This chapter presents sixteen case studies submitted by nine OECD member states. They are summarised and include the policy issues raised by the authors of the case studies. This list of case studies provides an insight into the extent to which hybrid technologies, involving more than one single technology, will be important in the net-zero carbon landscape later this century.
Carbon Management: Bioeconomy and Beyond
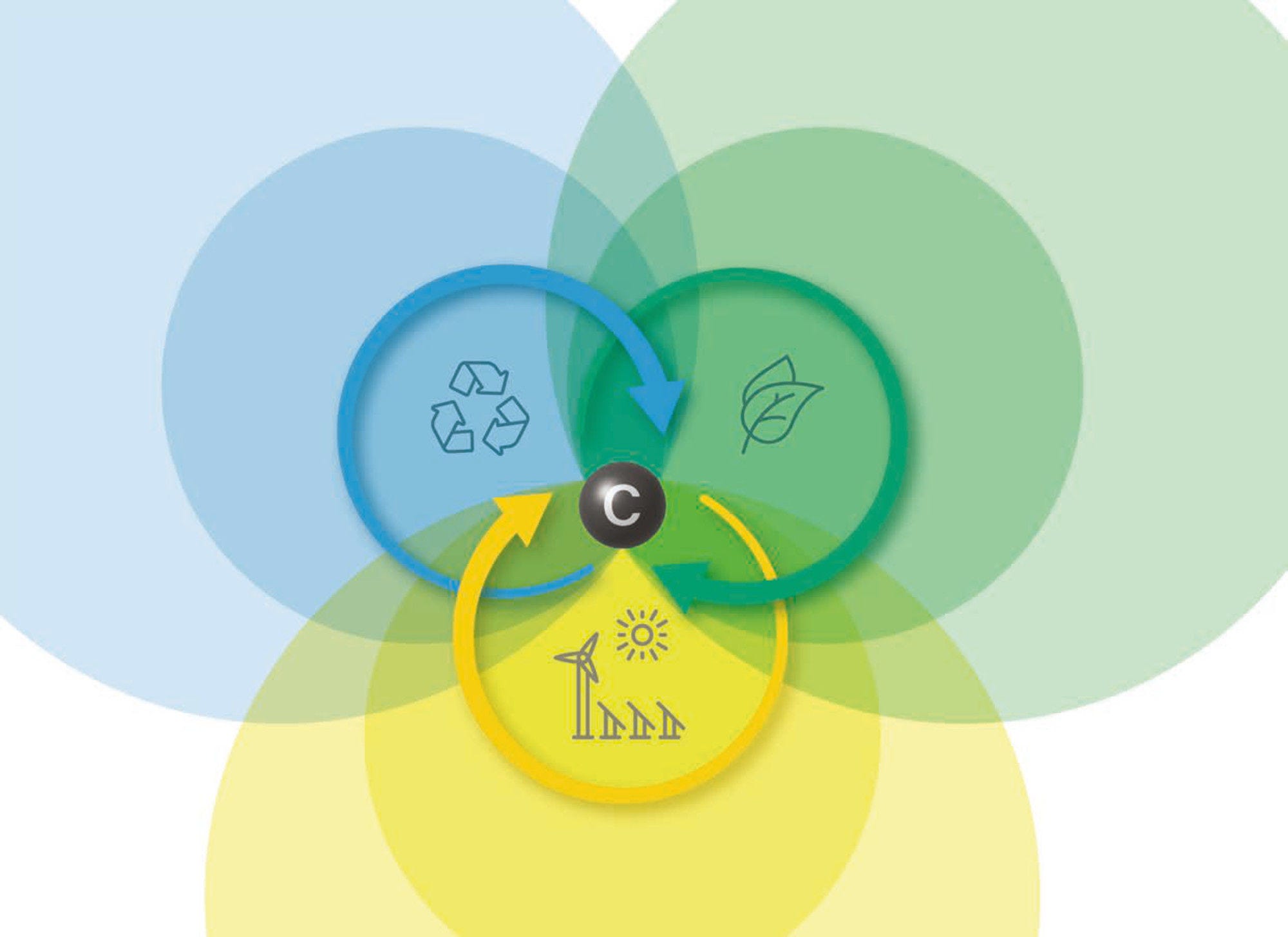
5. Case study summaries and their main policy points
Abstract
The table below summarises the sixteen case studies submitted by nine OECD member states.
Table 5.1. Case studies of carbon management technologies
Country |
Case study |
Main technology |
Convergence? |
---|---|---|---|
Austria |
Carbon2Product Austria (C2PAT): capturing and using CO2 from cement production |
Nanotechnology |
Chemistry, photovoltaics |
Canada |
Brock Commons timber-framed high-rise building |
Construction materials |
Chemistry, wood technology |
Toundra Greenhouse - Turning waste and emissions into food with the circular bioeconomy |
Bioenergy with carbon capture and utilisation (BECCU) |
Waste heat capture, digital, water recycling |
|
Innovation challenges as funding mechanisms to accelerate emerging CCUS technologies |
Funding mechanisms for any CCUS technology |
Convergence is not specifically targeted but is not excluded |
|
Solid recovered fuels and CCUS in industry |
Waste-to-energy facilities that use SRFs with CCUS technologies e.g., gasification |
Waste recycling |
|
Germany |
ZeroCarbon FootPrint (ZeroCarbFP): Conversion of carbon-rich waste streams for a sustainable, biological synthesis of valuable substances |
Biotechnology, chemistry |
Waste recycling |
Italy |
Compostable bioplastics value chain |
Biotechnology, chemistry |
Industrial composting |
PlaCE: (Offshore) Platform conversion for eco-sustainable multiple uses |
Offshore rig decommissioning, mineral accretion, aquaculture, solar energy |
Life-Cycle Cost-Benefit, CCUS, renewable energy e.g., hydrogen production / storage |
|
Japan |
Carbon capture and utilisation by Saga City incineration plant |
Chemistry |
Renewable energy, incineration |
Recycle system development of municipal and industrial waste to useful raw chemicals |
Biotechnology |
Chemistry, gasification |
|
Korea |
Sustainable chemicals and fuels production using nanotechnology in Korea |
Nanotechnology |
CCU, chemistry |
Norway |
Production of fish feed by gas fermentation |
Biotechnology |
Aquaculture, CO2 capture, water electrolysis for hydrogen production |
Decarbonisation strategies of a ferrosilicon plant |
Biotechnology |
CCU, CCS, chemistry |
|
United Kingdom |
Captured CO2 for new fertilizers |
Chemistry, biochemistry |
Agricultural technology |
United States |
Digital agriculture: soil organic carbon networked measurements technologies |
Digital, ICT |
Agricultural technology |
Hybrid technologies for recycling waste carbon using gas fermentation and upgrading |
Biotechnology |
Catalysis, bioenergy |
Several other observations are worth noting that are not implicit from the case study summaries.
Some of the case studies address the industries that are considered to be the hardest to abate, in particular chemicals, cement, steel, aviation.
Renewable energy is frequently used as the energy source. In particular, several of the countries that submitted case studies are planning for a hydrogen economy to fuel these technologies. This net-zero carbon future will go hand in hand with hydrogen, resulting in the need for high levels of investment in both manufacturing and energy technologies. While energy and transportation policy is relatively mature, this is not the case for hydrogen or the other sectors highlighted in the case studies.
Biomass features in the majority of the case studies. One of the case studies concerns funding mechanisms and is exempt from this calculation. Of the fifteen other case studies, thirteen either directly or indirectly involve biomass, but are not necessarily biotechnology-based projects. Examples are the use of wood in high-rise building construction (direct) and digital agriculture (indirect). This is highlighted because some of the most controversial policy issues raised in this report arise from biomass utilisation and its connection to land use, perhaps the greatest source of policy dilemmas and trade-offs. The use of other feedstocks like waste gases and chemically recycled plastics relieve pressure on land but have their own policy issues.
The policy issues that are raised are many, and most are familiar to innovation policy makers. In many cases, the technologies are designed to replace an existing technology, usually of fossil or mineral origin, that is very well established in the market. The driving force is sustainability more generally, and more specifically reduction of emissions. As described elsewhere, that creates dilemmas for policy makers.
Soil as a resource is often forgotten or under-valued, and yet, as mentioned, land use is the most controversial and complex of policy issues. Several of the case studies have an emphasis on improving soil quality, recognising its place as the resource most important to the existence of humans, as without it there is virtually no food. Soil is also an enormously important carbon sink but also, due to human exploitation practices, can contribute to a large proportion of global warming potential.
Austria: Carbon2Product Austria (C2PAT)
Summary
In the cement industry, a large proportion of CO2 emissions cannot be avoided. As it is crucial to find ways to reduce the emissions form the cement industries, the Carbon2Product Austria (C2PAT) project proposes a novel pathway for the utilisation of unavoidable CO2 emissions. By capturing CO2 from cement production, processing that CO2 and using it as a resource, the project aims at demonstrating a first-of-kind, cross-sectoral carbon value cycle at industrial scale and promoting climate neutrality in Austria.
The overall system is based on the integration and joint operation of different technologies that will be combined into one novel hybrid technology and a holistic value cycle. A facility cluster will be installed by 2025 at the site of Lafarge’s cement plant in Mannersdorf. This cluster will comprise a carbon capture unit, a new photovoltaic (PV) park operated by VERBUND, water electrolysis for the production of green hydrogen, a new synthesis route using reverse water-gas shift reaction (RWGS) and a low-temperature Fischer-Tropsch synthesis unit. Intermediates will be processed at OMV/Borealis sites into olefins and ultimately renewable-based plastics. The demonstration plant will also provide important technical insights and information that will ultimately lead to the construction of an industrial full-scale plant by 2030.
Public intervention and supporting policies
In order to address potential sustainability dilemmas and to bring the C2PAT business case and similar ones to fruition, a sustainability framework is needed. This framework has to consist of a combination of different building blocks. On the one hand, these building blocks have to address the supply side: quotas for the share of CO2-neutral products offered or produced are needed. Sustainable and long-term incentives and mechanisms are needed to keep the products constantly in the market. These could include e.g., CO2 trading certificates or adequate CO2 pricing that really covers external effects. Moreover, LCAs should be made mandatory for certain products to capture all impacts throughout their life cycle and enable communication of the results accordingly.
Regarding green energy and hydrogen technologies, there is a need for public funding to address potential environmental sustainability dilemmas e.g., land-use change. Additionally, it is essential to establish global sustainability standards for energy and hydrogen production incorporating the SDGs. These standards will create a common, international understanding and should provide a basis for creating international regulations for the import of green energy and hydrogen for the future electrification of different sectors. As a final important component, to assure the successful implementation of CCUS technologies, the creation of regulatory measures is needed, which must enable competitiveness against fossil-based products/materials, economic viability and the adoption of new energy systems.
On the other hand, there are certain elements that are necessary for the demand side: there is definitely a need for regulations as well as incentives to push the demand side, such as carbon footprint labelling, tax reductions or credit schemes. The trend that customers are increasingly willing to spend more money on CO2-neutral products can be supportive in this regard. However, this positive attitude on the part of customers is not always the case: measures are clearly needed to increase public acceptance. Public discussions need to be initiated that address potential environmental sustainability dilemmas and increase the understanding for higher-priced CO2-neutral products, corresponding innovations and required behavioural changes in consumption and lifestyle. The C2PAT project will also contribute to building up this necessary framework for CCU and CCS technologies. There will be a ‘regulatory group’ in the project, which will develop practical requirements and actions for Austria, but also for Europe, on the basis of the demonstration plant.
Canada 1: Embedding carbon in the built environment
Summary
The University of British Columbia (UBC) Brock Commons student residence building is an 18-storey wood hybrid building built in 2013. The building is 17 storeys of mass-timber built on a one-storey concrete podium, with two concrete staircases, and cost CAD 40.5 million to build. Each floor is made of five-ply cross-laminated timber (CLT) with the building columns made of glue-laminated timber (GLT). Some 2 233 m3 of Canadian timber was used to construct the building, saving approximately 2 432 tonnes of CO2 from being emitted, with 1 753 tonnes of CO2 sequestered in the timber itself, and 679 tonnes of CO2 avoided in substituting traditional construction materials with low-carbon wood materials. It was built in 70 days, approximately four months faster than projects of similar size using traditional construction materials.
Public intervention and supporting policies
The project was supported with funding from the Canadian federal government under Natural Resources Canada’s Tall Wood Building Demonstration Initiative1, as well as funding from the provincial government of British Columbia.
The building was designed to maximise fire protection for occupants, a common concern regarding mass timber buildings. It was also designed to meet the Leadership in Energy and Environmental Design (LEED) gold certification, a sustainability accreditation system that recognises environmental stewardship in building design and operations. Mass timber buildings are expected to provide new socio-economic opportunities across the supply chain, from harvesting to manufacturing facilities, as well as forest-based communities.
Canada 2: Using innovation challenges as funding mechanisms to accelerate emerging CCUS technologies in Canada
Summary
CCUS technologies are relatively nascent with few industrial scale commercial plants in operation today. While private-public partnerships have been effective in the development and demonstration of some flagship CCUS projects in Canada, significant research and capital is still required to help de-risk these new technologies for private investors. To help address investor hesitancy in CCUS technologies, recent innovation models centred on ‘Challenges’ and ‘Prizes’ have become popular among both private and public organisations to help spur innovation. These innovation challenges typically put forth a broad technological challenge or goal to the public with the best technological advance awarded prize money. Typically, these challenges are open to academics, start-ups, and SMEs that have developed new technologies that could address the challenge.
Public intervention and supporting policies
To date, three CCUS innovation challenges have been made available in Canada by both private and public actors: the USD 20 million Carbon XPRIZE2 by Natural Resources Group and Canada’s Oil Sands Innovation Alliance (COSIA), the CAD 35 million Emission Reduction Alberta (ERA) Grand Challenge3 by Emissions Reduction Alberta, and the CAD 13 million Sky’s the Limit Challenge4 by Natural Resources Canada. All three competitions have been part of a broader initiative to support the development, scale-up and commercialisation of CCUS technologies in Canada.
CCUS potential, and the speed of its deployment, will likely still require some government funding and intervention to further de-risk CCUS technologies. However, innovation challenges can also do this, limiting the need for government funding and still accelerating technological development and deployment. In addition, supporting government frameworks, strategies or policies, like Canada’s CCUS strategy that is currently in development, can send important investment signals to private investors and help further de-risk investment in new and emerging technology.
Canada 3: Turning waste and emissions into food with the circular bioeconomy
Summary
The Toundra Greenhouse in Saint-Félicien, Quebec is a 27.5 hectare greenhouse complex. It uses bioenergy carbon capture and utilisation (BECCU) technology to capture up to 30 metric tonnes of CO2 per day (11 000 tonnes of CO2 annually) from the neighbouring sawmill’s cogeneration heat and power (CHP) plant. The captured carbon is transferred directly to the greenhouse to grow upwards of 45 million cucumbers per year. The greenhouse also captures waste heat from the neighbouring CHP plant to heat the greenhouse throughout the year and has a specially built lagoon to capture rainwater and snow melt to water the cucumbers throughout the year.
The greenhouse was built in 2016, and expanded in 2020, costing CAD 100 million dollars. The greenhouse was part of an initiative to improve food security in the region as well as improve the circularity of the CHP plant. The greenhouse also provides important socio-economic benefits to the region by providing employment opportunities, with more than 100 people currently employed to maintain and operate the greenhouse, as well as improving the resilience of the local supply chain.
Public intervention and supporting policies
The project was supported with CAD 3 million in funding from the Canadian federal government and CAD 5 million in funding from the Quebec provincial government. It supports decarbonising Canada’s natural resource sector while providing new economic opportunities for rural communities in Canada.
Canada 4: Using Solid Recovered Fuels and CCUS in Industry
Summary
Solid recovered fuel (SRF) is biogenic (e.g., scrap wood, paper) and non-biogenic (e.g., plastic) feedstock sourced and sorted from non-hazardous municipal solid waste (MSW) and used as a feedstock in various applications. More specifically, SRFs can be used in processes such as gasification to produce energy and chemicals, with the added benefit of improving waste management, especially in large urban areas. In addition, unlike unsorted MSW used in waste-to-energy facilities, which can include contaminants requiring specialised treatment, SRFs can be used in both pre-existing waste-to-energy facilities, as well as less capital-intensive facilities that do not require extensive flue gas treatment because it is a standardised, non-hazardous feedstock. Furthermore, pairing waste-to-energy facilities that use SRFs as a feedstock with CCUS technologies could help to achieve multiple sustainability objectives, such as improving waste and plastics management, while simultaneously improving/reducing the life cycle emissions associated with energy, fuels and/or chemicals production.
Another application of SRFs in current infrastructure includes using them for controlled combustion and displacing fossil-based fuels to reduce emissions in high emissions industries. For example, using SRFs as an alternative fuel paired with CCUS technology in cement kilns could help improve waste management in selected regions while significantly reducing the GHG emissions in the cement industry. Pairing waste-to-energy facilities and heavy industry that use SRFs with CCUS technologies could help make some industries or sectors net-neutral or net-negative, improving the overall carbon management of a number of industrial sectors.
Public intervention and supporting policies
The potential for such a set of processes to make a significant impact is dependent upon several highly variable factors, including sufficient funds for innovation in CCUS, emerging policies to drive the use of alternative feedstocks, and public support for waste-to-energy facilities. In addition, technology for waste-to-energy facilities may need to be de-risked further to incentivise further private investment.
Germany: ZeroCarbon Footprint (ZeroCarbFP)
Summary
By developing innovative products and systems with high potential for added value, the change from a fossil to a bio-based industry is promoted. One of the selected innovation alliances to boost this is ZeroCarbon FootPrint (ZeroCarbFP). ZeroCarbFP aims for the conversion of carbon-rich waste streams into valuable products and thus contributes to a sustainable carbon management. The use of alternative raw materials supports the greater independence from fossil feedstock by having a positive effect on cost structures and competitiveness. Thereby, the alliance takes up a central innovation topic for politics, economy and society, like protection of arable land for food production, the sustainable use of waste streams as a source for carbon, closing of cycles (cradle-to-cradle) by the circular economy. Furthermore, the cross-alliance doctorate programme UfIB - Bioeconomy Initiative Promoting Implementation will strengthen the next generation of scientists and link academia and industry in the development of sustainable production routes.
The sub-programme 1 Bioplastics of the ZeroCarbFP alliance is highlighted. In this sub-programme CO2 as sole carbon source for the production of mono- and dicarboxylic acids as precursors for biopolymers (focus: succinic acid as monomer for biodegradable bioplastics) is addressed. In this sub-programme, microbial fixation of CO2 is researched. A two-stage fermentation has been developed which converts CO2 and hydrogen gas into acetate and succinic acid. The process can be coupled to an existing bioethanol plant, capturing the emitted CO2 from fermentation. This eliminates the need to compress and transport CO2 and enables the cascading use of CO2. The hydrogen can be produced on-site by electrolysis powered by renewable electricity. Using waste and side streams adequately as a starting material leads to an overall higher material efficiency, reduces the dependency on critical raw materials and increase the flexibility of production sites.
Public intervention and supporting policies
The projects under ZeroCarbFP are funded by the German Federal Ministry of Education and Research (BMBF). Initially, ZeroCarbFP had a total budget of EUR 48 million and a time horizon of nine years, which was split into a research, a development and a piloting phase. The programme started in July 2013 and is planned to finish in March 2024.
Italy 1: Bio-based, biodegradable and compostable bioplastics for organic recycling and the creation of compost
Summary
Organic waste can be a valuable source of nutrition for soils. The development of a biodegradable and compostable plastic value chain (products such as bags, packaging, or cutlery) together with an efficient system for biowaste collection and recycling, can create a mixed waste suitable for organic recycling and for the creation of high-quality compost, to be used as a soil improver. The case of the city of Milan is a key example of the efficiencies that biodegradable and compostable materials can provide in the waste management system.
This case study is based on bioplastics that, other than being biodegradable and compostable, are also produced from renewable resources. This contributes to EU climate ambitions: by reducing emissions; by replacing fossil-based materials and energy with bio-based ones, notably biomaterials, and biochemicals; and by facilitating the use of compost in agriculture. It also prevents organic waste from ending up in a landfill, a practice that will be prohibited in the European Union from January 2024. The construction of integrated industrial and agricultural value chains is one of the central elements of the model to promote the sustainable use of biomass, contributing to soil protection and health.
Public intervention and supporting policies
The project is coherent with the main EU and Italian legislative acts related to sustainability and to the promotion of circular bioeconomy and biodiversity protection, such as: the European Bioeconomy Strategy5; the Italian Bioeconomy strategy; the new Waste Directive6; the Green New Deal7; the Farm to Fork8 strategy, the Next Generation Europe and the Italian Recovery and Resilience Plan (PNRR); and the latest Best Available Techniques (BAT) and the new regulation on fertilizers.
Also required is promoting incentives within the Common Agricultural Policy (CAP) and the Climate Change and Horizon Future agreements for the return of resources to agriculture, for the maintenance of soil quality and fertility, decarbonisation and the use of biodegradable products.
Italy 2: PON-Platform Conversion for Eco-Sustainable Multiple Uses (PlaCE)
Summary
Decommissioning of offshore oil and gas infrastructures involves removal of large tonnages of materials to be dismantled and recycled. Rather than remove them completely, offshore oil structures can be used to support marine communities, in some cases of regional significance. Materials, structures, power connection to shore could be instead reused and become an advantage for new installations rather than waste. In this context, an experiment in reusing an offshore platform destined to be decommissioned started in 2020, funded by the PON-FESR project ‘PlaCE: Platform Conversion for Eco-Sustainable Multiple Uses’9 and is still ongoing. The platform is Viviana 1, operated by Eni Spa, placed offshore of Pineto in a 21-metre water depth, in front of the Abruzzo Region, at the Central-East side of Italy.
The scope of the project is to demonstrate the added value of the multiple uses of marine areas and to promote its replicability starting from the reuse of existing platforms. Based on the local climate conditions, the selected reuse solutions are a set of innovative eco-sustainable strategies for offshore aquaculture, solar panels for power independence of the platform activities and the life extension of the offshore infrastructure based on mineral accretion technology under low voltage electrolysis to protect the platform’s steel jackets from corrosion. Besides the field activities, the demonstration project prompts the optimisation of marine renewable energy integration, the set-up of business models and the replicability in other sites to extend the range of economic activities. Overall, the case study boosts the blue circular economy by cost-sharing technologies and logistics and by reusing existing structures.
Public intervention and supporting policies
Japan 1: Recycle system development of municipal and industrial waste to useful raw chemicals
Summary
This case study concerns a system of waste treatment to useful chemicals developed by Sekisui Chemical Co using a microorganism by LanzaTech. Various kinds of waste generated from homes and factories are used as carbon feedstocks. They are gasified to generate hydrogen and carbon monoxide (CO), and then converted to ethanol using anaerobic fermentation technology. The produced ethanol can be used as fuels or solvents or can be used as raw material for ethylene and produce polyethylene or used in various chemical industry fields. Between 2014 and 2018, a pilot study to prove the concept was carried out by running the facility of 10 000 litres per year from gasified waste. Currently, a demonstration facility capable of treating 20 tonnes per day of waste is under construction for operation to start in 2022. In the future, a vision to realise a circular ecosystem on a community-by-community basis, aiming for processing capable of 200 tonnes per day or more (equivalent to the waste generated by 200 000 people per day), will be constructed in Japan and elsewhere.
Public intervention and supporting policies
It is necessary to support the demonstration of feasible implementation at the municipal level in terms of cost and operation. In addition, when the facility is installed at multiple locations, it is necessary to provide an education programme for operations. The cost competitiveness is currently weak against fossil resource-derived products. Therefore, direct support such as subsidies, taxation such as carbon pricing, fossil usage restrictions, are useful. Indirect support from multiple perspectives such as regulatory aspects and standards such as ISO and green labels should be effective. It is also useful to expand the use of private sector vitality for public works projects using private finance initiatives or other monetary mechanisms to address the issues of local governments.
Japan 2: Efforts of CCU by the Saga City cleaning plant
Summary
Saga City is the first city in Japan to separate and recover CO2 from part of the exhaust gas generated during waste incineration, and to use it in the CCU project to cultivate microalgae that produce raw materials for cosmetics and other products, and to cultivate agricultural crops. The facility for separating and recovering CO2 (CCU facility) has been installed at the Saga City cleaning plant and has been in operation since August 2016. Currently, around the Saga City cleaning plant, there is a concentration of industries using waste biomass-derived CO2 as a resource that will contribute to the formation of a decarbonised society and the creation of employment. Saga City, which was certified as a biomass industrial city in 2016, aims to become “a city where what used to be waste is recycled while creating value as energy and resources”, and the CCU project is one of the projects to realise this future vision.
Public intervention and supporting policies
Policy instruments, such as public procurement and subsidies, can be used to encourage the creation of markets for products using waste biomass-derived CO2 and the introduction of equipment to capture the CO2 used in their production. The involvement of the public (local government) is essential for the CCU project at the waste treatment plant because it uses local environmental infrastructure, and that environmental infrastructure carries a negative image. In addition, public involvement is also required for the handling of the separated CO2 (storage or utilisation). In particular, in the case of CO2 utilisation, the involvement of government is necessary because there may be restrictions under existing laws depending on the business development. In addition, direct support, such as subsidies, is effective in developing CCU technology in Japan. Saga City’s CCU project has received support (subsidy) from the Ministry of the Environment for the construction of the facilities, and the relevant ministries have cooperated in clearing the legal hurdles for the industrial use of CO2.
Korea: The transition towards a carbon-neutral economy: Sustainable chemicals and fuels production using nanotechnology in Korea
Summary
This project is focussed on technologies to produce sustainable chemicals and fuels from captured CO2 emitted by the oil refinery process. A large proportion of heavy industries such as steel, petrochemicals, oil refining, and cement emit large amounts of GHGs in Korea. The industry sector was estimated to be responsible for 37% of Korea’s total GHG emissions in 2017.
The CCU Customised for Oil Refinery project aims for development and demonstration of an on-site CCU process with a scale of 1-10 tonnes CO2 per day (TRL 6-7), including CO2 capture technology for CO2 emission sources in an oil refinery complex (e.g., fluid catalytic cracking unit), catalytic and electrocatalytic CO2 conversion technologies to produce syngas and olefins, and fuels (gasoline, diesel, jet, etc.) production technology from syngas. The key technology of the project is nanotechnology for the development of high-performance CO2 conversion catalysts to produce sustainable chemicals and fuels.
Public intervention and supporting policies
This case study provides an example of CCU innovation R&D project in Korea, a ‘CCU Customised for Oil Refinery (2022-2025)’ with a budget of USD 25 million supported by the Ministry of Trade, Industry and Energy (MOTIE).
In Korea, CCUS technologies were developed by Korea CCS 2020 Project with a budget of USD 150 million supported by the Ministry of Science and ICT for years 2011- 2020. The CCS technology developed in this project has been applied to a multi-ministerial joint project (USD 50 million, 2021-2023), and a large-scale integrated CCS demonstration project is currently underway.
Norway 1: Production of fish feed by gas fermentation
Summary
In this case study the enabling technology is bacterial fermentation using CO2 as carbon source and energy from green hydrogen. The bacterium used is a naturally occurring strain of Cupriavidus necator, which has been extensively studied and is approved for food and feed applications.
The main sustainability objective is to replace soy as a protein ingredient in feed for aquaculture of salmon. Farmed salmon is the second largest export industry in Norway with an annual production of 1.35 million tonnes, requiring more than 400 000 tonnes of concentrated protein in the feed. The import of soy is the main source of CO2 emissions within the salmon industry, both directly from soy cultivation, processing, and transport and indirectly from land-use change. Beside its negative climate effects, soy cultivation is seen as a major cause of deforestation and biodiversity loss.
For Norway, sustainably produced feed ingredients are key, not only to continue salmon farming at the current level, but for further expansion. So far, public measures to stimulate this industry have focussed on technology and innovation. This case study illustrates the interplay of several technologies creating a unique industrial ecosystem, comprising gas fermentation, advanced aquaculture, novel CO2 capturing systems, and improved water electrolysis for hydrogen production.
Public intervention and supporting policies
In Norway, three main public facilitator organisations are cooperating to establish a pilot facility for gas fermentation. In 2016, Innovation Norway, the main government agency for industry development, took an initiative to map and describe opportunities in this field. Subsequently, SIVA, a funding body for research infrastructure, acquired an existing facility suitable for gas work, and the Norwegian Research Council provided a major grant to fund a first set of equipment. This open-access facility is now being administrated by NORCE with G2F as its first customer.
Norway offers several national R&D funding programmes driving the green transition, and several companies involved have received public support for their respective projects. This case study illustrates the importance of grant mechanisms simulating complete value chain and cross-sectorial cooperation. In 2021 the Norwegian government announced the ambitious goal that all feed should come from sustainable sources by 2030. Recently, sustainable feed has been defined as a national mission and development of a set of grant programmes and policies are in progress.
Norway 2: Decarbonisation strategies of a ferrosilicon plant
Summary
Finnfjord is a ferrosilicon producer located in northern Norway. While virtually all their electrical power consumption comes from hydropower, they are still dependent on fossil carbon as a reducing agent in their metallurgical process, leading to an annual emission of 300 000 tonnes of CO2.
This case study is about Finnfjord’s multiple strategies to become carbon neutral. In summary they have four options, and a particularly interesting aspect of this case study is the fact that all four strategies are actively pursued. A first option is to introduce bio-based carbon in their core process. Alternatively, the emitted CO2 could be captured for permanent storage (CCS). This case study, however, is focussing on their two alternatives for CCU, which include one biotechnology project (cultivation of microalgae) and one chemical project (production of e-methanol).
Public intervention and supporting policies
The relative attractiveness of each alternative will depend on future market regulations and policy framework. The microalgae project depends on an early-stage technology; hence technology risk is the main concern and public R&D grants are important. Based on the potential value for the Norwegian aquaculture industry, Finnfjord has recently been offered a national grant of NOK 53 million or USD 5 million to further develop and test their microalgae process at full industrial scale. It is also expected that this funding will finance a detailed sustainability assessment.
The e-methanol project in contrast, is about deploying an already proven technology. While there is still technology risk in the actual process integration, for instance in energy optimisation, the dominating risks are regulatory, e.g., related to future CO2 emissions tax and potential market incentives for green methanol. Given the inherent risk in first-of-kind projects, the Finnfjord/CRI/Statkraft consortium is applying for a flagship grant from the EU Innovation Fund.
The case study illustrates how public policies can influence the attractiveness of different industry options, thus steering the sustainability consequences and trade-offs. If the carbon tax is too high, CCS would probably be preferred. This would benefit climate, but not biodiversity. If, on the other hand, market incentives are strong, carbon recycling (CCU) would be more attractive, potentially reducing the pressure on bioresources. However, assuming a combination of high carbon tax and strong market stimulation, DAC may become a preferred option for future microalgae or e-methanol production, both from a commercial and a sustainability perspective.
United Kingdom: CCUS for Zero Carbon Fertilizer production
Summary
The study is of a commercialised technology for the production of net-zero carbon fertilizers to allow a wide range of businesses to generate commercial value from captured carbon and other agricultural and industrial waste streams while also delivering improved sustainability. It is now commercially viable without any government subsidies. It uses captured CO2 from industrial power generation to stabilise a wide variety of materials (such as ammonia and phosphates) from agricultural and industrial waste streams and uses these to create new fertilizer products with significantly lower than usual carbon and resource footprints.
Public intervention and supporting policies
This technology is important in realising climate policy goals as it commercialises and incentivises capturing and converting waste, and it targets one of the most difficult decarbonisation sectors - agriculture, which currently contributes around 10% of UK greenhouse gases. The technology is fully commercialised and therefore relies on no UK government subsidies.
United States 1: Hybrid technologies for recycling waste carbon using gas fermentation and upgrading
Summary
The case study focusses on a public-private partnership (US Department of Energy and LanzaTech) for fermentation of waste gas to produce sustainable aviation fuel (SAF) while illustrating other opportunities for carbon recycling. Technologies for waste gas recycling include gas fermentation, which produces ethanol and other chemicals directly, and chemical catalysis. SAF is made by combining gas fermentation (biotechnology) with chemical catalysis in a specific hybrid process called Alcohol-to-Jet (ATJ). Such hybrid processes offer pathways to a broad range of carbon-based products from wastes.
In the first commercial plants to demonstrate this technology, LanzaTech used bacteria to produce ethanol from CO-rich steel mill or ferroalloy waste gases. That ethanol can then be converted to SAF using the ATJ process, which has been demonstrated on two commercial flights. Moving forward, a 10 million gallon per year ATJ production facility is under construction at LanzaJet Freedom Pines Fuels, LLC in Soperton, Georgia, USA, with support from the US Department of Energy.
Public intervention and supporting policies
On 9 September 2021 the US government announced new steps to coordinate innovation across the federal government, aircraft manufacturers, airlines, fuel producers, airports, and non-governmental organisations to advance the use of cleaner and more sustainable fuels in American aviation12. As a part of this effort, a new Sustainable Aviation Fuel Grand Challenge was announced to produce at least 3 billion gallons of SAF per year by 2030 and sufficient SAF to meet 100% of aviation demand by 205013. Together, these announcements send a strong signal that decarbonisation of the aviation sector is a key piece of the broader US decarbonisation strategy.
An important driver for SAF is the International Civil Aviation Organization’s (ICAO) Carbon Offsetting and Reduction Scheme for International Aviation (CORSIA)14 agreement that aims to lower CO2 emissions on international flights to reduce contributions of the aviation industry to CO2 levels. Adopted in 2016 with available purchases of carbon credits to offset emissions, CORSIA aims for carbon-neutral growth from 2020. Because another implementation option is a procurement pledge by airlines for increasing volumes of SAF over time, the private sector is working to develop processes to deliver SAF to meet an EPA finalised new rule to set carbon emissions standards for airplanes manufactured in the United States15.
The US Congress is considering supportive policies including grant programmes for the US Department of Transportation, as well as GHG performance-based tax credits16. Other potentially impactful policies include the US Renewable Fuel Standard (RFS)17 which mandates alternative fuel production through use of RINs (renewable identification numbers). To the extent that fuel pathways also sequester carbon through CCUS, they may also be eligible for tax credits under section 45Q of the US tax code. Sub-national policies such as California’s Low Carbon Fuel Standard (LCFS)18 are also important drivers for action as evidenced by the fact that nearly all SAF used in the US today is sold in the State of California.
In applied research and development, industrial partners cost-share the government contribution at 20% (research) to 50% (development). In addition to research, the US government helps mitigate risk for first-of-a-kind, clean energy projects through loan guarantees. For example, the US Department of Energy supported the SAF development in this case study and supported the second-generation fermentation reactor that is currently at a pilot stage.
The US government also provides instruments that allow their national laboratories to work with industry including Cooperative Research and Development Agreements (CRADAs) and transferring technology from laboratories to industry. Establishing technology-neutral policies is critical and the policy must support the end goal. Finally, it remains important that policy instruments support and allow broad licensing of the technology in the United States and abroad.
United States 2: Digital agriculture: Soil organic carbon networked measurements technologies
Summary
Realising the potential for carbon-negative agriculture offers a significant step towards meeting US climate targets, including administration goals of a net-zero economy by 2050. Equally significant, however, is the ability to accurately measure this sector’s contribution. Today’s emissions monitoring, reporting, and verification (MRV) tools for agricultural carbon intensity (i.e., g CO2-eq. per acre) comprise complex instrumentation, laborious sampling and analysis, and operational challenges (e.g., weather interference), which have restricted the resolution and fidelity of MRV outputs. If the broader bioeconomy is to deliver fully on its potential, there is an urgent need to better quantify the emissions footprint of natural systems so that it can accurately account for its economic and environmental impacts.
This case study is focussed on the US Department of Energy’s SMARTFARM programme19, a new experimental two-phase effort underway to advance agricultural measurement technologies and practices for carbon management. Focusing on biofuel feedstock crops, the programme is structured in two phases, with the first phase focussed on gathering high-resolution carbon intensity data using state-of-the-art tools and technologies (e.g., acre/sub-acre sampling, eddy covariance towers, soil chambers), and the second phase focussed on developing new metrology technologies capable of low-cost, low-uncertainty MRV of carbon intensity.
Due to the critical nature of agricultural outputs such as food, fibre, and fuel, the emissions associated with the sector have traditionally been accepted as the cost of doing business. The global carbon budget has now reached a point at which no sector can be left out of the drawdown equation. As the global economy shifts its ambitions from incremental carbon reduction to dramatic carbon removal, the agricultural sector has a unique role to play by offering relatively large-scale, low-cost, and, importantly, near-term sequestration; however, the sector cannot serve as a net sink without adequate incentives, and incentives are difficult to distribute without justification.
Public intervention and supporting policies
While technology solutions are needed to bridge the data gap in agricultural supply chains, there are several challenges that could prevent the distribution of much-needed financial incentives for agricultural carbon drawdown, including:
Protocols are varied in terms of contractual and MRV obligations, and available protocols lack rigor in terms of MRV, which introduces uncertainty about the net carbon impact of enrolled farms. Though technology limitations place a major economic constraint on MRV options for these protocols - a constraint that the SMARTFARM programme seeks to eliminate - there is a broader question of how farmers are expected to access, compare, and securely enter agreements with carbon market developers.
Credit quality is varied in terms of uncertainty, additionality, and residence time. This variation can make it difficult for credit buyers to know what they are paying for. While variation is likely to persist as carbon markets mature, clarity in what the crediting requirements are (i.e., degree of certainty, additionality, residence time) and how they are verified can help buyers make decisions and improve estimates of the sector’s overall carbon impact.
Carbon drawdown requires new management tools and techniques, which need to be proven in multiple cropping environments to evaluate efficacy and gain farmer acceptance. Farmer education about how and where to implement these practices can help to mitigate concerns and optimise implementation.
Public intervention through programmes such as SMARTFARM can bring transparency, accessibility, and credibility to the process of reducing agricultural emissions and increasing soil carbon uptake. These efforts, when combined with the resources of the private sector can provide a significant pathway to farmer acceptance and adoption of practices that optimise for yield and carbon intensity, paving the way for a reimagined agriculture sector that sequesters more carbon than it emits.
Notes
← 1. https://cfs.nrcan.gc.ca/publications?id=40337 Last accessed 1 March 2023.
← 2. www.xprize.org/prizes/carbon/teams/carbon_upcycling_nlt Last accessed 1 March 2023.
← 3. www.eralberta.ca/grand-challenge-innovative-carbon-uses/ Last accessed 1 March 2023.
← 4. https://impact.canada.ca/en/challenges/green-aviation Last accessed 1 March 2023.
← 5. https://op.europa.eu/en/publication-detail/-/publication/edace3e3-e189-11e8-b690-01aa75ed71a1/language-en/format-PDF/source-149755478 Last accessed 1 March 2023.
← 6. Direttiva (UE) 2018/851, che modifica la direttiva 2008/98/CE relativa ai rifiuti, e direttiva (UE) 2018/852, che modifica la direttiva 1994/62/CE sugli imballaggi e i rifiuti di imballaggio.
← 7. https://ec.europa.eu/info/strategy/priorities-2019-2024/european-green-deal_it Last accessed 1 March 2023.
← 8. https://ec.europa.eu/info/strategy/priorities-2019-2024/european-green-deal/actions-being-taken-eu/farm-fork_it Last accessed 1 March 2023.
← 9. https://dicam.unibo.it/en/research/research-projects/conversion-of-off-shore-platforms-for-multiple-eco-sustainable-uses-place Last accessed 1 March 2023.
← 10. https://ponricerca.gov.it/ Last accessed 1 March 2023.
← 11. http://bluegrowth-place.eu/ Last accessed 1 March 2023.
← 12. www.whitehouse.gov/briefing-room/statements-releases/2021/09/09/fact-sheet-biden-administration-advances-the-future-of-sustainable-fuels-in-american-aviation/.
← 14. www.icao.int/environmental-protection/CORSIA/Pages/default.aspx Last accessed 1 March 2023.
← 15. www.epa.gov/regulations-emissions-vehicles-and-engines/control-air-pollution-airplanes-and-airplane-engines-ghg Last accessed 1 March 2023.
← 16. www.natlawreview.com/article/implementation-recent-amendments-to-45q-carbon-sequestration-tax-credit Last accessed 1 March 2023.
← 17. www.epa.gov/renewable-fuel-standard-program Last accessed 1 March 2023.
← 18. www.transportpolicy.net/standard/california-fuels-low-carbon-fuel-standard/ Last accessed 1 March 2023.