This section highlights the typical initiating events that could lead to a leak during hydrogen production via electrolysis (such as mechanical failure or over pressurisation). It also proposes recommendations on key safety elements for a water electrolysis site.
Risk-based Regulatory Design for the Safe Use of Hydrogen
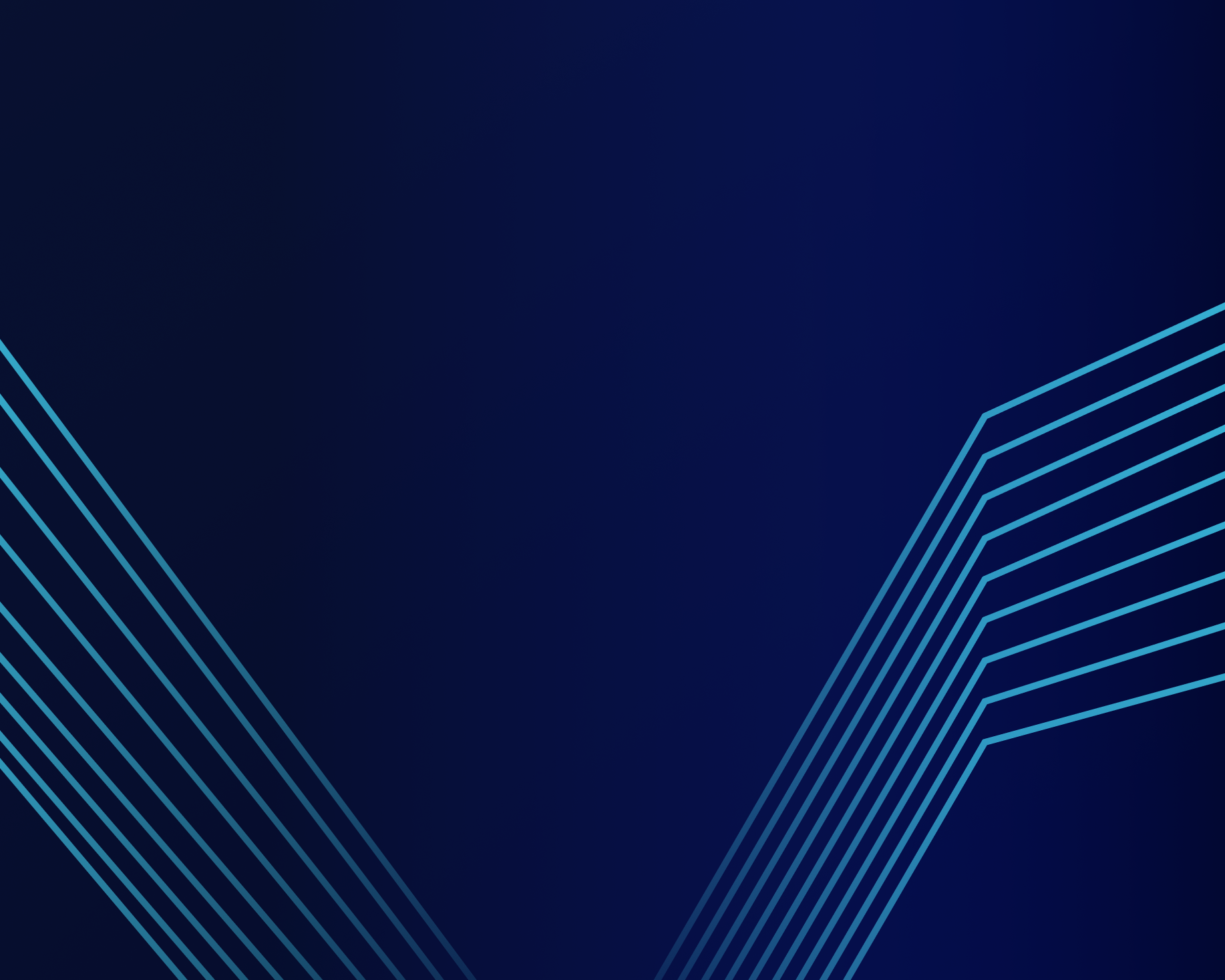
17. Production: Leakage in water electrolysis installations
Abstract
Hydrogen production by water electrolysis
Hydrogen production by water electrolysis is a technology that has been used for many years. Among the different types of electrolysers, an alkaline electrolyser is the more mature technology, with most large-scale plants (up to 165 MW) built in response to hydrogen demand for the production of ammonia (Krishnan et al., 2020[1]). Other technologies, including a proton exchange membrane (PEM) electrolyser, anion exchange membrane (AEM) electrolyser, and a solid oxide electrolyser cell (SOEC) are gaining market traction as they expect to be either more flexible or efficient and are more compact (IRENA, 2018[2]).
An electrolyser system comprises of an electrical power source, an electrolyser to split water molecules into H2 and O2 gases, gas collection and compression by a series of compressors and then either direct transmission for use in situ via pipework or storage within pressure cylinders for subsequent use either in situ or at another location.
Pressurised electrolysers are usually preferred due to the lower costs gained by avoiding or reducing the stages of mechanical compression of the generated hydrogen gas. Typically, hydrogen is delivered at 30 bar pressure. However, the pressure in storage systems and compressor are significantly higher (up to 1 000 bar).1 Compressors and storage systems are the major source of a potential leak in hydrogen production plants.
Global decarbonisation goals and the increasing investments in green hydrogen production require large-scale water electrolysis systems, where limited operating experience exists. In principle, safety aspects for large-scale electrolysers are the same as in small-scale electrolysers. However, some risk components, e.g. failure modes and their frequency of occurrence, could differ in large scale plants and further research is needed to provide the relevant data.
Existing technical norms
ISO 22734:2019 has been developed to cover construction, safety and performance requirements for hydrogen gas generation appliances i.e. electrolysing water to produce hydrogen. The standard applies to electrolysers for industrial and commercial use, indoor and outdoor residential use in sheltered areas, such as garages, utility rooms and similar residential locations.
OSHA Standard 1910.103 of Occupational Safety and Health Administration (OSHA) (United States Department of Labor, 2023[3]) in the United States uses the maximum hydrogen storage inventory to determine the location of a hydrogen storage vessel. This has a control hierarchy ranging from an outdoor location to a separate building,2 a special room3 and inside buildings not in a special room and exposed to other occupancies. The OSHA standard provides the minimum distances of several specified outdoor exposures (e.g. buildings, oxygen storage, open flames, concentration of people in congested areas, such as offices, etc.) from the hydrogen storage system based on the volume (three groups of volume range are determined), as well as the fire-resistance rating of structures, operating and maintenance instructions. Based on this standard when there are no fire walls between the system and the exposure the maximum separation distance among all kinds of exposures and all groups of system’s volume specified by the standard does not exceed 15.24 m.
Key safety / failure elements
The causes of hydrogen accidents in production site are similar to those of accidents which occur in the conventional hydrocarbon-based industry sectors.4 Typical initiating events that could lead to a hydrogen leak are:
mechanical failure of the components of the system, e.g. compressor, and of pipework that connects them
over pressurisation in one or more components of the system,
corrosion,
damage due to impact, and
human error (accidental opening of valves etc).
In general, the risk of harm associated with electrolysers is small compared to the risk of harm associated with compressors and pressurised storage.5 Risk calculations (Matthijsen and Kooi, 2006[4]) estimated the individual fatality risk 10-6 per year contour to be 4.5 m for pipework in HRS with onsite hydrogen production via electrolysis.
Hydrogen incidents (involving fire/explosion and injuries) relating to pipework failure are reduced over the last decades because of modern valve design and implementation of safety regulations.6 Based on historical data7 for compressors, cylinders, hoses, joints, pipes and valves the connecting pipe leaking frequency falls within the acceptable range8 set by the Purple book (Dutch Ministry of Transport and Water Management, 2005[5]) which are between 5∙10-7 - 5∙10-6 m-1∙y-1 for small leak (10 % of pipe diameter) dependent on the pipe nominal diameter and 1∙10-7 - 1∙10-6 m-1∙y-1 for rupture, suggesting a low risk of hydrogen leakage from the pipework connecting to the electrolyser.
In case of a hydrogen leak, the degree of confinement is a key parameter in the likely occurrence of an explosion. Deflagration of a free hydrogen-air gas cloud would lead to a maximum overpressure in the order of 10 kPa (Hysafe, 2023[6]) which is not considered a serious threat to life (7 kPa is the threshold to result people falling to the ground), while higher degrees of congestion can lead to increased probability of explosion. Another key factor in hydrogen safety is the presence or not of mechanical ventilation inside confined spaces that can affect the hydrogen distribution, and consequently, the maximum concentration levels that are achieved and the residence time of the flammable mixture inside the confinement.
Recommendations on key safety elements for a water electrolysis site for hydrogen production
Site layout
The inventory of the on-site hydrogen storage should be limited to the smallest practical amount required to meet operational demands.
The electrolyser should be located outdoors. If this is not possible, then any building or room in which an electrolyser is situated should be adequately ventilated to quickly disperse any hydrogen concentrations.
Hydrogen storage tubes should be situated outdoors.
Compressors should be located outdoors, or where this is not possible, indoors within a well-ventilated room. Compressors should be protected from impact by being located behind barriers or within a cage.
Safety distances between the different components of the production site should be implemented. In siting and layout design a safety distance of 6 m between all components and the compressors, which are considered the major risk contributors along with storage system, whereas a minimum of a 2 m distance9 between electrolysers should be considered. Electrolyser size is also a factor and should be considered while adjusting minimum distance. This is because the size of the electrolyser determines the hydrogen production rate.
Protective walls can reduce the safety distances, because they can act as a physical barriers protecting from the expansion of a potential explosion, provided that their endurable pressure is higher than the explosion pressure. The location of the protective walls relative to the facility should be carefully designed as in case of ignition protective walls can lead to increased overpressures in the area that they enclose. Furthermore, the reflected shock waves may cause secondary damage in front of the wall.
Standards / materials
The installation of hydrogen generation systems should meet the requirements of relevant standards, like ISO 22734:2019 (construction, safety, and performance requirements for hydrogen gas generation appliances). Moreover, standards like the OSHA Standard 1910.103 can be considered as safety reference for separation distances between the storage system and certain types of exposures.
Protective walls, if installed, should be constructed of non-combustible materials.
All equipment which is located within a potential flammable zone should comply with the ATEX Directive (European Commission, 2014[7]).
Non-combustible materials should be used in compartments or locations containing hydrogen storage vessels or hydrogen pipelines.
Safety devices
Pressure relief valves (PRV) should be fitted to all components that operate at high pressure. Relief valves should direct any vented hydrogen upward.
Flammable gas detectors and alarm systems should be provided. Alarms should be activated before a flammable gas concentration reaches 2 vol% (half LFL of 4 vol%), while automatic safety shutdown devices are recommended to shut of the hydrogen supply at 3 vol% concentration levels. The International Electrotechnical Commission standard, IEC 60079-29-1, specifies general requirements for construction, testing and performance, and describes the test methods that apply to portable, transportable and fixed equipment for the detection and measurement of flammable gas or vapour concentrations with air. ISO 26142:2010 – Hydrogen detection apparatus — Stationary applications10 provides the performance requirements of hydrogen detection apparatus in stationary installations (ISO, 2023[8]). The provision in this International Standard covers the hydrogen detection apparatus used to achieve the single and/or multifaceted safety operations, such as nitrogen purging or ventilation combined with supply system shut-off according to a hydrogen leak concentration. Hydrogen detection apparatus certified under this Standard ensure functional performance requirements, such as reliability, response time, stability, measuring range, selectivity and contamination.
Automated shutdown systems and local emergency stop buttons should be fitted in the electrolyser, compressor and storage areas.
Practices
The production site should be kept clean, free of combustible materials and potential ignition sources and without obstructions.
Proper and clear marking of the area with visible warning signs in the electrolyser room, the compressor site and in the storage facility to minimise the risk of ignition.
The number of flanged joints to pipework should be minimised, as flanged joints pose a greater risk of hydrogen leakage. Welded connections for joining pipework are preferred as they can reduce the generation of flammable atmospheres from small scale leakages.
Emergency arrangements should include specification of site evacuation arrangements and the provision of cooling to compressors and storage tanks in the event of a fire. During an incident an exclusion zone of at least 50 m to keep the public away from an accident scene should be provided.
Controls
Regular visual inspections (at least weekly) and risk-based maintenance of the electrolyser, the compressor and the equipment components, including the pipework, is crucial. Incorrect operation of a water electrolyser can lead to oxygen ingression in the hydrogen stream, which may exceed the explosion threshold limit. Using two staff (two pairs of eyes) for maintenance activities can reduce the risk of human error.
References
[5] Dutch Ministry of Transport and Water Management (2005), Guidelines for Quantitative Risk Assessment.
[7] European Commission (2014), Equipment for explosive atmospheres (ATEX), https://single-market-economy.ec.europa.eu/single-market/european-standards/harmonised-standards/equipment-explosive-atmospheres-atex_en.
[6] Hysafe (2023), Hysafe - Safety of Hydrogen as an Energy Carrier, http://www.hysafe.org/.
[2] IRENA (2018), Hydrogen from renewable power: Technology outlook for the energy transition, International Renewable Energy Agency.
[8] ISO (2023), ISO Standards, https://www.iso.org/standard/52319.html.
[1] Krishnan, S. et al. (2020), “Power to gas (H2): alkaline electrolysis. In Technological learning in the transition to a low-carbon energy system”, Academic Press, pp. 165-187.
[4] Matthijsen, A. and E. Kooi (2006), “Safety distances for hydrogen filling stations”, Journal of Loss Prevention in the Process Industries, Vol. 19/6, pp. 719-723.
[3] United States Department of Labor (2023), OSHA Laws & Regulations, https://www.osha.gov/laws-regs/regulations/standardnumber/1910/1910.103.
Notes
← 1. In low pressure releases (at 30 bar) through small, free jets leaks (d<1 mm) the flammable mixture extends less than 2.1 m from the leak point. In higher pressure releases (at 350 bar) and small leaks (d<1 mm) the extent flammable mixture extends to 6.3 m, leading also to longer flame length in case of jet fires. However, the distance of concentrations of nearly-stoichiometric mixtures, which are the most hazardous due to the fast burning velocities, is limited to less than 1 m, even for 350 bar operational pressures. Flash fires caused by a rupture in a stationary high pressure hydrogen storage vessel can lead to long harm distances (harm criteria 1% fatality) in order of “tens” m.
← 2. Made of light non-combustible construction on a substantial frame. Walls and roofs shall be lightly fastened and designed to relieve at a maximum internal pressure of 25 pounds per square foot. Windows shall be of shatterproof glass or plastic in metal frames. Doors shall be located in such a manner that they will be readily accessible to personnel in an emergency. Adequate ventilation to the outdoors. No sources of ignition. More details in https://www.osha.gov/laws-regs/regulations/standardnumber/1910/1910.103.
← 3. Floors, walls, and ceilings shall have a fire resistance rating of at least 2 hours. Walls or partitions shall be continuous from floor to ceiling and shall be securely anchored. At least one wall shall be an exterior wall. Openings to other parts of the building shall not be permitted. Windows and doors shall be in exterior walls and doors shall be located in such a manner that they will be accessible in an emergency. Windows shall be of shatterproof glass or plastic in metal frames. Adequate ventilation. No sources of ignition. Explosion venting shall be provided in exterior walls or roof only. More details in https://www.osha.gov/laws-regs/regulations/standardnumber/1910/1910.103.
← 4. Analysis, from three incident databases (ENSAD ,HIAD 2.0 and H2tools ) showed that the causes of hydrogen accidents are similar to those of accidents which occur in the conventional hydrocarbon-based industry sectors. ENSAD reported no hydrogen releases at production sites.
← 5. Based on the HIAD2.0 and h2tools incident databases.
← 6. H2tools database reported no accidents (involving fire/explosion and injuries) relating to pipework failure after 1990 - when modern valve design and safety regulations were implemented.
← 7. Data coming from offshore oil industry.
← 8. According to calculations performed by Sandia National Laboratory in the United States.
← 9. As per Chinese standard, design code for hydrogen station GB50177-2005.
← 10. See Directive 2014/34/EU on Equipment for explosive atmospheres (ATEX) (European Commission, 2014[7]).