diets link environmental sustainability and human health”, Nature, Vol. 515/7528, pp. 518-522, https://doi.org/10.1038/nature13959.
[51] UNEP (2021), Food Waste Index Report 2021, United Nations Environment Programme, https://www.unep.org/resources/report/unep-food-waste-index-report-2021.
[39] USDA (2021), International Agricultural Productivity statistics (October 2021 update), USDA Economic Research Service (ERS), https://www.ers.usda.gov/data-products/international-agricultural-productivity/.
[42] Villoria, N. (2019), “Technology Spillovers and Land Use Change: Empirical Evidence from Global Agriculture”, American Journal of Agricultural Economics, Vol. 101/3, pp. 870-893, https://doi.org/10.1093/ajae/aay088.
[27] Wassmann, R., Y. Hosen and K. Sumfleth (2009), Reducing Methane Emissions from Irrigated Rice, International Food Policy Research Institute (IFPRI), https://ebrary.ifpri.org/utils/getfile/collection/p15738coll2/id/28232/filename/28233.pdf.
[49] Willett, W. et al. (2019), “Food in the Anthropocene: the EAT–Lancet Commission on healthy diets from sustainable food systems”, The Lancet, Vol. 393/10170, pp. 447-492, https://doi.org/10.1016/s0140-6736(18)31788-4.
Notes
Copy link to Notes← 1. The agriculture category from UNFCCC inventories covers only the non-CO2 emissions associated with agricultural production and some small CO2 sources related to soil improvements. Fossil-fuel emissions generated on the farm are accounted as part of the “Energy” sector. Changes in carbon stocks in agricultural soils are accounted as part of the LULUCF category.
← 2. LULUCF corresponds to the land use and land use change part of AFOLU, therefore AFOLU = agriculture + LULUCF.
← 3. Emissions from AFOLU in 2010-19 averaged 11.9 ± 4.4 billion tonnes carbon dioxide equivalent (GtCO2eq), out of a total of 56.3 ± 6.1 GtCO2eq, per year. In 2019, AFOLU emissions amounted to 12.8 ± 5.0 GtCO2eq out of a total of 58.6 GtCO2eq with IPCC AR6 GWP100 for CH4 and N2O (IPCC, 2022[5]; Minx et al., 2021[6]).
← 4. 5.9 ± 4.1 GtCO2eq per year.
← 5. 6.0 ± 1.7 GtCO2eq per year for the IPCC agriculture category (which excludes on-farm energy consumption).
← 6. On average, agriculture generates 4.2 ± 1.3 GtCO2eq per year in methane emissions and 1.8 ± 1.1 GtCO2eq per year in nitrous oxide emissions.
← 7. Global Warming Potentials (GWPs) are used to convert GHGs to carbon dioxide equivalent (CO2eq), providing a common scale to measure the climate impacts of individual GHGs. Carbon dioxide is the reference and has a GWP of 1 for all time periods. Methane from non-fossil sources such as agriculture is estimated to have a global warming potential (GWP100) of 27.0 ± 11, meaning that one tonne of methane emissions will absorb 27 times more energy over a 100-year period than one tonne of carbon dioxide. Nitrous oxide has a GWP100 of 273 ± 130 times that of carbon dioxide for a 100-year timescale.
← 8. Average annual non-CO2 emissions from agriculture have risen from 5.2 ± 1.4 GtCO2eq during 1990-99, to 6.0 ± 1.7 GtCO2eq for the period 2010-19 (using IPCC AR6 GWP100 values to aggregate CH4 and N2O emissions to CO2eq) (IPCC, 2022[5]). Non-CO2 LULUCF emissions are estimated to account 0.6 GtCO2eq and are accounted separately in IPCC (2022[5]).
← 9. Or 4.1 GtCO2eq.
← 10. The share of livestock emissions associated with enteric fermentation, manure management and manure deposited on grassland accounts for 67% of direct agricultural emissions over the 54 countries covered in this report, based on IPCC AR6 GWP100 for methane and nitrous oxide. When adding manure applied to cropland as organic fertiliser, this emissions share rises to 70% (FAO, 2022[36]).
← 11. Earlier estimates from (IPCC, 2019[71]) put global food systems emissions at between 10.8 and 19.1 GtCO2eq per year, or 21-37% of total anthropogenic emissions. According to (Poore and Nemecek, 2018[13]), food systems generate 26% of anthropogenic GHG emissions; this rises to 31% if non-food agriculture and other drivers of deforestation are included. (Crippa et al., 2021[11]) found that food systems contributed 34% to total anthropogenic GHG emissions in 2015, while (FAO, 2021[12]) estimated total emissions from food systems at 16.5 GtCO2eq, or 31% of global emissions in 2019.
← 12. At 1.2 kg CO2eq/USD and 1.1 kg CO2eq/USD respectively.
← 13. At 1.2 t CO2eq/ha and 1.5 t CO2eq/ha respectively.
← 14. Estimates are based on a comprehensive meta-analysis of 1 530 studies covering more than 38 000 commercial farms in 119 countries.
← 15. Beef originating from beef herds generates 90 kg CO2eq per kg of product and 45 kg CO2eq per 100g of protein. Lamb and mutton generates 34 kg CO2eq per kg of product and 17 kg CO2eq per 100g of protein.
← 16. 30 kg CO2eq per kg of product and 15 kg CO2eq per 100g of protein.
← 17. Rice generates emissions of 3.9 kg CO2eq per kg of product. Most grains generate less than 3 kg CO2eq per kg of product and per 100g of protein, while most fruits, vegetables, roots and tubers have average emissions of less than 1 kg CO2eq per kg of product.
← 18. In these examples, highest emissions refers to the 90th percentile, while lowest refers to the 10th percentile. The highest emissions from beef reach 188 kg CO2eq per kg, compared to 34 kg CO2eq per kg for the lowest within beef herds and 16 kg CO2eq per kg for the lowest within dairy herds.
← 19. The lower bound corresponds to the average results based on Integrated Assessment Models (IAMs). These models have the advantage of capturing the possible combinations between options in a structurally consistent framework, but they do not capture all the range of mitigation options analysed by sectoral models.
← 20. Technical potentials can be as high as 8 GtCO2eq for dietary changes and 5.8 GtCO2eq per year for food loss and waste reduction, according to IPCC (2022[5]). Considering there are strong overlaps between these two mitigation options, acting on the same sources, we do not sum up here the two estimates but only present the highest.
← 21. For instance, in the case of beef originated from beef herds, 25% of production with the largest emissions intensities represent 56% of the sector’s emissions and 61% of land use (Poore and Nemecek, 2018[13]).
← 22. This is also true for other forms of environmental policies. Studies have shown that most distortive agricultural support is also the most environmentally harmful from a nitrogen pollution perspective (Henderson and Lankoski, 2019[55]; Henderson and Lankoski, 2020[72]; OECD, 2020[66]; DeBoe, 2020[65]), or for biodiversity (DeBoe, 2020[65]; Lankoski and Thiem, 2020[63]).
← 23. Laborde et al. (2021[56]) estimate that removing domestic support and border measures would increase direct agricultural emissions by 1.7%. Guerrero et al. (2022[57]) finds in a similar assessment accounting for the full AFOLU that such policy change would generate an emission increase of 0.5%.