This chapter lays out the main messages and major policy findings derived from an evidence base which consists of: sixteen national case studies from nine OECD member states; four international workshops during the research phase of the project; one workshop post-research; contributions to several IEA/Biofuture Platform workshops leading up to the Clean Energy Ministerial1; desk research and detailed inputs from the steering group for this work. As a further aid to policy analysis, the IEA maintains a database of CCUS policies2.
Carbon Management: Bioeconomy and Beyond
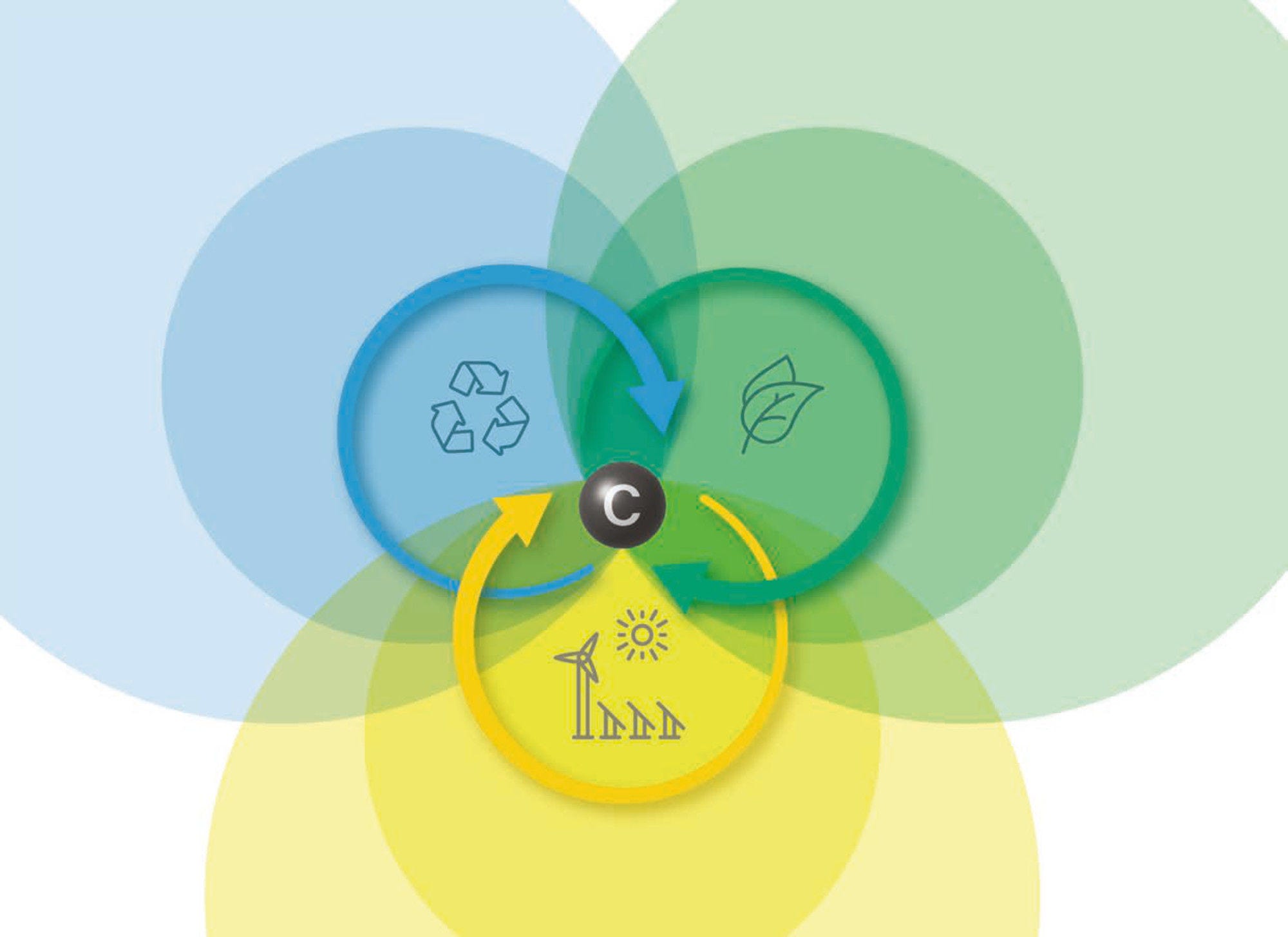
2. Main policy implications and recommendations
Abstract
Carbon management – balancing policy trade-offs and dilemmas
Sustainability tunnel vision
With the recent and significant attention given to emission reduction to mitigate climate change, other aspects of sustainability have sometimes been crowded out of policy conversations, creating a potential for unintended consequences that later may need to be reversed (if possible).
What may be called ‘sustainability tunnel vision’ is to be avoided by policy makers as it may provide a tendency to approve technologies and actions that reduce emissions while simultaneously compromising other sustainability goals, such as biodiversity, water quality, food security (Figure 2.1) For example, evolution takes millions of years to create the current genetic diversity in nature, thus loss in biodiversity is just as irreversible as climate change.
Figure 2.1. Sustainability tunnel vision

Source: Cognizant Research. Graphic by Jan Koniezko , https://digitally.cognizant.com/moving-beyond-carbon-tunnel-vision-with-a-sustainability-data-strategy-codex7121.
National studies increasingly find that the availability of biomass for net zero in industry could be a bottleneck (Fuss, 2021). Many OECD member states are biomass importers. In Norway the import of large volumes of soy protein is essential for its fish farming industry (Norwegian case 1). On the other hand, the United States has done extensive work over a number of years to show the feasibility of being able to resource at least a billion tons of biomass per year sustainably (USDOE, 2017, 2016, 2011, 2005).
Creating a high demand for biomass from countries rich in biomass carries the risk of initiating unsustainable harvesting practices to meet export demand. For example, there are many negative economic, environmental and social consequences of illegal logging (Reboredo, 2013). It already costs nations tens of billions of dollars annually (Lynch et al., 2013), and deforestation and forest degradation account for close to 20% of global emissions3. It readily descends into violent crime, warlordism, even assassinations (Nuwer, 2016; Scheidel et al., 2020). Between 15 and 30% of wood traded globally is obtained illegally, rising to 50-90% in key tropical countries (Nellemann, 2012), making deforestation very difficult to control, and creating more than one unintended consequence that would need to be corrected.
The challenge of value-based policies
Carbon footprint, life cycle analysis (LCA), and other sustainability assessment mechanisms represent technical exercises, while balancing different sustainability criteria is an ethical or value-based political process. Hence, the implementation of global policies faces the challenge of obtaining agreement across geographical, economical, ethnic and religious groups, which cannot be solved by rational arguments alone.
In a green transition, values and therefore values-based policies will be given higher priority as there are important issues such as land rights, workers’ rights, indigenous people’s knowledge and their protection from biopiracy, child labour, forced labour. This is enshrined in the principles of ‘just transitions’4. With forestry and agriculture and their residues as increasingly exploited feedstocks, policy need to include forest owners, landowners and farms owners. In the European Union alone, there are 16 million private forest landowners, most of them owning small forests (Hetemäki et al., 2017).
Social impact assessment methods still lack a global consensus (Bouillass et al., 2021). Social LCA (S-LCA), while relatively new, is continuously evolving (Marting Vidaurre et al., 2020). S-LCA integrates traditional life cycle assessment but with the focus on social aspects. Sala et al. (2015) presented methodologies and indicators for S-LCA for supporting product policies in order that social and economic benefits can be improved while reducing environmental impacts.
The need to prioritise land use and bioresources
Land use and land use change is a major, if not the major, source of sustainability trade-offs. The concept of indirect land use change (ILUC) (Searchinger et al., 2008) refers to land use change occurring elsewhere when crops used for biofuels and bioproducts displace the production of food or feed. Hence, even when confined to certified feedstock, a general increased in demand may still trigger ILUC. It has proven both controversial and influential, as demonstrated by legislation in countries with low-carbon fuel policies such as Canada, the United States and the European Union to require inclusion of sustainability criteria for biomass feedstocks used in low-carbon fuel production (Khanna et al., 2017). Its measurement has, however, proven inconsistent, reducing confidence in its use.
As sustainable biomass is a limited feedstock, it would be optimal to use the biological complexity and unique properties of biological molecules where it is most valuable and efficient, e.g., food, fibre and bioactives. In other words, optimise for the carbon value instead of the energy value of biomass, an approach broadly consistent with new approaches in the US DOE. It further suggests that it would be a waste to use it simply for energy production or in many cases to decompose it to simple chemical building blocks for the chemical industry. Here, recycling or CCU-derived hydrocarbons would be a better alternative.
This is reflected in Figure 2.2, showing that while biomass will be an important starting point for green chemicals, CO2 will take over as the predominant feedstock for the German chemical industry from 2040 onwards. A similar prediction is found for sustainable aviation fuel (SAF) in the IEA Net Zero by 2050 scenario.
Figure 2.2. A scenario for future feedstocks of the German chemical industry

Source: Kircher (2020)
A specific initiative within the German plan for the protection of climate, Klimaschutzplan-2050, illustrates policies to stimulate the use of CO2 as a raw material5. The Federal Ministry for Education and Research (BMBF) is contributing to the Klimaschutzplan-2050 under the national framework programme on ‘Research for Sustainability – Fona’6. Under Fona, a first funding measure on using CO2 as raw material was published in 2018: ‘CO2Plus – material use of CO2 to broaden the basis of raw material’7.
Further policy aspects of carbon recycling
Conversion of traditional waste streams is seen as the basis for a circular economy and cascading value chains (Klitkou et al., 2019). However, it is important to avoid implementing policies for local recycling of carbon that do not make sense from an overall energy perspective (Hernandez and Cullen, 2019). Indeed, such policies may in fact sub-optimise energy consumption within a larger system. Most industry processes are validated by calculating the mass and energy balances of the process (Larsson, 1992) and carbon conversion technologies need to be subjected to the same scrutiny.
Even if CCU is motivated primarily by a reduced need for bioproduction and stress on land resources, rather than emission reduction per se, energy considerations require CCU technologies to be chosen with care. With the right application it may also provide opportunities for mid- to long-term carbon sequestration (Peplow, 2022). While a liquid fuel may return the carbon to the atmosphere very quickly, carbon incorporated in a construction material may have a duration of decades or longer. These aspects of carbon recycling technologies should be preferably assessed in their early developmental trajectories.
Local and international access to key resources
Regional aspects of feedstocks and energy
Biomass and the other renewable carbon feedstocks such as waste recycling, will differ in availability in different regions. Regions and countries need to be aligned in goals even if their strategies differ. Hence, a mostly rural region may have a different strategy from a highly industrialised one. National policy makers will need to be comfortable with different strategies that aim for the same end goal.
Still, regions may find themselves in competition over feedstocks and/or energy carriers. Renewables will presumably alter how regions and countries interact, thereby impacting trade and patterns of cooperation (Scholten et al., 2020) and nations will need to align their foreign and trade policy appropriately.
Government programmes are promoting research and development across supply and value chains, but the markets for supply of material feedstocks and energy receive comparatively less attention (Knight et al., 2015). Uncertainty with respect to key resources is a main factor that deters investors. This lack of attention to supply markets possibly reflects reluctance by governments to be seen to be intervening in markets and potentially contravening anti-competitive practices (Institute of Risk Management and Competition and Markets Authority, 2014).
Analysis points to the potential importance of buyer cooperatives and other forms of supply market intermediaries to facilitate access to bioresources (Knight et al., 2015). This is consistent with the activities of publicly funded regional clusters. Regional clusters are well positioned to evaluate local options, to build capacity in the regions, and then to look beyond the regions (Philp and Winickoff, 2017). Building capacity at the local level depends on business quality and business relationships of trust. Building beyond the clusters can exploit the expertise gained at regional level to expand and join up with other regions. Such clusters can be creating “significant local added value, local jobs and reducing climate emissions” (Refsgaard et al., 2021).
It is important that regions can demonstrate their strengths and weaknesses to national governments in the competition for resources and investment. In the context of smart specialisation8, France mapped all its regions in detail (Box 2.1). The analysis resulted in a definition of the smart specialisation areas (SSAs) for each region. This policy approach has several advantages and is a model that could be adapted specifically for innovation towards net-zero carbon.
Box 2.1. Defining a smart specialisation strategy for research and innovation in the French regions
“In response to the European Commission's ambitions, the French regions have committed to mobilising nearly 20% of the ERDF [European Regional Development Fund] total amount for the first thematic objective concerning research, transfer development and innovation, because innovation and territories are closely linked. While innovation is rooted in the wealth and diversity of territories and their residents, the ability to innovate is a major issue for each territory and a key development and job creation factor”.
Marie-Caroline Bonnet-Galzy, Commissioner General for Territorial Equality, in: General Commission for Territorial Equality (2015)
A policy document was prepared as a tool for innovative ecosystem stakeholders and public decision makers to facilitate comparison and cooperation between French regions, including overseas territories. It also helps the regions of other countries to identify the French regions’ strengths and envisage collaboration. The tool analysed each region with respect to strengths and weaknesses and mapped key figures like gross domestic (GERD) and business enterprise expenditure (GBERD), as well as numbers of researchers, patents and business creation.
The United States Billion Ton reports may give leads on how to harmonise the approaches to biomass sustainability, which vary in underlying methodologies, assumptions and analyses. It is important to effectively estimate the sustainable capacities for biomass production for both domestic use and international biomass trade.
Connecting national to regional approaches was an important element of the Japanese Biomass Towns concept that came out of the 2002 Biomass Nippon Strategy. The strategy sets three types of goals - technical, regional and national, with specific action plans for production, collection and transportation, conversion technologies and for energy or material use (Kuzuhara, 2005). With the encouragement of central government, a total of 318 municipalities participated in making Biomass Town plans (Box 2.2).
While the local dimension of bioresources from agriculture or forestry is obvious, access to other feedstocks like industry carbon gases from cement and steel plants (CCU) or solid waste recycling could also have a local dimension. A typical consideration for a CO2 emission point is the choice between CCU and CCS. As previously discussed, utilising CO2 will depend on the local availability of energy, while CCS would typically need either to have road or rail transport of the gases or a dedicated pipeline, to the point of long-term geological storage.
Box 2.2. The Biomass Town Plan policy, Japan
The Biomass Town is an area where a comprehensive biomass utilisation system is established and operated. Each step from biomass generation, conversion, distribution and use is coordinated through the various stakeholders, and, importantly, the activities are appropriate to the local community.
A Biomass Town Plan is a planning document that describes the target area characteristics, implementing bodies, goals and effects, the procedure for developing the plan, biomass potential, and biomass utilisation, all of which eventually contribute to building consensus among various stakeholders to formulate the Biomass Town. Local governments led the development and implementation of the plans to realise Biomass Towns. As such the procedure bears similarities to Smart Specialisation, with a sharp focus on biomass.
Biomass Industrial City
Subsequently, the Biomass Commercialization Strategy was formulated by seven ministries in 2012 and the Biomass Industrial City/Region Scheme, which concentrates on economic sustainability, started in 2013. So far, more than 70 municipalities are recognised as Biomass Industrial Cities/Regions.
Note: See the case study on Saga City Japan (Japan 2: Efforts of CCU by the Saga City cleaning plant). Saga City was certified as a Biomass Industrial City in 2016, with the aim to become a city that recycles waste while creating value as energy and resources.
International feedstock trading
Large quantities of biomass are being shipped around the globe, with most of it destined for OECD countries. However, trading of biomass is controversial, due to many dilemmas. It is reasonable to assume that access to biomass will become a primary strategic asset. As with petroleum, biomass is a critical resource which is not equally distributed, and biomass is thus likely be a cause of international rivalry and disputes (Marvik and Philp, 2020). Today, the strategic importance of arable land is typically related to food security, but access to biomass will also be essential for energy and other sectors, like chemicals and materials.
The energy required to transport large volumes of biomass argues in favour of local utilisation, but in the absence of sufficient quantities of sustainable biomass, import from abroad may be essential. The European Union for example, imports 16% of its biomass needs (Lühmann, 2021). While demand is expected to increase in the efforts to defossilise industry, there is little potential for expanding local production, implying that import is likely to increase. In the UK, the Drax powerplant is an illustrative case. In their efforts to move away from coal, they currently import 8 million tonnes of wood pellets, primarily from North America9.
Geopolitical shifts may occur, increasing the pressure to harmonise transnational biomass policies. International biomass disputes have already started and there is a need to control this through policy to ensure smooth future trade. An extreme example is the blockage of wheat ready for export from Ukraine during the summer of 2022. Bosch et al. (2015) highlighted biomass disputes that can range from trivial to very serious. For example, the increase in palm oil production in Indonesia from 2006-2010 was accompanied by a range of economic, environmental and social negative consequences (Obidzinski et al., 2012).
In 2015, four of Asia’s largest companies were excluded from the Norwegian sovereign wealth fund, the largest in the world, due to concerns over severe environmental damage caused by land clearing at Indonesian palm oil plantations10. This was the first time that Norway’s central bank made the final decision rather than the Ministry of Finance in an effort to depoliticise the fund. In all four cases, Norges Bank’s executive board decided there were no other options but divestment.
Accepting that international biomass disputes are inevitable, there may be a need for the establishment of an international biomass dispute settlement facility (Taanman and Enthoven, 2012) (Box 2.3). In 2013, the Roundtable on Sustainable Palm Oil established a dispute settlement facility11 (DSF) with these exact matters in mind.
Box 2.3. An international biomass dispute settlement facility (BDSF) as a governance tool
Biomass may be the most controversial of the renewable feedstocks due to its connection to land and land use. A strong ethical component of governance is necessary due to the plethora of environmental and social consequences of non-sustainable overuse of biomass: soil erosion, loss of carbon sink function, biodiversity loss, water pollution, and more difficult to quantify, social issues such as indigenous people’s rights, workers’ rights, child labour, fraud, illegal logging.
The idea for a BDSF was explored by Taanman and Enthoven in 2012 for the Netherlands Department of Economic Affairs, Agriculture and Innovation and the City of The Hague. Thirty-five stakeholder interviews were conducted and unanimously all interviewees expected that the number of biomass conflicts will increase in future as pressure on available fertile land grows.
Joint fact finding, negotiation, mediation, arbitration and judicial settlement are the main mechanisms for dispute settlement. International organisations prioritise mediation and arbitration over judicial settlement (Allee and Elsig, 2015).
Accounting for carbon in imports and exports
Disputes and carbon management intersect when carbon-containing goods and biomass are exported around the world. The import of carbon-intensive goods from a country with less stringent climate action controls is open to unintentional or deliberate abuse by the importer and the exporter. Exporting countries can shift production to countries with lower controls. Importing countries can have products replaced by more carbon-intensive imports.
The EU Carbon Border Adjustment Mechanism (CBAM)12 is considered a “landmark tool to put a fair price on the carbon emitted during the production of carbon intensive goods that are entering the EU, and to encourage cleaner industrial production in non-EU countries”. The CBAM is meant to ensure the carbon price of imports is equivalent to the carbon price of domestic production, and that the EU's climate objectives are not undermined. Moreover, the CBAM is designed to be compatible with WTO-rules.
Training the workforce of the future
The need for a specially trained workforce will exist throughout the different technologies of the green transition. While competence in chemistry or engineering will remain key in green manufacturing, some emerging technologies would be crucially dependent on unique skills.
In the burgeoning synthetic biology industry for instance, the skills and education of the workforce is considered by many to be a key pinch point for the industrial development. However, it is argued that at least 30 PhDs in biology and biochemistry are available for every technical engineer (Kitney et al., 2021), thus what could be missing is the training of engineers, mathematicians and computer scientists with a basic understanding of biology.
While much attention is directed towards training of PhDs, a workforce development programme needs to be much broader, for instance by including apprenticeships and day-release education. Higher education is rising to the challenges with a diverse range of solutions from technician training, massive open online courses (MOOCs) and business management courses (Philp, 2022).
Interdisciplinarity implies a problem-solving function not necessarily implicit to multidisciplinarity. A useful distinction was made by Boix Mansilla et al. (2000) who framed interdisciplinarity as the capacity to integrate knowledge and modes of thinking in two or more disciplines.
This, in fact, challenges the teachers as much as the students: MacLeod (2018) stated that “traditional scientific education and training has remained divided by disciplines such as microbiology, chemistry and computing. The challenges to higher education remain on many levels”. Multi- and interdisciplinary education would break this silo situation to create graduates more adept at problem solving.
Public R&D support for carbon management technologies
Selection criteria for innovation support programmes
In business literature, innovations have been considered the most critical driver of economic growth (e.g., Shakina and Barajas, 2020). In principle, public subsidies should support innovation programmes with the largest value for society (Nicolaides, 2013), but R&D alone is not always the most efficient tool to reach climate targets, which may require more specific selection criteria and coupling to market stimulation (Fischer and Newell, 2007).
Innovation policies range from framework conditions, generic measures to support the business population to more targeted direct (grant or loan funding) and indirect (e.g., R&D tax credits) measures for SMEs and entrepreneurs. As such, often a wide number of ministries and agencies across government at both central and subnational level are involved. This makes for a complex domain to deliver effective, efficient and coherent policies. Figure 2.3 is an adaptation of a diagramme from the Milken Institute (2013) showing the major steps along the way.
Figure 2.3. Funding renewable carbon technology development

Source: Adapted from Milken Institute (2013)
The vast array of technical challenges in carbon management indicates the need for hybrid technologies if any one technology does not meet all requirements of economic and environmental benefits. For example, a biotechnology process used to generate small-molecule intermediates followed by chemo-catalytic conversion to larger fuel molecules is likely to reach commercialisation faster than biotechnology alone (Lynd et al., 2022). This may create difficulties for government agencies responsible for grants and investments in these technologies due to the multi-disciplinarity inherent in building hybrid processes.
For innovation in carbon management, it is especially important to combine both techno-economic and environmental assessment that can reveal the impact of policy incentives (Scown et al., 2021). No environmental impact model exists that accommodates all aspects of circularity (Das et al., 2022). When recycled and renewable materials are involved, mass balance analysis is a chain-of-custody approach to account for materials entering and leaving a system.
The mass balance approach is typically used in the chemical industry, where the volume of renewables is allocated to take account of all yields and losses13. Mass balance of materials may be obvious, but just as important is energy consumption. Funding a project that makes sense in terms of materials, but does not make sense from an energy perspective, may result in public money being wasted.
Development of carbon management technologies may be particularly difficult for startups and SMEs, as the timeline and cost of realising a new technology or process can be prohibitive. Moreover, these emerging processes will typically support a public good, rather than a well-defined consumer need, thus public funding bodies should be prepared to accept immature markets and higher market risk than has been the norm.
In order to increase the chances of success, R&D programmes are often reinforced by auxiliary programmes. SynbiCITE14 is the industrial translation engine for seven key UK synthetic biology research hubs. SynbiCITE supports the growth of companies (start-ups and SMEs) in synthetic biology by providing scientific and technical support through its staff scientists working in the context of an advanced biofoundry. In addition, SynbiCITE provides business support and business courses (Basic Business and Lean Launchpad). In 2022 a new investment company was created (SynBioVen) with an investment of GBP 20 million to partner with SynbiCITE.
Greater efficiency of public investments might be obtained through the public finance of production facilities at pilot and/or demonstrator scale, although the benefit needs to be proven. Models already exist e.g., the GBP 24 million Centre for Process Innovation (CPI)15 in the UK. CPI helps SMEs understand the commercial feasibility of products or processes in a way that reduces risk to the companies and their investors. Such facilities could maximise their benefits by offering a range of ancillary business services, such as training, quality management and certification (Schieb and Philp, 2014).
Support for an emerging technology seen as a public good justifies a strong focus on targeted instruments for R&D, but also realising that broadly based R&D programmes complement targeted instruments as they can discover new applications from more blue-sky research and answer fundamental research questions of low value to the private sector. In other words, science and science funding should adapt to combine curiosity driven science with science more relevant to society’s needs (Rodrigues, 2021).
A relevant example is the open access NFFA consortium for nanotechnologies16. NFFA is a pan-European consortium of 22 international partners with a core of 13 co-located foundries and large-scale facilities (Figure 2.4). It allows researchers to face complex nanoscience challenges that cannot be provided by any single research infrastructure alone. Supported by the Horizon 2020 Pilot project, it offers free of charge access for academia and industry.
Figure 2.4. The NFFA consortium
Prize competitions as an innovation driver
Fixed contracts, grants and prizes all have roles in the innovation process, and prizes have been hailed for some advantages. One purported advantage is that they can build a larger community of practice compared to, say, grants, as inducement prize competitions tend to be open to an audience wider than technical experts. Experience at NASA suggests that prizes work best when the government can articulate a specific problem requiring solutions which can leverage private sector market demand (National Academies of Science, Engineering and Medicine, 2020).
The XPRIZE for Carbon Removal17 is the largest inducement prize in history at USD 100 million. It is a four-year competition open to teams from anywhere on the planet, with the objective of specifically pulling CO2 from the oceans or atmosphere and to sequester it sustainably. To win the grand prize, teams must demonstrate a potential for scaling:
Demonstrate a working solution to remove at least 1 000 tonnes per year.
Model their costs at a scale of 1 million tonnes per year.
Show a pathway to achieving a scale of gigatonnes per year in future.
Industry clusters set to enhance innovation
The main rationale for public policies to promote technology clusters through infrastructure and knowledge-based investments, networking activities and training is an increase in knowledge spillovers among actors in clusters (Box 2.4). This is purported to generate a collective pool of knowledge that results in higher productivity, more innovation and an increase in the competitiveness of firms.
Technology and regional clusters are a leading support mechanism for SMEs (Wilde and Hermans, 2021), providing a range of services, such as: access to venture capital and other finance routes; business advice on the strategic use of standards, labels, certificates, assistance with specific LCA and sustainability tools, access to demonstration and testing facilities. National government programmes can provide a wide range of support mechanisms for clusters, especially exemptions from tax and national insurance payments.
Box 2.4. Cleantech Cluster, Lithuania
There are many relevant clusters to choose from in Europe as the publicly funded cluster model has proven very popular. The motivations and competencies of Cleantech Cluster Lithuania illustrate the typical capabilities of clusters. Cleantech Cluster Lithuania connects clean technology companies, science and research institutions and other entities that contribute with their professional knowledge, skills, business activities, reputation and experience.
Collaboration: Members collaborate in developing new solutions, products and services, participate in public tenders and international funding programmes.
Corporate image: Membership in the cluster creates added value for the company offering features of responsibility and sustainability.
Networking: Cluster membership allows access to specialised information and contacts with decision makers. The cluster coordinator engages in lobbying and networking.
Innovation and finance: The cluster promotes innovation, creates a better environment for R&D, reinforces the value chain of the clean technology sector and facilitates access to investment.
Other financing instruments for green projects
As shown in Figure 2.3, several funding mechanisms and financial instruments may be relevant as projects progresses. Green Bonds is a further option to enable capital raising for new and existing projects with environmental benefits. The Green Bond Principles18 instrument is a mechanism to mobilise large capital sums, with the financing and management of project risks undertaken by the project sponsors, not the investors that might or might not have the capacity to manage said risks.
Green Banking can serve climate goals by financing climate change mitigation technologies hand-in-hand with the private sector. A government-backed green investment bank differs from a typical fund in that it should not just disburse government money, but as a bank it should be able to raise its own finance and fill a gap in the market for government-backed bonds, bring in banking expertise and offer a range of commercially driven interventions - loans, equity and risk-reduction finance. As a publicly capitalised entity, a green investment bank may facilitate private investment into domestic low-carbon and climate-resilient infrastructure and other green sectors such as water and waste management. These dedicated green investment entities have been established at national level and at state and even city level (OECD, 2016). Akomea-Frimpong et al. (2021) reviewed various instruments for green banking.
The UK government has created an Infrastructure Bank19, capitalised with GBP 22 billion to fund low-carbon investment and rapidly scale up operations. It is meant to be a key instrument for the United Kingdom to meet its net-zero emissions target by 2050. This is partly a response to the UK Energy Security Bill outlined in April 2022. It also coincides with appointing Ofgem20 as the new energy regulator to ensure consumers get heating at a fair price.
The root problem: Sustainable alternatives are often less competitive
Public market stimulation is necessary
This green transition to net-zero carbon by 2050 is fundamentally different from the major transitions since the first industrial revolution. The transitions from wood to coal and coal to oil were transitions to more efficient feedstocks and energy carriers, but with the key component of pollution as an unpriced market externality. As a result, the market was dominant in their uptake, never having to bear the economic cost of pollution.
In this green transition, however, the opposite pertains, and in the absence of public policy this makes the transition unattractive to the market. Thus, in the green transition many markets based on renewable carbon will need to be driven by policy. New carbon supply chains depend on novel technologies; hence implementation of carbon management is strongly connected to innovation policies.
Table 2.1 shows a simple example of how carbon tax could influence the relative attractiveness of CCS, CCU and DAC. If the carbon tax is too high, CCS would probably be preferred. This would benefit climate, but not biodiversity. If, on the other hand, market incentives are strong, carbon recycling (CCU) would be more attractive, potentially reducing the pressure on bioresources. While DAC technologies are at an early stage of development, DAC may eventually become a significant alternative to biomass (Fuss, 2021). Policies will determine the relative attractiveness of CCS, CCU and DAC, as illustrated in Table 2.1. Thus, assuming a combination of high carbon tax and strong market stimulation, DAC may become a preferred option, both from a commercial and a sustainability perspective.
Table 2.1. Policies will determine the attractiveness of CCU
Policies for: |
Supply side |
Demand side |
Sustainability benefits |
---|---|---|---|
Continued emissions |
Low |
Low |
Business as usual |
CCS |
High |
Low |
Stimulating decarbonisation but no benefit for land use and biodiversity |
CCU |
High |
High |
Reducing pressure on bioresources but limited climate mitigation effects |
DAC |
Extra high |
Extra high |
DAC becomes competitive to CCU: Benefits for climate as well as bioproduction and biodiversity |
Source: OECD (2022)
Public policies will have to make choices
As many markets in the green transition depend on regulations, it is unavoidable that public policies take a role in selecting the trajectories towards a more sustainable society. It further implies that public policies will be involved in prioritising technologies and technical pathways in the new green supply chains. This report aims to guide policy makers in their implicit choices of technology platforms and associated feedstocks as they negotiate the uncharted waters of innovation and potential unintended consequences.
Urgency creates a dilemma for governments. From upstream research through downstream R&D&I to demonstration/flagship projects, urgency dictates that failures have to be minimised due to the proximity of 2050. This calls for careful selection of projects for public funding and intensive scrutiny of value chain and public-private partnership stakeholders. Selection criteria have to go beyond good science and engineering to include techno-economic assessments, resource availability, social impact assessment, and environmental credentials (not necessarily just emissions reduction).
The term ‘picking winners’ first arose in the context of technology foresight in 1983, the message being that this is exactly what government should do i.e., invest in emerging technologies that could enable economic development (Irvine and Martin, 1984). This was in direct conflict with the ideology that the market should do the picking, not governments, with the argument that governments are ill-equipped to do so. The one-off logic of picking winners has been largely rejected.
On the other hand, it can be argued that a core function of government is to supply public goods or benefits (e.g., climate change mitigation) that markets either fail to provide or cannot provide efficiently (Anomaly, 2013). Given the short-term run up to 2050, it is critical that the optimal outcome technology paths are chosen. Hence, the ‘picking’ should be based on societal benefit criteria and assessment procedures involving both public and industry considerations. In conjunction with industry, philosophy could change to “creating winners” (White and Wilkinson, 2017) and retaining them (Autio and Rannikko, 2016) rather than picking them. For retention, Autio and Ranniko argue for policy initiatives that:
1. are highly selective, e.g., requesting evidence for emission reductions
2. emphasise strong growth motivation as a key selection criterion
3. control milestone achievements and condition support on achievement
4. promote the exchange of insights on how to effect rapid organisational growth
5. rely on public–private partnerships for hands-on, capacity-boosting support.
Note that none of these recommendations question the technology-neutrality principle. Actual emissions reduction technology and trajectory selection should be technology-neutral, which has long been a favoured principle in governments for the design of policy in areas characterised by rapid technological change (Aisbett et al., 2021), and is especially relevant to hybrid technologies (see Policies to stimulate industrial symbiosis).
Project assessment framework to guide public investment
A diversity of sustainability criteria and certification schemes
There are many proposed indicators of sustainability21, far too many for it to be immediately apparent which can be used to measure sustainability. In addition, there is no collectively agreed-upon definition, making measuring sustainability difficult (El-Chichakli et al., 2016). Moreover, as discussed in The challenge of value-based policies, the challenge is exacerbated by the difficulty of value-based comparison of different sustainability objectives.
Today, theories of sustainability rest on three pillars: economic, environmental, and social – some remain more difficult than other to measure. While there are standardised methodologies for measuring economic and environmental aspects, social aspects are much more difficult to quantify (van Dam and Junginger, 2011). Furthermore, sustainability frameworks that focus on social implications also may bear a more holistic meaning of sustainability by including the health and well-being of people and societies (Shawki, 2016).
Tools used to measure environmental impacts include Input/Output Analysis, Life Cycle Assessment (LCA), Material Flow Analysis, Recycling Efficiency Rate, Global Reporting Initiative (GRI), and GHG Indicators. LCA is the most commonly used approach, and its ability to quantify means that it can be used to compare environmental impacts of different processes and manufacturing systems. Social LCA, as discussed, focuses on social impacts of the product and hence can address organisational aspects of the value chain. Life cycle costing (LCC) assesses all the costs of a product during its entire life cycle.
While all of these tools have their pros and cons, there is no one single consolidated tool to date. In fact, there is no internationally agreed framework for measuring biomass sustainability, no agreed criteria and no agreed tools for measurement (Bracco et al., 2018) and none of the tools available is robust enough to cover sustainability in all three pillars (Iacovidou et al., 2017). There is at least one international journal, Environmental and Sustainability Indicators22, dedicated to the subject.
Sustainability indicators should be easy to use and certification systems inexpensive to incentivise uptake by SMEs as they comprise the bulk of private companies. Without commonly agreed upon and standardised tools and frameworks, the unintended consequence could result in de facto standardisation of competing sustainability indicators and frameworks, leaving private companies at odds with government climate goals (de Freitas Netto et al., 2020). There is a surprising lack of empirical studies on how companies are measuring the environmental impact of their business in practice (Das et al., 2022), which reinforces the need for policy intervention.
Currently, the UN Food and Agriculture Organization (FAO) is developing a methodology for assessing sustainability of bio-based products. It recommends a step-by-step approach to sustainability assessment that considers all three pillars, and also differentiates between territorial and product assessments. As part of this approach, countries or producers and manufacturers are provided with a long list of scientifically robust indicators, from which to choose a limited set of core indicators that suits their needs and circumstances (FAO, 2019).
There are various private sector suppliers of sustainability certification schemes (SCS), notably the Roundtable on Sustainable Biomaterials (RSB) and International Sustainability and Carbon Certification system (ISCC). The ISCC system23 covers all sustainable feedstocks, including agricultural and forestry biomass, waste and residues, non-biorenewables, recycled carbon materials and the respective supply chains. It is also notable in its coverage of sectors; it is already used globally for the chemical industry, packaging, industrial applications as well as in the food, feed and bioenergy markets (Schmitz, 2020).
The importance of standards and certification in policy
Stringent standards and certification give confidence to consumers and industry as they provide credibility to claims of performance and sustainability (Dammer and Carus, 2015), such as ‘bio-based’, ‘renewable raw material’, ‘biodegradable’, ‘recyclable’, or ‘reduced greenhouse gas impact’. They help verify claims such as biodegradability and bio-based content that will promote market uptake (OECD, 2011). Third party verification is a means to prevent unwarranted claims and greenwashing.
According to the British Standards Institution (BSI), a surprisingly large proportion of annual productivity growth can be attributed to standards (BSI, 2015). Companies can use matching or beating a standard as an R&D and marketing tool, which then spurs competitors to innovate further, driving technical advances and delivering efficiencies. Standards are typically developed in close cooperation between industry, research and policy makers, which is essential to create the right environment for new products and technologies to grow to full-scale deployment.
Standards and certification schemes are also joining-up measures between policy frameworks and practical implementation. Standards provide the necessary scientific basis for implementing legislation by demonstrating compliance with legal requirements. Similarly, they are essential for all public market intervention, and they are important to verify that policy goals and targets are being met.
The inadequacy of industrial classification codes
National industrial classification codes have multiple important functions. One of these is to enable market and economic analysis in specific sectors and sub-sectors. Unfortunately, their usefulness to measure the progress of the green transition is often limited.
By way of example, Carlson (2016) found that in the United States it was impossible to (economically) distinguish a bio-based chemical and the identical chemical made from fossil resources. This is because there is no North American Industrial Classification System (NAICS) code (Executive Office of the President of the United States, 2017) for these products. The only relevant code is for a subset of pharmaceutical production. However, for the 2022 revision, the US Office of Management and Budget (OMB) has accepted recommendations with respect to bio-based products manufacturing and renewable chemicals manufacturing, including the decision to “continue research and outreach in this important emerging area” (Federal Register, 2021). A team is in the process of making recommendations in this space and is currently drafting a Request for Information.
Similarly, Ronzon et al. (2020) described the challenges in estimating ‘bio-based shares’ for sectors which only partially belong to the bioeconomy, as reported in the European NACE (Nomenclature Statistique des Activités Économiques dans la Communauté Européenne) classification24. NACE codes refer to “fermentation of sugarcane, corn or similar to produce alcohol and esters” (European Commission, 2008), but there is no general classification system for chemicals or materials based on biomass or other renewable carbon sources.
Market intervention to encourage private investments in renewable carbon
The most pressing need for the net-zero carbon transition is investment, both private and public. As demonstrated, the green transition is an overhaul of global economics and environmental commitments, with far-reaching social ramifications. The financial commitments are significant, being measured in the USD trillions by 2050. Initially this focus was on energy and transportation transformation, however it has become clear that it must involve all sectors and all countries.
While only mainstream private finance can match the scale of climate action needed for the net-zero transition, it is unlikely that the ambition will be realised without large concomitant public funding to de-risk private investments. Unfortunately, there is little room for manoeuvre as time is of the essence, and public investments have to be efficient, maximising climate effect while minimising failures. Therefore, not only do public investments need to protect private investment through de-risking, but the public investments also need protection.
Hence, the overarching challenge for government policies in general is to encourage investment in renewable carbon technologies while discouraging further investment in fossil. On the supply side a major push is required to de-risk private investments in testbeds, pilot and demonstration facilities. Large public investments are needed to get emerging technologies to an advanced stage. Equally urgent are policy measures to develop the demand-side (market) to make private investments flow. Market interventions must be consistent over time, however, as market uncertainty is lethal to private finance.
Tax incentives have become a favoured policy tool that governments use to encourage companies to invest in R&D (OECD, 2020b). The sums required for technology deployment are likely to be much higher than in R&D, especially in capital projects and those requiring bespoke or highly specialised large-scale equipment. In the United States, federal production and investment tax credits have helped promote the development and deployment of wind and solar energy technology and more recently CCUS technologies. Similarly, Canada (Box 2.5) has introduced tax incentives from the CCUS sectors, while Denmark has chosen a strategy based on contracts for difference25.
Box 2.5. Canadian investment tax credit for CCUS
The 2022 Canadian Federal Budget of April 7 establishes a refundable investment tax credit for Carbon Capture, Utilisation and Storage (CCUS) beginning in 2022. The CCUS Tax Credit follows the announcement of its 2030 Emission Reduction Plan for achieving a net-zero economy by 2050.
The tax credit seeks to incentivise CCUS technologies that capture CO2 emissions (from industrial processes, fuel combustion, or directly from air) for storage or utilisation in industrial processes. It will offset the costs of purchase and installation for eligible equipment.
The CCUS Tax Credit is offered on a sliding scale on the cost of purchasing or installing eligible equipment incurred in a taxation year, provided that the eligible equipment is used in a CCUS project for an eligible use. For the years 2022-30 the CCUS Tax Credit is:
60% for eligible capture equipment used in a DAC project
50% for other eligible capture equipment
37.5% for eligible transportation, storage and use equipment.
Source: Johnson et al. (2022).
‘Stick policies’ – lower dependence on continued use of fossil carbon
The advantages that fossil holds at present are so overwhelming that governments need to create greater balance. In fuels and energy, where the policy is most mature, the gulf to be crossed is still stark (Figure 2.5). The lack of a level playing field is greatly exacerbated by large-scale fossil fuel subsidies, many of which are at odds with an industry over 100 years old. Combining fossil fuel subsidy reform with a realistic carbon price and carbon tax are the two most obvious ways to encourage investment in renewable carbon use and necessary for meeting the United Nations sustainable development goals (SDGs) (El-Chichakli et al., 2016).
Figure 2.5. Global fuels investments demonstrate the reality of the competition between fossil and renewable energy

Source: IEA (2023), World Energy Investment 2023, IEA, Paris www.iea.org/reports/world-energy-investment-2023, License: CC BY 4.0
An IEA analysis has suggested that no further new investments in fossil fuels should be made if 2050 climate goals are to be reached. However, planned investments within the oil majors, both private and state-owned, show that the industry is preparing for massive investments26. In response to that article, Fatih Birol of the IEA “warned against investing in large new oil and gas developments, which would have little impact on the current energy crisis and soaring fuel prices but spell devastation to the planet”27.
Discontinue fossil fuel subsidies
Monitoring fossil fuel subsidies, the IEA has routinely returned annual subsidy figures in the region of USD 0.5 trillion. The International Renewable Energy Agency (IRENA) tracked USD 634 billion in total energy-sector subsidies in 2020 and found that while around 70% went to fossil fuels, 6% went to biofuels and just over 3% to nuclear (IRENA, 2020). OECD calculations showed a significant drop in subsidies during COVID-19, with a predicted surge in 202128 as economies reopened (Figure 2.6).
Figure 2.6. Fossil fuel subsidies fail to reach those in society that would most benefit

Note: Data for 2021 are on a preliminary basis.
Source: IEA/OECD press release (2022) “Support for fossil fuels almost doubled in 2021, slowing progress toward international climate goals, according to new analysis from OECD and IEA www.oecd.org/environment/support-for-fossil-fuels-almost-doubled-in-2021-slowing-progress-toward-international-climate-goals-according-to-new-analysis-from-oecd-and-iea.htm (29 August 2022)
Fossil fuel subsidies challenge governments’ net-zero pledges while swamping investment in sustainable energy infrastructure. Governments could use the money saved by scrapping these subsidies to fund decarbonisation projects and technologies. The International Institute for Sustainable Development (IISD) has estimated that removing fossil fuel consumption subsidies in 32 countries would cut their GHG emissions by an average of 6% by 2025 (IISD, 2021).
While most of these fossil subsidies are inefficient and wasteful, their removal has proven difficult (OECD, 2020a) and is exacerbated by international crises. For any country attempting reform, removing such subsidies all at once could cause serious economic reverberations, with the possibility of social unrest, as was seen in Ecuador in 2019 when a fuel tax hike was introduced (Timperley, 2021). Kazakhstan, in the top 25 countries for fossil fuel subsidies29, erupted in violence in 2022, sparked by a rise in the price of liquefied petroleum gas (LPG)30.
What has been working, however, is a self-peer review process. Since 2013, G20 countries have developed and implemented a methodology for voluntary, country-led peer reviews of fossil fuel support as a “valuable means of enhanced transparency and accountability” (OECD/IEA, 2021). While some countries have made progress in reducing or phasing out these subsidies, others increased subsidies as part of rescue and recovery from the pandemic-induced crisis (OECD/IEA, 2021).
Fossil carbon emission taxes
Carbon taxes and emission trading systems (ETS) are the most cost-effective means of reducing CO2 emissions (OECD, 2013). In addition, they also encourage investment in climate change mitigation technologies (Probst et al., 2021) and clearer consumption choices for all public and private spending, which can also future-proof investments (OECD, 2021).
Explicit carbon prices can either be set through a carbon tax, expressed as a fixed price per tonne of emissions, or through cap-and-trade systems, where an emissions reduction target is set through the issuance of a fixed number of permits, and the price is set in the market through supply and demand. In an emissions trading scheme or system, firms must remit allowances to cover their emissions. In essence, a government fixes the supply of allowances, and allowance trading establishes the emissions price.
In 2021 China opened the world’s largest emissions trading market31, covering 40% of its emissions, and 12% of global emissions, as part of drive to net zero by 2060. China will also use a declining cap to ensure emissions fall every year. At the time of writing there are 65 carbon prices schemes implemented in 45 national jurisdictions, representing some 21.5% of global GHG emissions32. However, the level is set nowhere near high enough: the average price of emissions worldwide is only around USD 2 per tonne, when a realistic level would be much higher. In the OECD framework for decarbonisation of the economy (OECD, 2022), it is evident that most countries under-price their carbon emissions.
Recently, vom Berg et al. (2021) have argued that a fossil carbon tax, i.e., a raw material tax, would be more efficient and simpler to implement than an end-of-pipe CO2 emissions tax. In other words, taxing fossil fuels directly at extraction from the well may be more efficient.
The world’s operating or developing carbon market schemes had a combined value of USD 272 billion at the end of 202133, including Europe, Canada, China, Japan, New Zealand, South Korea, Switzerland and the United States. There are essentially two methods for using revenues from these taxes to help grow the net-zero carbon economy. In the first, revenues are added to the general budget of a government, and that government can choose to use these revenues for climate-friendly purposes. Alternatively, the revenues can be legally earmarked or hypothecated for specific projects or purposes, rather than being added to the general budget. Both approaches have advantages: adding to the general budget minimises the cost for new administration, while earmarking is more direct, transparent, and perhaps easier to gain public acceptance (Marvik and Philp, 2020).
Tax revenue could be a cost-effective way to support long-term, higher risk research and more targeted short- to mid-term R&D, pre-competitive or near-market. There is also a case for the return of tax revenues to consumers in a ‘citizen dividend’. Budolfson et al. (2021) found that revenues from carbon tax will be large enough to fund policies that can promote equality and relieve poverty. The choice is political, but judicious spending across a range of purposes is indicated.
Carbon tax is not a panacea
Carbon taxes target CO2 emissions from fossil fuels. They do not directly target other carbon compounds like methane, which has a larger warming potential. Carbon emissions from many sources, such as agriculture, deforestation, waste management, and poor land use, are hard to assess and monitor and these are generally not taxed directly (World Bank, 2022). While most US policy schemes implicitly price such emissions in terms of CO2 equivalents as part of full LCA assessment, non-tax-based policies would alternatively be needed to discourage emissions from sources like waste and farm management and land use practices.
A disadvantage of carbon taxes is that they may affect poorer people comparatively more than the more affluent. If the tax is accompanied by price rises, standard of living may drop, triggering unrest. Governments will need to consider if a phased increase in carbon tax would be favourable to mitigate unintended consequences. Coupling to less extreme but also less efficient measures, such as feebates and regulations, might improve public acceptability further due to their smaller or less direct impact on energy prices (IMF/OECD, 2021).
Strong, transparent and inviolable rules and regulations would be needed to make a carbon market work. Otherwise, emissions might even increase, and countries might ‘double-count’ their carbon saving efforts or take credit for low-carbon projects which would have happened anyway. Few carbon offsetting schemes are properly regulated, making the assessment of emissions reduction very difficult to measure and confirm. The burgeoning carbon offset industry has already been beset with doubts about the operational efficiency. Indeed, carbon offset schemes have been termed a “bookkeeping trick”34, to obscure real emissions. West et al. (2023) examined the effects of 26 carbon offset project sites to reduce deforestation in six countries on three continents and found that most projects had have insignificant effects on reducing deforestation. For projects that did have effects, the reductions were substantially lower than claimed. This could lead to the conclusion that, in fact, offsetting to contain deforestation is failing (Jones and Lewis, 2023).
ETS versus Effective Carbon Rate
The ‘effective carbon rate’ sums carbon price, ETS and fuel excise. For different sectors the composition of effective rates is useful for a government to see how the system is working and where adjustments might be warranted. Figure 2.7 shows the effective carbon rates across the G20 countries in 2021.
Figure 2.7. The composition of effective carbon rates by sector, G20 economies, 2021

Note: G20 includes all the OECD countries and non-OECD Inclusive Framework jurisdictions that are members of the G20, except Saudi Arabia. Taxes are those applicable on 1 April 2021. The ETS price is the average ETS auction price for the first semester of 2021, except for China and the United Kingdom where it is based on information for the period in which they were operational (China: 16/07/2021, United Kingdom: 19/05/2021-30/06/2021) and the U.S. RGGI and Massachusetts and Tokyo subnational systems where, due to data limitations, the 2020 average was used. ETS coverage estimates are based on the OECD’s (2021), Effective Carbon Rates 2021, with ad hoc adjustments to account for recent coverage changes. Emissions refer to energy-related CO2 only and are calculated based on energy use data for 2018 from IEA (2020), World Energy Statistics and Balances. The figure includes CO2 emissions from the combustion of biomass and other biofuels. Carbon prices are averaged across all energy-related emissions, including those that are not covered by any carbon pricing instrument.
Source: OECD (2022), Taxing Energy Use 2022
In the electricity and industry sectors, emissions pricing mostly takes the form of emissions trading systems. In all other sectors, fuel excise taxes continue to dominate compared to explicit carbon prices. In buildings, there is a roughly even split between emissions trading systems and carbon taxes (OECD, 2021). The most relevant sector for this report is industry, and this could signal to governments that higher revenues might be more easily generated by improving the effective carbon rate through the carbon tax.
‘Carrot policies’ – building markets for renewable carbon
A level playing field is needed to include all economic sectors in all countries if net-zero carbon is to be achieved. However, this century has seen large and global policy action in energy and transportation, most prominent among the instruments being quotas and mandates for liquid biofuels.
It may not be surprising that the vast majority of public policy has been directed towards energy. Nevertheless, the mineral, chemical and material sectors account for the largest industrial sources of emissions, and it is now time for governments to focus here as decarbonisation of the energy and transport sectors is beginning to happen.
Production targets and mandates for renewable carbon fuels
Targets and mandates exemplify the different approaches to the introduction of biofuels in Europe and the United States. Incorporation targets (i.e., targets of percentages of biofuels blended into gasoline and diesel) have been approved voluntarily by several EU member states as national initiatives. The US biofuels policy has specified absolute production quantities through a mandate rather than a less-binding incorporation target (Ziolkowska et al., 2010). Mandates and targets for biofuels production have become standard for introduction of biofuels, and what follows may be partially or fully applicable to other low-carbon fuels such as CO2 fuels.
Volume-based biofuels mandates provide certainty of demand within defined timescales, which gives the policy certainty that investors seek. They focus government support on certain technologies. Mandates are not without controversies and their application needs to be carefully thought through before deployment. A lesson from biofuels mandates is the need for countries to properly assess the technology pros and cons prior to unrolling market incentives such as mandates and to match them with focused technology-push policies. This way a country can match the technologies to its longer-term goals (Biofuture Platform, 2018). Conversely, if poorly chosen the selected mandated technologies may not provide the most cost-effective alternative to mitigate GHG emissions of the mandated sector(s) (Biofuture Platform, 2018).
In the European Union, ambitious blending mandates for sustainable aviation fuel (SAF) were recently agreed35 as part of ReFuelEU Aviation (Box 2.6). Consistent with the IEA Net Zero 2050 scenario, the proposed mandates include not only bio-based fuels, but a progressing sub-mandate for synthetic fuels, i.e., fuels based on recycled carbon. This market stimulation scheme for SAF illustrate the importance of well-defined criteria for qualifying production pathways.
Box 2.6. European Union sustainable aviation fuel blending mandates
As part of the EU’s “Fit for 55” package, the ReFuelEU Aviation blending mandates were agreed between the European Parliament and the European Council in April 2023, representing all 27 member states. This SAF policy, which is set to be implemented from 2025, will require airlines to use a minimum level of sustainable fuels for flights departing from EU airports. As part of the deal, airports will be required to ensure that their fuelling infrastructure is “fit for SAF distribution” (see Table 2.2).
The SAF mandate will start at a minimum of 2% share of SAF from 2025, rising to 70% by 2050 (Table 2.2). Moreover, there is a further requirement that a certain fraction of SAF should be synthetic fuel, i.e., based on recycled carbon defined by a set of detailed regulations. This sub-mandate will start at 1.2% in 2030, increasing to 35% in 2050. In other words, half of the SAF blending in 2050 should be synthetic fuels. At the time of writing, the step-up plan for synthetic fuel sub-mandates in 2040 and 2045 is not clear.
These blending mandates will require well-defined production routes. In addition to comply with a 70% emission reduction compared to a standard, there are further criteria related to allowed feedstocks. For instance, fossil CO2 can only be used as feedstock for synthetic fuel (RFNBO) until 2041, after which the CO2 should come from biogenic sources or DAC. The energy used, which in practice means hydrogen, should only come from renewable sources, implying that green hydrogen is accepted, while blue hydrogen is not.
It is noteworthy that a 20% blending mandate in 2035 would translate into a market demand of about 12 million tonnes of SAF in Europe alone. The fact that the current global capacity of SAF is only in the order of 300 000 tonnes illustrates the ambition level of this policy scheme.
Table 2.2. RefuelEU: Agreed mandates (April 2023)
Year |
Overall SAF mandate |
Synthetic sub-mandate |
---|---|---|
2025 |
2% |
- |
2030 |
6% |
1.2% |
2032 |
- |
2% |
2035 |
20% |
5% |
2040 |
34% |
Unclear |
2045 |
42% |
Unclear |
2050 |
70% |
35% |
Lapan and Moschino (2012) found biofuels production mandates to be more revenue-neutral than tax and excise reductions. They derived that an ethanol volume mandate is equivalent to a combination of an ethanol production subsidy and a fossil fuel (petrol) tax that is revenue neutral. They conclude that the (optimal) ethanol mandate yields higher welfare than the (optimal) ethanol subsidy.
An alternative to production mandates is based on carbon intensity, as used in the California Low Carbon Fuel Standard (LCFS), which sets annual carbon intensity (CI) standards. This approach is technology-agnostic, and the standard, or benchmark, decreases annually, with the intent of reducing the GHG emissions in transport by 20% in 2030. The policy effect in California is worth noting. About 60% of the market value of cellulosic ethanol is proving to be policy driven. This makes emerging biofuels with low carbon intensities cost-competitive with fossil fuels in the California market (IEA-AMF, 2020).
Stimulating markets for renewable carbon in chemicals
Public policy for reducing emissions in the mineral, chemical and material industries is a very different proposition compared to the energy sector. Energy has well-defined markets and regulation, and the number of fuels is small, while chemical and material products constitute tens of thousands. Thus, in these sectors obeying rules on carbon accounting, measurement and reporting is a much harder task than in energy.
A carbon intensity scheme would of course not work for carbon-based chemicals. Moreover, with tens of thousands of products, it is difficult to see how mandates or other support mechanisms used in the energy sector could be applied to renewable chemicals (Philp, 2015). Many aspects would be more complicated to assess, e.g., data gaps on real emissions savings, highly variable production pathways with different feedstock and energy inputs. Most of all, the bureaucracy behind mandating production of so many products would be most unattractive to the producers. However, a great many of the fossil products are manufactured via naphtha or a small number of platform chemicals such as olefins, hence a full LCA for the more limited number of key base chemicals may work in this sector.
Carus et al. (2014) described an innovative approach to the final problem above. As the biofuels market has now determined levels of GHG emissions savings for different categorisations, then it might be possible to describe an “ethanol equivalent”, from which other chemicals could be compared.
Green public procurement
Public procurement affects a substantial share of world trade flows. It accounts for 13% of GDP on average in OECD member countries (OECD, 2012). It is also being increasingly used to drive innovation and economic growth (OECD, 2019). Public procurement is a highly fragmented landscape: in the EU there are over 250 000 public authorities, and public procurement accounts for 14% of EU GDP (Núñez Ferrer, 2020).
Public procurers naturally tend to be very price-sensitive, which is a barrier for any innovative product. A real success story is the USDA BioPreferred Program36, which specifically aims to increase the purchase and use of bio-based products. The programme has identified 139 categories of bio-based products (e.g., cleaners, carpet, lubricants, paints) and around 20 000 products for which agencies and their contractors have purchasing requirements.
There are broadly two main activities of the Biopreferred Program: mandatory federal purchasing and a voluntary labelling initiative (USDA, 2020). Providing a central product registry through an online catalogue enables purchasers to locate and compare products from all participating manufacturers, thereby encouraging them to compete to provide products with higher bio-based content.
More than 3 500 products have been approved to use the USDA Certified Biobased Product label (Figure 2.8). The programme partners with the ASTM International37 (formerly the American Society for Testing and Materials) to ensure quality control and consistent results. This offers purchasers a universal standard to assess a product’s bio-based content.
Figure 2.8. The USDA Biopreferred voluntary label

Note: The capital letters FP appear on the label if the product is qualified for Federal Procurement.
Source: Courtesy of Andrew Jermolowicz, Director, Business Development Division, Rural Business- Cooperative Service, USDA Rural Development.
Policies to stimulate industrial symbiosis
Industrial symbiosis can be defined as38 “the use by one company or sector of underutilised resources broadly defined (including waste, by-products, residues, energy, water, logistics, capacity, expertise, equipment and materials) from another, with the result of keeping resources in productive use for longer”.
Stimulating cross-sectorial cooperation can assume many functions, including networking stakeholders, building resilient value chains, reducing capital needs, and consolidating the availability of resources and skilled labour. The main benefit of industrial symbiosis, however, may be energy optimisation, reducing overall cost, and the interplay between multiple applications of the same feedstock, reducing resource as well as business risk in the whole supply chain.
The concept is by no means new: as far back as at least 1997, the industrial ecology concept had been linked to decarbonisation (Erkman, 1997), but it can attract new impetus with the present policy focus on decarbonisation and carbon management. Perhaps the most famous example is the decades-old Kalundborg symbiosis in Denmark. At Kalundborg, waste, including physical materials but also heat, from one industrial facility becomes a feed to another.
While this is entirely consistent with the theory of the circular economy, Kalundborg is an example of a self-organised industrial symbiosis. A more deliberately planned model e.g., Zero carbon Humber, UK (Box 2.7, Figure 2.9) is integrated for carbon management and net-zero carbon planning. The planned model needs a conscious effort to identify firms and make them co-locate to share resources, therefore, it frequently involves at least one governmental agency.
Box 2.7. The proposed industrial symbioses at Humber, UK
A highly relevant example still under development is the UK Humber symbiosis, relevant because it encompasses CCS, hydrogen and BECCS (Figure 2.9). Its coastal location allows for sub-sea storage of CO2 in the Southern North Sea. This is likely to be the exception rather than the rule as suitable sites for geological storage are limited. The location is described as the most carbon-intensive industrial region of the UK. For it to be viable in the future in the face of ever-tightening environmental legislation, CCS and clean hydrogen are most likely a necessity. Note that the plan accommodates “deep water facilities for international shipping of CO2, green hydrogen and ammonia”. There are 14 formal partners, with public support from over 50 other international, national and regional organisations.
Figure 2.9. A schematic of what “Zero carbon Humber” might look like
References
Aisbett, E., W. Cheng and F. Beck (2021), “Green industrial policy and technology neutrality: odd couple or unholy marriage?”, ZCEAP Working Paper 01-21, 3 March, 2021. Australian National University, Canberra.
Akomea-Frimpong, I., D. Adeabah, D. Ofosu and E.J. Tenakwah (2021), “A review of studies on green finance of banks, research gaps and future directions”, Journal of Sustainable Finance & Investment, DOI: 10.1080/20430795.2020.1870202.
Allee, T and M. Elsig (2015), “Why do some international institutions contain strong dispute settlement provisions? New evidence from preferential trade agreements”, The Review of International Organizations 11, 89-120.
Anomaly, J. (2013), “Public goods and government action”, Politics, Philosophy & Economics 14, 109-128.
Autio, E. and H. Rannikko (2016), “Retaining winners: Can policy boost high-growth entrepreneurship?”, Research Policy 45, 42-55.
Biofuture Platform (2018), “Creating the biofuture: a report on the state of the low carbon bioeconomy”, Biofuture Platform, https://c4c70c1f-2927-42e5-9e36-d0ba89574795.filesusr.com/ugd/dac106_f28f692c4e9242d9b4552da29e612a74.pdf?index=true.
Boix Mansilla, V., W.C. Miller and H. Gardner (2000), “On disciplinary lenses and interdisciplinary work”, In: S. Wineburg and P. Grossman (Eds.), Interdisciplinary curriculum: Challenges of implementation. Teachers College Press, New York.
Bosch, R., M. van de Pol and J. Philp (2015), “Define biomass sustainability”, Nature 523, 526-527.
Bouillass, G., I. Blanc and P. Perez-Lopez (2021), “Step-by-step social life cycle assessment framework: a participatory approach for the identification and prioritization of impact subcategories applied to mobility scenarios”, The International Journal of Life Cycle Assessment 26, 2408-2435.
Bracco, S., O. Calicioglu, M. Gomez San Juan and A. Flammini (2018), “Assessing the contribution of bioeconomy to the total economy: a review of national frameworks”, Sustainability 10:1698.
BSI (2015), “The economic contribution of standards to the UK economy”, British Standards Institution, London, UK.
Budolfson, M., F. Dennig, F. Errickson, S. Feindt, M. Ferranna, M. Fleurbaey, D. Klenert, U. Kornek, K. Kuruc, A. Méjean, W. Peng, N. Scovronick, D. Spears, F. Wagner and& S. Zuber (2021), “Protecting the poor with a carbon tax and equal per capita dividend”, Nature Climate Change 11, 1025-1026.
Carlson, R. (2016), “Estimating the biotech sector’s contribution to the US economy”, Nature Biotechnology 34, 247-255.
Carus, M., L. Dammer, A. Hermann and R. Essel (2014), “ Proposals for a reform of the Renewable Energy Directive to a Renewable Energy and Materials Directive (REMD). Going to the next level: Integration of bio-based chemicals and materials in the incentive scheme”, Nova paper no.4 on biobased economy 2014-05, 46 pp. Nova-Institüt, Huerth, Germany.
Dammer, L. and M. Carus (2015), “Standards, norms and labels for bio-based products”, In: Aeschelmann, F., M. Carus, W. Baltus, H. Blum, R. Busch, D.Carrez, C. Ißbrücker, H. Käb, K.-B. Lange, J. Philp, J. Ravenstijn and H. von Pogrell (2015), Bio-based Building Blocks and Polymers in the World. Capacities, production and applications: status quo and trends towards 2020. Pub. nova-Institut GmbH, Chemiepark Knapsack, Köln, Germany, report 2015-05.
Das, A., J. Konietzko and N. Bocken (2022), “How do companies measure and forecast environmental impacts when experimenting with circular business models?”, Sustainable Production and Consumption 29, 273-285.
de Freitas Netto, S.V., M.F.F. Sobral, A.R.B. Ribeiro and & G. and da Luz Soares (2020), “Concepts and forms of greenwashing: a systematic review”, Environmental Sciences Europe 32, 19, https://doi.org/10.1186/s12302-020-0300-3.
El-Chichakli, B., J. Von Braun, C. Lang, D. Barben and J. Philp (2016), “Five cornerstones of a global bioeconomy”, Nature 535, 221-223.
Erkman, S. (1997), “Industrial ecology: an historical view”, Journal of Cleaner Production 5, 1-10.
European Commission (2008), “NACE rev 2. Statistical classification of economic activities in the European Community”, Eurostat Methodologies and Working Papers KS-RA-07-015-EN-N. Office for Official Publications of the European Communities, Luxembourg.
Executive Office of the President of the United States (2017). The North American Industry Classification System. www.census.gov/eos/www/naics/2017NAICS/2017_NAICS_Manual.pdf.
FAO (2019), “Indicators to monitor and evaluate the sustainability of bioeconomy”, UN Food and Agriculture Organization, Rome.
Federal Register (2021), “Office of Management and Budget, North American Industry Classification System—Revision for 2022”, Federal Register 86, No. 242, p. 72277.
Fischer, C. and R.G. Newell (2007), “Environmental and technology policies for climate mitigation”, Resources for the Future Discussion Paper 04-05 (REV), Resources for the Future, Washington, DC, https://media.rff.org/documents/RFF-DP-04-05-REV.pdf.
Fuss, S. (2021), “The importance of CCUS for reaching 2050 goals – why and how?”, Presentation at the virtual OECD-BNCT workshop: Carbon Management and Policies towards 2050, October 07, 2021.
General Commission for Territorial Equality (2015), “Synthesis of the research & innovation strategies for smart specialisation of French regions”, www.europe-en-france.gouv.fr/sites/default/files/2016_synthese_strategies_regionales_innovation_eng.pdf.
Hernandez, A.G. and J.M. Cullen (2019), “Exergy: A universal metric for measuring resource efficiency to address industrial decarbonisation”, Sustainable Production and Consumption 20, 151-164.
Hetemäki, L., M. Hanewinkel, B. Muys, M. Ollikainen, M. Palahí and A. Trasobares (2017), “Leading the way to a European circular bioeconomy strategy”, From Science to Policy 5, European Forest Institute, https://doi.org/10.36333/fs05.
Iacovidou, E., J. Millward-Hopkins, J. Busch, P. Purnell, C.A. Velis, J.N. Hahladakis, O. Zwirner and A. Brown (2017), “A pathway to circular economy: Developing a conceptual framework for complex value assessment of resources recovered from waste”, Journal of Cleaner Production 168, 1279-1288.
IEA (2020), “World Energy Investment Update October 2020”, International Energy Agency, Paris.
IEA-AMF (2020), “The role of renewable transport fuels in decarbonizing road transport”, www.ieabioenergy.com/wp-content/uploads/2020/11/Production-Technologies-and-Costs.pdf.
IISD (2021), “Cutting emissions through fossil fuel subsidy reform and taxation”, International Institute for Sustainable Development, Winnipeg.
IMF/OECD (2021), “Tax policy and climate change: IMF/OECD report for the G20 finance ministers and central bank governors”, September 2021, Italy, www.oecd.org/tax/tax-policy/tax-policy-and-climate-change-imf-oecd-g20-report-september-2021.pdf.
Institute of Risk Management and Competition and Markets Authority (2014), “Competition law risk, a short guide”, Crown copyright, London.
IRENA (2020), “Energy subsidies: evolution in the global energy transformation to 2050”, International Renewable Energy Agency, Abu Dhabi.
Irvine, J. and B.R. Martin (1984), “Foresight in science: picking the winners”, Frances Pinter Publishers, London.
Johnson, G., McInerney, T., Gilmour, B., Sigalet, B., and Ciechanowski, P. (2022). Canadian Budget Proposes New Investment Tax Credit for Carbon Capture, Utilization and Storage. Bennett Jones. Available online at: www.bennettjones.com/Blogs-Section/Canadian-Budget-Proposes-New-Investment-Tax-Credit-For-Carbon-Capture-Utilization-and-Storage.
Jones, J.P.G. and S.L. Lewis (2023), “Forest carbon offsets are failing”, Science 381, 830-831.
Khanna, M., W. Wang, T. Hudiburg, and E.H. DeLucia (2017), “The social inefficiency of regulating indirect land use change due to biofuels”, Nature Communications 8, 15513.
Klitkou, A., A.M. Fevolden and M. Capasso (eds.) (2019), “From waste to value valorisation pathways for organic waste streams in circular bioeconomies”, Routledge, London, https://doi.org/10.4324/9780429460289.
Kircher, M. (2020), “Away from oil. Potential and limits of the bioeconomy”, Springer, Heidelberg, 978-3-662-61490-7.
Kitney, R.I., J. Bell and J. Philp (2021), “Build a sustainable vaccines industry with synthetic biology”, Trends in Biotechnology 39, 866-874.
Knight, L., A. Pfeiffer and J. Scott (2015), “Supply market uncertainty: Exploring consequences and responses within sustainability transitions”, Journal of Purchasing & Supply Management 21, 167–177.
Kuzuhara, (2005), “Biomass Nippon Strategy—Why “Biomass Nippon” now?”, Biomass and Bioenergy 29, 331-335.
Lapan, H. and G.C. Moschino (2012), “Second-best biofuel policies and the welfare effects of quantity mandates and subsidies”, Journal of Environmental Economics and Management 63, 224–241.
Larsson, J.E. (1992), “Model-based measurement validation using MFM”, IFAC Proceedings Volumes 25, 127-132.
Lühmann, M. (2021), “Sustaining the European bioeconomy: The material base and extractive relations of a bio-based EU-economy”, Chapter in: Backhouse, M., R. Lehmann, K. Lorenzen, M. Lühmann, J. Puder, F. Rodríguez and A. Tittor (eds.), Bioeconomy and Global Inequalities, pp. 287-307, Palgrave Macmillan, Cham.
Lynch, J., M. Maslin, H. Baltzer and M. Sweeting (2013), “Choose satellites to monitor deforestation”, Nature 496, 293-294.
Lynd, L.R., G.T. Beckham, A.M. Guss, L.N. Jayakody, E.M. Karp, C. Maranas, R.L. McCormick, D. Amador-Noguez, Y.J. Bomble, B.H. Davison, C. Foster, M.E. Himmel, E.K. Holwerda, M.S. Laser, C.Y. Ng, C. Yu, D.G. Olson, Y. Román-Leshkov, C.T. Trinh, G.A. Tuskan, V. Upadhayay, D.R. Vardon, L. Wang and C.E. Wyman (2022), “Toward low-cost biological and hybrid biological/catalytic conversion of cellulosic biomass to fuels”, Energy and Environmental Science 15, 938-990.
MacLeod, M. (2018), “What makes interdisciplinarity difficult? Some consequences of domain specificity in interdisciplinary practice”, Synthese 195, 697-720.
Marting Vidaurre, N.A., R. Vargas-Carpintero, M. Wagner, J. Lask, J. and I. Lewandowski (2020), “Social aspects in the assessment of biobased value chains”, Sustainability 12, 9843. https://doi.org/10.3390/su12239843.
Marvik, O.J. and J. Philp (2020), “The systemic challenge of the bioeconomy: A policy framework for transitioning towards a sustainable carbon cycle economy”, EMBO Reports 21:e51478.
Milken Institute (2013), “Unleashing the power of the bio-economy”, Milken Institute, Washington, DC.
National Academies of Sciences, Engineering, and Medicine (2020), “The role of inducement prizes: proceedings of a workshop in brief”, The National Academies Press, Washington DC.
Nellemann, C. (2012), “INTERPOL Environmental Crime Programme. Green Carbon, Black Trade: Illegal Logging, Tax Fraud and Laundering in the Worlds Tropical Forests. A Rapid Response Assessment.”, United Nations Environment Programme.
Nicolaides, P. (2013), “The economics of subsidies for R&D: the intrinsic difficulty of determining optimum subsidies and implications for reform of EU State Aid rules on R&D”, Bruges European Economic Research Papers 26, http://aei.pitt.edu/58621/1/beer15_(26).pdf. Last accessed 04 February 2022.
Núñez Ferrer, J. (2020), “The EU’s Public Procurement Framework. How is the EU’s Public Procurement Framework contributing to the achievement of the objectives of the Paris Agreement and the Circular Economy Strategy?”, Policy Department for Economic, Scientific and Quality of Life Policies. Report number PE 648.770, April 2020.
Nuwer, R. (2016), “Illegal logging has become more violent than ever”, National Geographic, February 03.
Obidzinski, K., R. Andriani, H. Komarudin and A. Andrianto (2012), “Environmental and social impacts of oil palm plantations and their implications for biofuel production in Indonesia”, Ecology and Society 17, 25, http://dx.doi.org/10.5751/ES-04775-170125.
OECD (2022), “A framework to decarbonise the economy”, OECD economic policy paper 31, OECD Publishing, Paris.
OECD (2021), “Carbon pricing in times of COVID-19. What has changed in G20 economies?”, OECD Publishing, Paris, www.oecd.org/tax/tax-policy/carbon-pricing-in-times-of-covid-19-what-has-changed-in-g20-economies.htm.
OECD (2020a), “Designing fossil fuel subsidy reforms in OECD and G20 countries: a robust sequential approach methodology. OECD Publishing, Paris.
OECD (2020b) “The effects of R&D tax incentives and their role in the innovation policy mix: Findings from the OECD microBeRD project, 2016-19”, OECD Science, Technology and Industry Policy Papers, No. 92, OECD Publishing, Paris.
OECD (2019), “Productivity in public procurement a case study of Finland: measuring the efficiency and effectiveness of public procurement”, OECD Publishing, Paris.
OECD (2016), “Green investment banks: Scaling up private investment in low-carbon, climate-resilient infrastructure, green finance and investment”, OECD Publishing, Paris. ISBN 978-92-64-24511-2.
OECD (2013), “Effective carbon prices”, OECD Publishing, Paris.
OECD (2012), “Progress made in implementing the OECD recommendation on enhancing integrity in public procurement”, OECD Publishing, Paris.
OECD (2011), “Future prospects for industrial biotechnology”, OECD Publishing, Paris.
OECD/IEA (2021), “Update on recent progress in reform of inefficient fossil-fuel subsidies that encourage wasteful consumption”, www.oecd.org/fossil-fuels/publicationsandfurtherreading/OECD-IEA-G20-Fossil-Fuel-Subsidies-Reform-Update-2021.pdf.
Okereke, C. (2007), “An exploration of motivations, drivers and barriers to carbon management: The UK FTSE 100”, European Management Journal 25, 475-486.
Peplow, M. (2022), “The race to recycle carbon dioxide”, Nature 603, 780-783.
Philp, J. (2022), “Skills and education for engineering biology”, Chapter 3 in: Importance of Microbiology Teaching and Microbial Resource Management for Sustainable Futures, pub. Elsevier, in press.
Philp, J. and D.E. Winickoff (2017), “Clusters in industrial biotechnology and bioeconomy: the roles of the public sector”, Trends in Biotechnology 35, 682-686.
Probst, B., S. Touboul, M. Glachant and A. Dechezleprêtre (2021), “Global trends in the invention and diffusion of climate change mitigation technologies”, Nature Energy 6, 1077-1086.
Reboredo, F. (2013), “Socio-economic, environmental, and governance impacts of illegal logging”, Environment Systems and Decisions 33, 295-304.
Refsgaard, K., M. Kull, E. Slätmo and M. WøienMeijer (2021), “Bioeconomy – A driver for regional development in the Nordic countries”, New Biotechnology 60, 130-137.
Rodrigues, R.R. (2021), “Downside up: Science matters equally to the Global South.”, Communications Earth & Environment 2, 100.
Ronzon, T., S. Piotrowski, S. Tamosiunas, L. Dammer, M. Carus and R. M’barek (2020), “Developments of economic growth and employment in bioeconomy sectors across the EU”, Sustainability 12:4507.
Sala, S., A. Vasta, L. Mancini, J. Dewulf and E. Rosenbaum (2015), “Social Life Cycle Assessment: State of the art and challenges for supporting product policies”, EUR 27624. Luxembourg (Luxembourg): Publications Office of the European Union.
Scheidel A., D. Del Bene, J. Liu, G. Navas, S. Mingorría, F. Demaria, S. Avila, B. Roy, I. Ertöra, L. Temper and J. Martínez-Alier (2020), “Environmental conflicts and defenders: a global overview”, Global Environmental Change 63: 102104.
Schieb, P.-A. and J.C. Philp (2014), “Biorefinery policy needs to come of age”, Trends in Biotechnology 32, 496-500.
Schmitz, N. (2020), “ISCC as a solution provider for sustainably certified biopolymers supply chains”, Journal of Advanced Chemical Engineering 10, 6.
Scholten, D., M. Bazilian, I. Overland and K. Westphal (2020), “The geopolitics of renewables: New board, new game”, Energy Policy 138:111059.
Scown, C.D., N.R. Baral, M.Yang, N. Vora and T. Huntington (2021), “Technoeconomic analysis for biofuels and bioproducts”, Current Opinion in Biotechnology 67, 58-64.
Searchinger, T., R. Heimlich, R.A. Houghton, F. Dong, A. Elobeid, J. Fabiosa, S. Tokgoz, D. Hayes, T.-H. Yu (2008), “Use of US croplands for biofuels increases greenhouse gases through emissions from land‐use change”, Science 319, 1238–1240.
Shakina, E. and A. Barajas (2020), “'Innovate or Perish?': Companies under crisis”, European Research on Management and Business Economics 26, 145-154.
Shawki, N. (2016), “Norms and normative change in world politics: An analysis of land rights and the Sustainable Development Goals”, Global Change, Peace & Security 28, 249-269.
Taanman, M. and G. Enthoven, (2012), “Exploring the opportunity for a biomass dispute settlement facility”, The Hague Institute for Global Justice.
Timperley, J. (2021), “Why fossil fuel subsidies are so hard to kill”, Nature 598, 403-405.
USDA (2020), “An economic impact analysis of the U.S. biobased products industry (2019)”, www.rd.usda.gov/sites/default/files/usda_rd_economic_impact_analysis_us_biobased_products_industry.pdf.
USDOE (2017), “2016 Billion-Ton Report, Volume 2: Environmental Sustainability Effects of Select Scenarios from Volume 1, Efroymson, R.M. et al. (eds.), ORNL/TM-2016/727. Oak Ridge National Laboratory, Oak Ridge, US.
USDOE (2016), 2016 Billion-ton report: Advancing Domestic Resources for a Thriving Bioeconomy, Volume 1: Economic Availability of Feedstocks, Langholtz, M. et al. (eds.), ORNL/TM-2016/160, Oak Ridge National Laboratory, United States Department of Energy, Oak Ridge, US.
USDOE (2011), “U.S. billion-ton update: Biomass supply for a bioenergy and bioproducts industry”, Perlack, R.D. and B.J. Stokes (eds.), ORNL/TM-2011/224, Oak Ridge National Laboratory, United States Department of Energy, Oak Ridge, US.
USDOE (2005), “Biomass as feedstock for a bioenergy and bioproducts industry: The technical feasibility of a billion-ton annual supply”, ORNL/TM- 2005/66, Oak Ridge National Laboratory, United States Department of Energy, Oak Ridge, US.
van Dam, J. and M. Junginger (2011), “Striving to further harmonization of sustainability criteria for bioenergy in Europe: Recommendations from a stakeholder questionnaire”, Energy Policy 39, 4051-4066.
vom Berg, C., M. Carus, L. Dammer, T. Babayan and O. Porc (2021), “A tax on fossil carbon is more effective than a tax on CO2 emissions. A tool for elegantly pricing the true cause of global warming”, Nova Institute Report 21-05-25.
West, T.A.P., S. Wunder, E.O. Sills, J. Börner, S.W. Rifai, A.N. Neidermeier, G.P. Frey and A. Kontoleon (2023), “Action needed to make carbon offsets from forest conservation work for climate change mitigation”, Science 381,873-877.
Wilde, K. and F. Hermans (2021), “Deconstructing the attractiveness of biocluster imaginaries”, Journal of Environmental Policy & Planning 23, 227-242.
World Bank (2022), “What a carbon tax can do and why it cannot do it all”, January 19, 2022, World Bank Group, Washington DC, https://blogs.worldbank.org/energy/what-carbon-tax-can-do-and-why-it-cannot-do-it-all.
Ziolkowska, J., W.H. Meyers, S. Meyer, and J. Binfield (2010), “Targets and mandates: lessons learned from EU and US biofuels policy mechanisms”, AgBioForum 13, 398-412.
Notes
← 1. www.cleanenergyministerial.org/applications-now-open-for-cem13-mi7-side-events/#:~:text=The%2013th%20Clean%20Energy%20Ministerial,2022%20in%20Pittsburgh%2C%20United%20States Last accessed 1 March 2023.
← 2. www.iea.org/policies?topic=Carbon%20Capture%20Utilisation%20and%20Storage Last accessed 1 March 2023.
← 3. https://ec.europa.eu/commission/presscorner/detail/de/MEMO_10_511 Last accessed 1 March 2023.
← 4. "Just transitions" focus on the transition out of high-carbon activities and into the green economy, seeking to ensure harm to workers, communities, countries, and regions is avoided while maximising the benefits of climate action, www.ihrb.org/explainers/what-is-just-transition Last accessed 1 March 2023.
← 5. www.bmwk.de/Redaktion/DE/Artikel/Industrie/klimaschutz-klimaschutzplan-2050.html Last accessed 1 March 2023.
← 6. www.bmbf.de/SharedDocs/Publikationen/de/bmbf/FS/31648_Forschung_fuer_Nachhaltigkeit_en.pdf Last accessed 1 March 2023.
← 7. www.bmbf.de/foerderungen/bekanntmachung-1055.html#:~:text=Bekanntmachung%2025.06.2015%20%2D%2009.10.2015&text=Die%20F%C3%B6rderrichtlinie%20%E2%80%9ECO2Plus%20%E2%80%93%20Stoffliche%20Nutzung,%E2%80%9ENachhaltiges%20Wirtschaften%20und%20Energie%E2%80%9C.) Last accessed 1 March 2023.
← 8. https://s3platform.jrc.ec.europa.eu/what-we-do Last accessed 1 March 2023.
← 11. https://rspo.org/dispute-settlement-facility Last accessed 1 March 2023.
← 12. https://taxation-customs.ec.europa.eu/green-taxation-0/carbon-border-adjustment-mechanism_en#:~:text=The%20EU's%20Carbon%20Border%20Adjustment,production%20in%20non%2DEU%20countries.
← 13. www.vci.de/vci-online/themen/rohstoffe/use-of-renewable-raw-materials-in-the-chemical-industry-applying-mass-balance-approaches-integration-in-existing-production-and-supply-chains.jsp Last accessed 1 March 2023.
← 15. www.uk-cpi.com/work-with-us/smes/technology-focus Last accessed 1 March 2023.
← 17. www.xprize.org/prizes/elonmusk Last accessed 1 March 2023.
← 18. www.icmagroup.org/Regulatory-Policy-and-Market-Practice/green-bonds/green-bond-principles/ Last accessed 1 March 2023.
← 19. www.ukib.org.uk/ Last accessed 1 March 2023.
← 20. www.ofgem.gov.uk/ Last accessed 1 March 2023.
← 21. A definition of sustainability is given by Springer: “Sustainability indicators are quantitative and/or qualitative measures that aim to interrelate and assess different areas of social, environmental, economic, institutional, and territorial development. In general, they intend to achieve one or more of the following: evaluate sustainable development conditions and trends across time and space, monitor progress toward goals and targets, inform planning and decision making, compare different places and situations, raise awareness and encourage political and behaviour change, promote public participation, and improve communication on sustainability debates”. From the weblink: https://link.springer.com/referenceworkentry/10.1007/978-94-007-0753-5_3380. Last accessed 1 March 2023.
← 22. www.journals.elsevier.com/environmental-and-sustainability-indicators Last accessed 1 March 2023.
← 23. www.iscc-system.org/process/overview/ Last accessed 1 March 2023.
← 24. https://ec.europa.eu/eurostat/statistics-explained/index.php/Glossary:Statistical_classification_of_economic_activities_in_the_European_Community_(NACE) Last accessed 1 March 2023.
← 26. www.theguardian.com/environment/ng-interactive/2022/may/11/fossil-fuel-carbon-bombs-climate-breakdown-oil-gas Last accessed 1 March 2023.
← 27. www.theguardian.com/environment/2022/may/12/oil-gas-mega-projects-climate-iea-fatih-birol-carbon-bombs-global-energy-crisis-fossil-fuel Last accessed 1 March 2023.
← 28. www.oecd.org/coronavirus/en/data-insights/will-fossil-fuel-subsidies-surge-in-2021?utm_term=PAC&utm_medium=social&utm_source=facebook&utm_content= Last accessed 1 March 2023.
← 29. www.iea.org/topics/energy-subsidies Last accessed 1 March 2023.
← 30. www.ips-journal.eu/topics/democracy-and-society/kazakhstan-5638/ Last accessed 1 March 2023.
← 31. https://en.unesco.org/news/publications-policy-china-launches-worlds-largest-carbon-market#:~:text=From%20publications%20to%20policy%3A%20China%20launches%20world's%20largest%20carbon%20market,- 13%2F09%2F2021&text=Released%20in%20June%202021%2C%20the,largest%20national%20emissions%20trading%20scheme. Last accessed 1 March 2023.
← 32. https://carbonpricingdashboard.worldbank.org/ Last accessed 1 March 2023.
← 33. www.spglobal.com/commodityinsights/en/market-insights/latest-news/coal/012721-global-carbon-market-grows-20-to-272-billion-in-2020-refinitiv Last accessed 1 March 2023.
← 34. www.greenpeace.org/international/story/50689/carbon-offsets-net-zero-greenwashing-scam/ Last accessed 1 March 2023.
← 36. www.biopreferred.gov/BioPreferred/ Last accessed 1 March 2023.
← 37. www.astm.org/ Last accessed 1 March 2023.
← 38. www.weforum.org/agenda/2021/03/industrial-symbiosis-clusters/ Last accessed 1 March 2023.